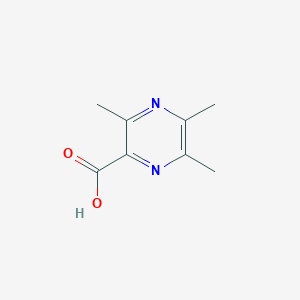
3,5,6-trimethylpyrazine-2-carboxylic Acid
Overview
Description
3,5,6-Trimethylpyrazine-2-carboxylic acid (TMP-COOH) is a methyl-substituted pyrazine derivative with a carboxylic acid functional group at the 2-position. It is synthesized via the oxidation of ligustrazine (tetramethylpyrazine, TMP) using potassium permanganate (KMnO₄) under controlled conditions (55°C, 24 hours), yielding a light-yellow solid with a melting point of 162–163°C . TMP-COOH serves as a critical intermediate in medicinal chemistry, particularly in the design of ligustrazine derivatives for anticancer, neuroprotective, and metabolic studies . Notably, it is a metabolite of tetramethylpyrazine in rabbits, formed via oxidation of a methyl group to a carboxylic acid .
Preparation Methods
Oxidation of Trimethylpyrazine Using Potassium Permanganate
The most widely documented method for synthesizing 3,5,6-trimethylpyrazine-2-carboxylic acid involves the oxidation of trimethylpyrazine (TMP) with potassium permanganate (KMnO₄). This approach leverages the strong oxidizing properties of KMnO₄ to convert a methyl group on the pyrazine ring into a carboxylic acid functionality.
Reaction Protocol
In a representative procedure, trimethylpyrazine (5.0 g, 36.8 mmol) is dissolved in water (200 mL), and an aqueous solution of KMnO₄ (8.6 g in 150 mL water) is added dropwise over 60 minutes at room temperature . The mixture is then stirred at 50°C for 12 hours. Post-reaction, the warm solution is filtered to remove manganese dioxide byproducts, and the filtrate is acidified to pH 2.0 using concentrated hydrochloric acid. The product is extracted with ethyl acetate, dried over anhydrous sodium sulfate, and recrystallized from acetone, yielding a light yellow solid (2.39 g, 47.8%) .
Key Parameters:
-
Oxidizing Agent : KMnO₄ (stoichiometric excess)
-
Temperature : 50°C
-
Reaction Time : 12 hours
-
Workup : Acidification, extraction, recrystallization
Alternative Oxidation Strategies
Hydrogen Peroxide-Mediated Oxidation
Although less common, hydrogen peroxide (H₂O₂) has been explored as an oxidizing agent in the presence of acetic acid (AcOH). In one protocol, trimethylpyrazine (21.8 g, 160 mmol) is treated with H₂O₂ (30%, 36 mL) in AcOH at 90°C for 4 hours under nitrogen . After neutralization with sodium hydroxide, the intermediate is extracted with dichloromethane (DCM) and further processed with acetic anhydride. While this method primarily yields chloromethyl derivatives, it highlights the versatility of H₂O₂ in functionalizing the pyrazine ring .
Synthesis via Intermediate Derivatives
Ligustrazinyloxy-Aromatic Acid Derivatives
A multi-step synthesis route involves the preparation of ligustrazinyloxy-aromatic acid derivatives as intermediates. For instance, bromo-TMP is coupled with hydroxybenzoic acids under alkaline conditions to form ester or ether bonds, followed by hydrolysis with 20% potassium hydroxide (KOH) at 60°C . While this method focuses on generating analogs, it underscores the reactivity of the pyrazine core in nucleophilic substitution reactions .
Critical Analysis of Methodologies
Yield Comparisons
Method | Oxidizing Agent | Temperature | Time | Yield | Source |
---|---|---|---|---|---|
KMnO₄ Oxidation | KMnO₄ | 50°C | 12 h | 47.8% | |
Hot KMnO₄ Oxidation | KMnO₄ | >50°C | N/A | N/A | |
H₂O₂/AcOH Oxidation | H₂O₂ | 90°C | 4 h | N/A |
The KMnO₄-mediated oxidation remains the most reliable method, balancing moderate yields with operational simplicity. The absence of yield data for alternative methods underscores the need for further optimization.
Challenges and Considerations
-
Byproduct Formation : Manganese dioxide precipitates necessitate filtration, adding complexity to the workup .
-
pH Sensitivity : Precise acidification (pH 2.0) is critical to maximize product recovery during extraction .
-
Solvent Selection : Ethyl acetate outperforms DCM in extracting the polar carboxylic acid product .
Chemical Reactions Analysis
Types of Reactions
Finafloxacin hydrochloride undergoes various chemical reactions, including:
Reduction: Involves the addition of hydrogen or the removal of oxygen.
Substitution: Involves the replacement of one atom or group with another.
Common Reagents and Conditions
Common reagents used in these reactions include sulfuric acid, N-bromosuccinimide, and other strong acids and bases. The reactions are typically carried out under controlled temperatures and pressures to ensure optimal yields and purity .
Major Products Formed
The major products formed from these reactions include various intermediates that are further processed to yield finafloxacin hydrochloride. These intermediates are crucial for the final synthesis and contribute to the compound’s efficacy and stability .
Scientific Research Applications
Biological Activities
Anticancer Properties
TMPCA has shown promise as an anticancer agent. Studies indicate that it inhibits the growth of tumor cells by damaging DNA and interfering with the cell cycle. This activity suggests potential applications in cancer therapeutics, particularly in targeting specific types of cancer cells .
Anti-platelet Activity
Research has demonstrated that TMPCA possesses anti-platelet properties. In vitro studies using platelet-rich plasma from rabbit blood revealed that TMPCA significantly inhibited platelet aggregation, which could have implications for cardiovascular disease treatments . The effective concentration (EC50) values for TMPCA's protective effects on damaged endothelial cells were also evaluated, showing promising results in cellular protection .
Applications in Flavor Chemistry
TMPCA is recognized for its flavor profile, particularly in food chemistry. It is a key compound in the volatile fraction of roasted coffee and contributes to the characteristic aroma of various food products. Its metabolites have been studied to understand their roles in flavor development during food processing .
Case Study 1: Anticancer Research
A study investigated the effects of TMPCA on breast cancer cell lines. The results indicated a dose-dependent inhibition of cell proliferation, with significant apoptotic effects observed at higher concentrations. This study highlights TMPCA's potential as a lead compound for developing new anticancer drugs.
Case Study 2: Flavor Profile Analysis
In a flavor chemistry study, researchers analyzed the volatile compounds released during the roasting of coffee beans. TMPCA was identified as a significant contributor to the aroma profile, enhancing the sensory attributes of coffee. This finding underscores its importance in food science and product development.
Summary of Findings
Mechanism of Action
Finafloxacin hydrochloride exerts its effects by selectively inhibiting bacterial type II topoisomerase enzymes, including DNA gyrase and DNA topoisomerase IV . These enzymes are essential for bacterial DNA replication, transcription, repair, and recombination. By inhibiting these enzymes, finafloxacin hydrochloride disrupts bacterial DNA processes, leading to cell death .
Comparison with Similar Compounds
Comparison with Structurally Similar Compounds
Structural and Functional Group Variations
2-Hydroxymethyl-3,5,6-trimethylpyrazine (TMP-OH)
- Structure : Differs by a hydroxymethyl (-CH₂OH) group at the 2-position instead of a carboxylic acid.
- Synthesis : Produced via oxidation of TMP, preceding TMP-COOH formation .
- Applications : Used in polyethylene glycol (PEG) conjugates to enhance solubility .
- Key Difference : The hydroxymethyl group reduces acidity compared to TMP-COOH, impacting pharmacokinetics and metabolic stability .
5,6-Dimethylpyrazine-2,3-dicarboxylic Acid
- Structure : Contains two carboxylic acid groups at the 2- and 3-positions and methyl groups at the 5- and 6-positions.
- Synthesis : Prepared via crystallographic methods, distinct from TMP-COOH’s oxidation route .
3-Amino-2-pyrazinecarboxylic Acid
- Structure: Features an amino (-NH₂) group at the 3-position and a carboxylic acid at the 2-position.
- Synthesis : Derived from pyrazine precursors through amination and oxidation .
- Applications: Exhibits antimicrobial activity due to the amino group’s hydrogen-bonding capability .
- Key Difference: The amino group introduces basicity, contrasting with TMP-COOH’s acidic profile .
1,2,4-Triazolo[4,3-a]pyrazine-3-carboxylic Acid
- Structure : A fused triazolo-pyrazine ring system with a carboxylic acid.
- Synthesis : Constructed via cyclization reactions, differing from TMP-COOH’s straightforward oxidation .
- Key Difference : The triazolo ring enhances planar rigidity, influencing binding to biological targets like enzymes .
Anticancer Activity
- TMP-COOH: Used in ligustrazine-triterpenoid conjugates (e.g., compound 11f) to enhance cytotoxicity and selectivity in cancer cells (e.g., HepG2, MCF-7) .
- Comparison with TMP-OH : TMP-COOH derivatives show superior metabolic stability in plasma compared to hydroxymethyl analogs, critical for drug candidate screening .
Neuroprotective Effects
- TMP-COOH : Conjugated with hydroxybenzoic acids (e.g., compounds 1d–5d ) to protect against CoCl₂-induced neurotoxicity in PC12 cells .
Physicochemical Properties
Metabolic Stability
Biological Activity
3,5,6-Trimethylpyrazine-2-carboxylic acid (TMPCA) has garnered attention in recent years due to its diverse biological activities. This compound is a derivative of trimethylpyrazine, which is known for its presence in various natural sources, including coffee and certain medicinal plants. The following sections provide a comprehensive overview of TMPCA's synthesis, biological activities, and relevant research findings.
Synthesis of this compound
TMPCA can be synthesized through various chemical pathways. One notable method involves the reaction of 3,5,6-trimethylpyrazine with carbonyl diimidazole in tetrahydrofuran (THF), followed by further reactions with trifluoroacetic acid to yield the desired carboxylic acid derivative .
Neuroprotective Effects
Recent studies have highlighted the neuroprotective properties of TMPCA. In a study evaluating the protective effect against cobalt chloride-induced neurotoxicity in PC12 cells, TMPCA demonstrated significant protective effects with an effective concentration (EC50) of 64.459 µM . Additionally, a derivative of TMPCA showed even greater efficacy with an EC50 of 4.249 µM, indicating that modifications to the structure can enhance its neuroprotective capabilities .
Antitumor Activity
TMPCA has also been investigated for its potential antitumor activity. A series of studies have shown that ligustrazine-based compounds, which include TMPCA as a moiety, exhibit inhibitory effects on histone deacetylases (HDACs), particularly HDAC1 and HDAC2. These compounds were evaluated for their antiproliferative effects against cancer cell lines such as HT-29 (colorectal cancer) and SH-SY5Y (neuroblastoma). The results indicated that these compounds could inhibit tumor cell growth effectively .
Table: Summary of Biological Activities of TMPCA
The biological activity of TMPCA may be attributed to its ability to modulate various cellular pathways. For instance, its role as an HDAC inhibitor suggests that it can influence gene expression related to cell cycle regulation and apoptosis in cancer cells. Moreover, its neuroprotective effects might be linked to its ability to reduce oxidative stress and inflammation in neuronal cells.
Q & A
Q. What are the optimized synthetic routes for 3,5,6-trimethylpyrazine-2-carboxylic acid, and how do reaction conditions influence yield?
Basic Research Question
The compound is synthesized via oxidation of 2-(chloromethyl)-3,5,6-trimethylpyrazine using KMnO₄ under controlled conditions. Key parameters include:
- Temperature : 50–55°C (optimal for oxidation without side reactions).
- Reaction Time : 12–24 hours (prolonged stirring ensures complete conversion).
- Purification : Recrystallization from acetone yields a light-yellow solid with 48% efficiency .
Methodological Insight : Lower yields (e.g., 48%) may result from incomplete oxidation or losses during filtration. Alternatives like optimizing KMnO₄ stoichiometry or using phase-transfer catalysts could improve efficiency.
Q. How is this compound utilized as an intermediate in medicinal chemistry?
Advanced Research Question
The carboxylic acid group enables derivatization via coupling reagents (e.g., 1,1-carbonyldiimidazole, CDI) to form amides or esters. For example:
- Derivative Synthesis : Reacting with CDI in THF generates activated intermediates for subsequent nucleophilic substitution, yielding compounds with neuroprotective or antimicrobial properties .
Data Table : Common Derivatives and Applications
Derivative Type | Target Activity | Reference |
---|---|---|
Ligustrazinyloxybenzoic esters | Neuroprotection (CoCl₂-induced toxicity) | |
Pyrazine-carboxamides | Antimicrobial agents |
Q. What methodologies assess the metabolic stability of this compound derivatives?
Advanced Research Question
In vitro plasma stability assays are critical for early-stage drug screening:
- Protocol : Incubate derivatives in rat plasma at 37°C, followed by HPLC or LC-MS analysis to quantify degradation over time .
- Key Finding : Derivatives with ester linkages (e.g., ligustrazinyloxybenzoic esters) show higher hydrolysis rates compared to amides, impacting bioavailability .
Q. How can structural modifications enhance the biological activity of this compound?
Advanced Research Question
Modifications at the carboxyl group or pyrazine ring alter pharmacokinetics and target binding:
- Carboxyl Group : Conversion to amides improves metabolic stability; e.g., trifluoroacetamide derivatives enhance lipophilicity .
- Pyrazine Ring : Methyl groups at positions 3,5,6 increase steric hindrance, reducing enzymatic degradation .
Structural-Activity Relationship (SAR) : - Hydrogen Bonding : The carboxylic acid participates in intermolecular H-bonding, influencing crystal packing and solubility .
Q. What analytical techniques are recommended for characterizing this compound and its derivatives?
Basic Research Question
- Melting Point : 162–163°C (recrystallized from acetone) .
- Spectroscopy : ¹H/¹³C NMR confirms substitution patterns; IR identifies carboxyl C=O stretches (~1700 cm⁻¹).
- Chromatography : HPLC purity assessment using C18 columns with UV detection at 254 nm .
Q. How do conflicting bioactivity results arise among derivatives, and how can they be resolved?
Advanced Research Question
Discrepancies may stem from:
- Assay Conditions : Varying cell lines (e.g., PC12 vs. bacterial models) or concentrations .
- Structural Isomerism : Undetected regioisomers during synthesis (e.g., CDI-mediated coupling) .
Resolution Strategy : - Dose-Response Studies : Establish EC₅₀/IC₅₀ values across multiple assays.
- X-ray Crystallography : Verify regiochemistry of active derivatives .
Q. What mechanistic insights exist for the biological activity of this compound derivatives?
Advanced Research Question
- Neuroprotection : Derivatives reduce oxidative stress in PC12 cells by upregulating Nrf2/ARE pathways .
- Antimicrobial Action : Pyrazine-carboxamides disrupt bacterial membrane integrity via hydrophobic interactions .
Experimental Design : Use knockout cell lines or enzyme inhibition assays (e.g., COX-2 for anti-inflammatory activity) to validate targets .
Properties
IUPAC Name |
3,5,6-trimethylpyrazine-2-carboxylic acid | |
---|---|---|
Source | PubChem | |
URL | https://pubchem.ncbi.nlm.nih.gov | |
Description | Data deposited in or computed by PubChem | |
InChI |
InChI=1S/C8H10N2O2/c1-4-5(2)10-7(8(11)12)6(3)9-4/h1-3H3,(H,11,12) | |
Source | PubChem | |
URL | https://pubchem.ncbi.nlm.nih.gov | |
Description | Data deposited in or computed by PubChem | |
InChI Key |
WVHVVMMOAVPNKV-UHFFFAOYSA-N | |
Source | PubChem | |
URL | https://pubchem.ncbi.nlm.nih.gov | |
Description | Data deposited in or computed by PubChem | |
Canonical SMILES |
CC1=C(N=C(C(=N1)C)C(=O)O)C | |
Source | PubChem | |
URL | https://pubchem.ncbi.nlm.nih.gov | |
Description | Data deposited in or computed by PubChem | |
Molecular Formula |
C8H10N2O2 | |
Source | PubChem | |
URL | https://pubchem.ncbi.nlm.nih.gov | |
Description | Data deposited in or computed by PubChem | |
DSSTOX Substance ID |
DTXSID50649160 | |
Record name | 3,5,6-Trimethylpyrazine-2-carboxylic acid | |
Source | EPA DSSTox | |
URL | https://comptox.epa.gov/dashboard/DTXSID50649160 | |
Description | DSSTox provides a high quality public chemistry resource for supporting improved predictive toxicology. | |
Molecular Weight |
166.18 g/mol | |
Source | PubChem | |
URL | https://pubchem.ncbi.nlm.nih.gov | |
Description | Data deposited in or computed by PubChem | |
CAS No. |
186534-01-0 | |
Record name | 3,5,6-Trimethylpyrazine-2-carboxylic acid | |
Source | EPA DSSTox | |
URL | https://comptox.epa.gov/dashboard/DTXSID50649160 | |
Description | DSSTox provides a high quality public chemistry resource for supporting improved predictive toxicology. | |
Retrosynthesis Analysis
AI-Powered Synthesis Planning: Our tool employs the Template_relevance Pistachio, Template_relevance Bkms_metabolic, Template_relevance Pistachio_ringbreaker, Template_relevance Reaxys, Template_relevance Reaxys_biocatalysis model, leveraging a vast database of chemical reactions to predict feasible synthetic routes.
One-Step Synthesis Focus: Specifically designed for one-step synthesis, it provides concise and direct routes for your target compounds, streamlining the synthesis process.
Accurate Predictions: Utilizing the extensive PISTACHIO, BKMS_METABOLIC, PISTACHIO_RINGBREAKER, REAXYS, REAXYS_BIOCATALYSIS database, our tool offers high-accuracy predictions, reflecting the latest in chemical research and data.
Strategy Settings
Precursor scoring | Relevance Heuristic |
---|---|
Min. plausibility | 0.01 |
Model | Template_relevance |
Template Set | Pistachio/Bkms_metabolic/Pistachio_ringbreaker/Reaxys/Reaxys_biocatalysis |
Top-N result to add to graph | 6 |
Feasible Synthetic Routes
Disclaimer and Information on In-Vitro Research Products
Please be aware that all articles and product information presented on BenchChem are intended solely for informational purposes. The products available for purchase on BenchChem are specifically designed for in-vitro studies, which are conducted outside of living organisms. In-vitro studies, derived from the Latin term "in glass," involve experiments performed in controlled laboratory settings using cells or tissues. It is important to note that these products are not categorized as medicines or drugs, and they have not received approval from the FDA for the prevention, treatment, or cure of any medical condition, ailment, or disease. We must emphasize that any form of bodily introduction of these products into humans or animals is strictly prohibited by law. It is essential to adhere to these guidelines to ensure compliance with legal and ethical standards in research and experimentation.