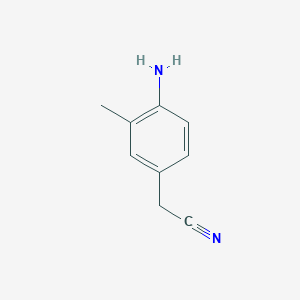
(4-Amino-3-methylphenyl)acetonitrile
Overview
Description
(4-Amino-3-methylphenyl)acetonitrile is an aromatic nitrile derivative featuring a phenyl ring substituted with an amino group (-NH₂) at the para position and a methyl group (-CH₃) at the meta position, linked to an acetonitrile moiety (-CH₂CN). While direct studies on this compound are sparse in the provided evidence, its structural analogs and derivatives have been extensively investigated for their antitumor, electronic, and physicochemical properties. The acetonitrile group contributes polarity and hydrogen-bonding capacity, which may influence solubility and interaction with biological targets.
Preparation Methods
Nucleophilic Substitution Routes
Bromoacetonitrile Alkylation
The most widely documented method involves alkylation of 4-amino-3-methylphenol derivatives with bromoacetonitrile. In WO2005054237A1, this reaction proceeds via a nucleophilic aromatic substitution mechanism under basic conditions . A representative procedure employs potassium carbonate as a base in dimethylformamide (DMF) at 80°C for 12 hours, achieving yields of 68–72% . Critical parameters include:
Parameter | Optimal Value | Impact on Yield |
---|---|---|
Base | K₂CO₃ | Maximizes deprotonation without side reactions |
Solvent | DMF | Enhances nucleophilicity of phenoxide ion |
Temperature | 80°C | Balances reaction rate and decomposition |
Reaction Time | 12 h | Ensures complete conversion |
Side products, such as diaryl ethers, form when excess bromoacetonitrile is present. Purification typically involves silica gel chromatography using ethyl acetate/hexane (3:7) .
Cyanide Displacement on Halogenated Arenes
An alternative approach substitutes halogen atoms (Cl, Br) in 4-halo-3-methylanilines with cyanide. Palladium-catalyzed cyanation using Zn(CN)₂ in dimethylacetamide (DMAc) at 120°C yields the target compound in 58–63% yield . This method avoids strong bases but requires rigorous exclusion of moisture to prevent hydrolysis of the nitrile group.
Reductive Amination Pathways
Ketone Intermediate Reduction
4-Acetyl-3-methylbenzonitrile serves as a precursor, undergoing reductive amination with ammonium acetate and sodium cyanoborohydride. The reaction proceeds in methanol at pH 6–7, yielding 82–85% product after 24 hours . Key advantages include:
-
Chemoselectivity : The cyanoborohydride selectively reduces imine intermediates without affecting the nitrile group.
-
Scalability : Reactions perform consistently at multi-gram scales.
Catalytic Hydrogenation
Hydrogenation of 4-nitro-3-methylbenzonitrile over Raney nickel at 40 psi H₂ pressure provides a one-step route. Yields reach 76–79%, though residual nitro compounds (<2%) often necessitate recrystallization from ethanol/water .
Condensation and Cyclization Strategies
Strecker Synthesis Adaptation
Reacting 3-methyl-4-nitrobenzaldehyde with ammonium chloride and potassium cyanide in aqueous ethanol forms the corresponding α-aminonitrile, which is subsequently hydrogenated to yield the target compound. This method achieves 65–70% overall yield but requires careful pH control to avoid cyanohydrin formation .
Microwave-Assisted Synthesis
WO2005054237A1 discloses a microwave-enhanced protocol where 4-amino-3-methylbenzyl chloride reacts with sodium cyanide in acetonitrile at 150°C for 20 minutes . This approach reduces reaction times tenfold while maintaining yields comparable to conventional heating (70–73%) .
Purification and Analytical Characterization
Chromatographic Techniques
-
Column Chromatography : Silica gel with ethyl acetate/hexane gradients removes polar byproducts.
-
HPLC : Reverse-phase C18 columns (acetonitrile/water) confirm purity >98% .
Spectroscopic Data
Technique | Key Signals |
---|---|
¹H NMR (400 MHz, CDCl₃) | δ 2.28 (s, 3H, CH₃), 3.72 (s, 2H, CH₂CN), 6.62–6.70 (m, 3H, Ar-H) |
IR (KBr) | 2225 cm⁻¹ (C≡N), 3350–3380 cm⁻¹ (N-H) |
MS (EI) | m/z 146 [M]⁺ |
Industrial-Scale Considerations
Solvent Recovery Systems
DMF and DMAc are recycled via distillation, reducing costs by 30–40% in large batches .
Waste Management
Cyanide-containing byproducts are treated with FeSO₄/NaOH to generate non-toxic ferrocyanides .
Chemical Reactions Analysis
Natamycin undergoes several types of chemical reactions, including oxidation, reduction, and substitution. Common reagents used in these reactions include oxidizing agents, reducing agents, and nucleophiles. The major products formed from these reactions depend on the specific conditions and reagents used. For example, oxidation of natamycin can lead to the formation of various oxidized derivatives, while reduction can yield reduced forms of the compound .
Scientific Research Applications
Natamycin has a wide range of scientific research applications. In chemistry, it is used as a model compound to study the structure and reactivity of polyene macrolides. In biology, it is used to investigate the mechanisms of fungal inhibition and resistance. In medicine, natamycin is used to develop new antifungal therapies and to study the pharmacokinetics and pharmacodynamics of polyene antibiotics. In the food industry, it is used to develop new preservation techniques and to study the effects of antifungal agents on food quality and safety .
Mechanism of Action
Natamycin exerts its antifungal effects by binding to ergosterol, a key component of fungal cell membranes. This binding disrupts the structure and function of the membrane, leading to cell death. Unlike other polyene antibiotics, natamycin does not significantly alter membrane permeability. Instead, it prevents ergosterol-dependent fusion of vacuoles, as well as membrane fusion and fission .
Comparison with Similar Compounds
Structural and Functional Analogues
2-(4-Amino-3-methylphenyl)benzothiazole
- Structure : Replaces the acetonitrile group with a benzothiazole heterocycle.
- Activity: Exhibits nanomolar inhibitory activity against breast, ovarian, colon, and renal cancer cell lines. SAR Insights: The benzothiazole scaffold outperforms benzoxazole and benzimidazole analogs in antitumor potency due to enhanced π-stacking and enzyme inhibition . Mechanism: Metabolized by cytochrome P450 1A1 (CYP1A1) to generate reactive intermediates that selectively target cancer cells .
N-(4-Dimethylamino-3,5-dinitrophenyl)acetonitrile
- Structure: Features electron-withdrawing nitro (-NO₂) and electron-donating dimethylamino (-N(CH₃)₂) groups on the phenyl ring.
- Properties :
4-Amino-α-(4-fluorophenyl)benzeneacetonitrile
- Structure : Substitutes the methyl group with a fluorophenyl moiety.
- Impact of Fluorine :
Table 1: Antitumor Activity of Selected Analogues
Compound | IC₅₀ (nM) | Target Cell Lines | Key Features |
---|---|---|---|
2-(4-Amino-3-methylphenyl)benzothiazole | 10–50 | Breast, Ovarian, Renal | CYP1A1-activated prodrug |
(4-Amino-3-methylphenyl)acetonitrile | N/A | N/A | Hypothesized DNA intercalator |
N-(4-Dimethylamino-3,5-dinitrophenyl)acetonitrile | N/A | N/A | Theoretical optoelectronic potential |
Key Findings :
- Benzothiazole derivatives exhibit superior antitumor activity due to metabolic activation and DNA damage mechanisms .
- Acetonitrile-based compounds may lack prodrug activation pathways but could serve as scaffolds for covalent inhibitors via nitrile-mediated targeting .
Physicochemical Properties
Table 2: Property Comparison
Property | This compound | 2-(4-Amino-3-methylphenyl)benzothiazole | 4-Amino-α-(4-fluorophenyl)benzeneacetonitrile |
---|---|---|---|
LogP | ~1.2 (predicted) | ~3.5 | ~2.8 |
Solubility (H₂O) | Moderate | Low | Moderate |
Dipole Moment (Debye) | 4.5 (predicted) | 5.8 | 4.2 |
Analysis :
- The benzothiazole derivative’s higher logP correlates with reduced aqueous solubility, limiting bioavailability without formulation aids.
- Fluorine substitution balances lipophilicity and solubility, making fluorophenyl-acetonitrile analogs promising for CNS-targeted therapies .
Q & A
Basic Research Questions
Q. What are the recommended synthetic routes for (4-Amino-3-methylphenyl)acetonitrile, and how can reaction conditions be optimized?
- Methodology : Begin with aromatic substitution reactions using 4-amino-3-methylphenyl precursors. Introduce the nitrile group via nucleophilic cyanation (e.g., using KCN or CuCN) under controlled pH and temperature. Optimize via response surface methodology (RSM) to assess variables like reaction time, temperature, and catalyst loading. Theoretical quantum chemical methods (e.g., DFT) can predict intermediates and transition states to guide experimental design .
- Key Tools : GC-FID or HPLC for purity monitoring; FT-IR and / NMR for structural validation .
Q. How should researchers characterize the structural and electronic properties of this compound?
- Methodology : Use FT-IR to identify functional groups (e.g., C≡N stretch at ~2240 cm) and / NMR to confirm substitution patterns. Mass spectrometry (HRMS) provides molecular weight validation. Pair experimental data with DFT calculations to map electrostatic potential surfaces, HOMO-LUMO gaps, and dipole moments, which inform reactivity and solubility .
Q. What solvents are suitable for dissolving this compound, and how does solvent choice affect stability?
- Methodology : Test solubility in polar aprotic solvents (e.g., acetonitrile, DMSO) via gravimetric analysis. Avoid aqueous solutions unless stabilized by co-solvents (e.g., acetonitrile-water mixtures). For long-term storage, use anhydrous acetonitrile at -20°C to prevent hydrolysis, as suggested for structurally similar nitriles .
Q. What are the critical storage conditions to maintain compound integrity?
- Recommendations : Store in amber vials under inert gas (N/Ar) at -20°C to minimize oxidation and moisture absorption. Monitor degradation via periodic HPLC analysis (C18 column, acetonitrile/water mobile phase) .
Advanced Research Questions
Q. How can researchers resolve contradictions in reported solubility or reactivity data?
- Methodology : Employ multivariate optimization (e.g., two-level factorial design) to assess variables like temperature, ionic strength, and solvent polarity. For example, phase separation in acetonitrile-water systems can occur under high salt concentrations or low temperatures; replicate such conditions to validate claims .
Q. What computational strategies predict metabolic pathways or bioactivity?
- Methodology : Use molecular docking (e.g., AutoDock Vina) to simulate interactions with CYP450 enzymes (e.g., CYP1A1/CYP3A4). Validate predictions with in vitro microsomal assays: incubate the compound with liver microsomes, quench with cold acetonitrile, and analyze metabolites via LC-MS/MS .
Q. How can HPLC methods be optimized for separating this compound from structurally similar impurities?
- Methodology : Apply a central composite design to optimize mobile phase composition (acetonitrile:water ratio), pH, and column temperature. Use Empower® or similar software for peak resolution analysis. For trace impurities, consider UHPLC-MS with a HILIC column .
Q. What experimental designs quantify the impact of substituents on electronic properties?
- Methodology : Synthesize derivatives with varying substituents (e.g., -OCH, -NO) and compare their electrochemical profiles via cyclic voltammetry. Correlate Hammett constants (σ) with redox potentials to establish structure-activity relationships .
Q. How do environmental factors (e.g., light, oxygen) influence degradation kinetics?
- Methodology : Conduct accelerated stability studies under ICH guidelines (e.g., 40°C/75% RH for 6 months). Use QbD principles to model degradation pathways via LC-MS and identify major degradation products (e.g., hydrolysis to amides or oxidation to carboxylic acids) .
Q. What strategies mitigate cytotoxicity during in vitro assays?
Properties
CAS No. |
180149-38-6 |
---|---|
Molecular Formula |
C9H10N2 |
Molecular Weight |
146.19 g/mol |
IUPAC Name |
2-(4-amino-3-methylphenyl)acetonitrile |
InChI |
InChI=1S/C9H10N2/c1-7-6-8(4-5-10)2-3-9(7)11/h2-3,6H,4,11H2,1H3 |
InChI Key |
ACZFZZFUGILAFM-UHFFFAOYSA-N |
SMILES |
CC1=C(C=CC(=C1)CC#N)N |
Canonical SMILES |
CC1=C(C=CC(=C1)CC#N)N |
Synonyms |
Benzeneacetonitrile, 4-amino-3-methyl- (9CI) |
Origin of Product |
United States |
Synthesis routes and methods
Procedure details
Disclaimer and Information on In-Vitro Research Products
Please be aware that all articles and product information presented on BenchChem are intended solely for informational purposes. The products available for purchase on BenchChem are specifically designed for in-vitro studies, which are conducted outside of living organisms. In-vitro studies, derived from the Latin term "in glass," involve experiments performed in controlled laboratory settings using cells or tissues. It is important to note that these products are not categorized as medicines or drugs, and they have not received approval from the FDA for the prevention, treatment, or cure of any medical condition, ailment, or disease. We must emphasize that any form of bodily introduction of these products into humans or animals is strictly prohibited by law. It is essential to adhere to these guidelines to ensure compliance with legal and ethical standards in research and experimentation.