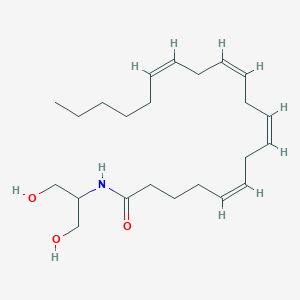
N-arachidonoyl dihydroxypropylamine
Overview
Description
N-arachidonoyl dihydroxypropylamine is a lipid-derived compound characterized by an arachidonic acid backbone conjugated to a dihydroxypropylamine moiety. Its chemical formula is C₂₃H₃₉NO₃, with a molecular weight of 377.29 g/mol . Structurally, it belongs to the N-acyl amide family, which includes endogenous cannabinoids like anandamide (AEA) and 2-arachidonoylglycerol (2-AG) .
Preparation Methods
Synthetic Routes for N-Arachidonoyl Dihydroxypropylamine
Acylation of Dihydroxypropylamine with Arachidonoyl Chloride
The most widely documented method involves the acylation of dihydroxypropylamine using arachidonoyl chloride. This reaction typically proceeds in anhydrous dichloromethane (DCM) or tetrahydrofuran (THF) under inert atmosphere (N₂ or Ar). Triethylamine (TEA) is employed as a base to neutralize HCl generated during the reaction.
Reaction Conditions:
-
Molar Ratio: 1:1.2 (dihydroxypropylamine : arachidonoyl chloride)
-
Temperature: 0–4°C (initial), progressing to room temperature over 4–6 hours
-
Catalyst: None required; base (TEA) serves as an acid scavenger
Yield: 65–72% after purification via silica gel chromatography.
Enzymatic Synthesis Using Lipase Catalysts
Recent advances explore lipase-mediated synthesis to enhance stereoselectivity and reduce byproducts. Candida antarctica lipase B (CAL-B) immobilized on acrylic resin demonstrates efficacy in catalyzing the amidation between arachidonic acid and dihydroxypropylamine .
Optimized Parameters:
-
Solvent: Tert-butyl alcohol (minimizes substrate denaturation)
-
Water Activity (a₄): 0.25 (controlled via salt hydrates)
-
Temperature: 37°C
-
Reaction Time: 48 hours
Yield: 58–63%, with >95% enantiomeric excess (ee) .
Critical Analysis of Methodologies
Chemical vs. Enzymatic Routes
A comparative evaluation reveals trade-offs between efficiency and sustainability:
Parameter | Chemical Acylation | Enzymatic Synthesis |
---|---|---|
Reaction Time | 4–6 hours | 48 hours |
Yield (%) | 65–72 | 58–63 |
Byproducts | HCl, unreacted chloride | Minimal |
Environmental Impact | High (organic solvents) | Low (aqueous-friendly) |
Enzymatic methods, while slower, align with green chemistry principles by avoiding harsh reagents .
Purification and Characterization
Post-synthesis purification is critical due to the compound’s sensitivity to oxidation. Reverse-phase HPLC (C18 column) with a gradient of acetonitrile/water (0.1% formic acid) achieves >98% purity. Structural confirmation relies on:
-
Mass Spectrometry (HRMS): m/z 385.57 [M+H]⁺
-
NMR (¹H, ¹³C): δ 5.3–5.4 ppm (arachidonoyl olefins), δ 3.6–3.8 ppm (dihydroxypropyl backbone)
Challenges and Mitigation Strategies
Arachidonic Acid Oxidation
The polyunsaturated arachidonoyl chain is prone to peroxidation. Strategies include:
-
Conducting reactions under argon with chelating agents (e.g., EDTA)
Scalability Limitations
Industrial-scale production faces hurdles in enzymatic cost and arachidonic acid availability. Hybrid approaches, such as chemoenzymatic cascades, are under investigation to balance efficiency and scalability .
Chemical Reactions Analysis
Types of Reactions: Arachidonoyl Serinol can undergo various chemical reactions, including:
Oxidation: The double bonds in the arachidonoyl chain can be oxidized to form epoxides or hydroxylated derivatives.
Reduction: The double bonds can also be reduced to form saturated derivatives.
Substitution: The hydroxyl groups in the serinol moiety can participate in substitution reactions to form esters or ethers.
Common Reagents and Conditions:
Oxidation: Reagents such as m-chloroperbenzoic acid (m-CPBA) or osmium tetroxide (OsO4) can be used for oxidation reactions.
Reduction: Hydrogenation using palladium on carbon (Pd/C) as a catalyst can be employed for reduction reactions.
Substitution: Acid chlorides or anhydrides can be used for esterification, while alkyl halides can be used for etherification.
Major Products:
Oxidation: Epoxides, hydroxylated derivatives.
Reduction: Saturated derivatives.
Substitution: Esters, ethers.
Scientific Research Applications
Chemical Properties and Mechanism of Action
NADOPA features a dihydroxypropylamine group attached to an arachidonoyl chain, which is notable for its multiple double bonds that contribute to its biochemical reactivity. The compound primarily acts as an agonist for the cannabinoid receptors (CB1 and CB2) and the transient receptor potential vanilloid 1 (TRPV1) ion channel. This interaction is critical in modulating pain perception, inflammation, and neuroprotection .
Biochemical Research
NADOPA serves as a model compound for studying endocannabinoid-like molecules. Its interactions with various receptors provide insights into lipid metabolism and cellular signaling pathways. Researchers utilize NADOPA to explore its effects on enzyme activity, gene expression, and cellular metabolism.
Neurobiology
NADOPA has been investigated for its neuroprotective properties. Studies demonstrate that it can stabilize hypoxia-inducible factor-1α (HIF-1α), promoting neuronal survival under oxidative stress conditions. This stabilization is independent of CB1 and TRPV1 receptor activation, suggesting alternative pathways for neuroprotection .
Inflammation Modulation
NADOPA exhibits significant anti-inflammatory effects. In vivo studies have shown that it reduces systemic inflammatory responses in mouse models challenged with lipopolysaccharides (LPS) or bacterial lipopeptides. Notably, NADOPA administration increased survival rates in endotoxemic mice by decreasing levels of pro-inflammatory cytokines and enhancing anti-inflammatory cytokine production .
Potential Therapeutic Applications
The compound's ability to modulate pain and inflammation positions it as a candidate for therapeutic strategies against neuroinflammatory diseases. Its dual action on cannabinoid receptors and TRPV1 suggests potential utility in managing conditions such as chronic pain and neurodegenerative disorders .
Data Tables
Case Studies
Case Study 1: Anti-inflammatory Effects in Mice
In a controlled study, NADOPA was administered to mice subjected to LPS-induced inflammation. Results indicated a significant reduction in plasma concentrations of inflammatory mediators such as IL-6 and CCL2, alongside an increase in the anti-inflammatory cytokine IL-10. The study concluded that NADOPA could be a novel therapeutic agent for acute inflammatory conditions .
Case Study 2: Neuroprotective Properties
Research involving human-induced pluripotent stem cell-derived neurons demonstrated that NADOPA enhances the expression of neurotrophic factors like BDNF and GDNF under oxidative stress conditions. This effect suggests that NADOPA may facilitate neuronal health by promoting survival pathways through enhanced growth factor signaling .
Mechanism of Action
Arachidonoyl Serinol exerts its effects by interacting with various molecular targets and pathways. It has been shown to activate N-type calcium channels in sympathetic neurons, leading to an increase in calcium current . Unlike traditional endocannabinoids, Arachidonoyl Serinol does not act through CB1 or CB2 receptors but may interact with a novel G-protein-coupled receptor . This unique mechanism of action makes it a valuable tool for studying the endocannabinoid system and its physiological effects.
Comparison with Similar Compounds
Structural and Functional Comparison with Analogous Compounds
Structural Features
The dihydroxypropylamine group distinguishes N-arachidonoyl dihydroxypropylamine from other arachidonoyl derivatives. Key structural comparisons include:
Compound | Formula | Molecular Weight | Key Functional Group |
---|---|---|---|
This compound | C₂₃H₃₉NO₃ | 377.29 | 1,3-Dihydroxypropylamine |
Anandamide (AEA) | C₂₂H₃₇NO₂ | 347.27 | Ethanolamide |
2-Arachidonoylglycerol (2-AG) | C₂₃H₃₈O₄ | 378.27 | Glycerol ester |
N-Arachidonoyl GABA (NA-GABA) | C₂₄H₃₉NO₃ | 389.29 | γ-aminobutyric acid (GABA) |
N-Arachidonoyl dopamine (NADA) | C₂₆H₃₇NO₃ | 411.28 | Dopamine conjugate |
Key Observations :
- The dihydroxypropylamine group introduces two hydroxyl groups, enhancing hydrophilicity compared to AEA (ethanolamide) or 2-AG (glycerol ester) .
Receptor Interactions and Signaling Pathways
- Cannabinoid Receptors (CB1/CB2): AEA and 2-AG are canonical endocannabinoids that activate CB1/CB2 with varying efficacy. AEA acts as a partial agonist, while 2-AG is a full agonist .
- TRPV1 Channels: NADA is a dual CB1/TRPV1 ligand, with strong TRPV1 agonism contributing to pain modulation . The hydroxyl groups in this compound could facilitate TRPV1 interactions, analogous to NADA’s catechol moiety .
Metabolic Stability and Enzymatic Degradation
Endocannabinoids are rapidly inactivated by enzymes like fatty acid amide hydrolase (FAAH) and monoacylglycerol lipase (MAGL):
- AEA : Primarily degraded by FAAH .
- 2-AG : Hydrolyzed by MAGL .
- This compound: The dihydroxypropylamine group may confer resistance to FAAH or MAGL, prolonging its half-life. However, this hypothesis requires experimental validation.
Physicochemical and Pharmacokinetic Properties
The hydroxyl groups in this compound likely increase aqueous solubility compared to AEA or 2-AG, which are highly lipophilic. This property could influence its distribution in aqueous compartments (e.g., cerebrospinal fluid) or reduce blood-brain barrier penetration .
Q & A
Q. Basic: What analytical techniques are recommended for determining the chemical structure of N-arachidonoyl dihydroxypropylamine?
Answer:
The chemical structure (C₂₃H₃₉NO₃) can be confirmed using tandem mass spectrometry (MS/MS) and nuclear magnetic resonance (NMR) spectroscopy. For example:
- Mass spectrometry : Use electrospray ionization (ESI) coupled with MSⁿ to fragment the molecule and identify characteristic ions (e.g., m/z 378.3 for [M+H]⁺) .
- NMR : Assign peaks for the arachidonoyl chain (δ 0.8–2.8 ppm for aliphatic protons) and dihydroxypropylamine moiety (δ 3.5–4.5 ppm for hydroxyl and amine groups) .
Q. Basic: How can researchers detect this compound in biological matrices?
Answer:
Liquid chromatography-tandem mass spectrometry (LC-MS/MS) is optimal for quantification:
- Sample preparation : Lipid extraction via Folch or Bligh-Dyer methods, followed by solid-phase extraction to isolate N-acylamines .
- Chromatography : Use a C18 column with gradient elution (mobile phase: 0.1% formic acid in water/acetonitrile).
- Detection : Monitor transitions specific to the molecular ion (e.g., m/z 378 → 255 for arachidonate cleavage) .
Q. Advanced: What experimental strategies can elucidate the role of fatty acid amide hydrolase (FAAH) in metabolizing this compound?
Answer:
- Inhibition assays : Treat cell lysates or tissue homogenates with FAAH inhibitors (e.g., URB597) and measure hydrolysis products via LC-MS/MS .
- Kinetic studies : Calculate and using recombinant FAAH and synthetic this compound as a substrate.
- Genetic models : Compare metabolite levels in FAAH-knockout vs. wild-type mice to assess enzymatic specificity .
Q. Advanced: How can researchers investigate interactions between this compound and transient receptor potential (TRP) channels?
Answer:
- Calcium imaging : Use HEK293 cells transfected with TRPV1 or TRPA1 channels. Measure intracellular Ca²⁺ flux (Fluo-4 dye) upon compound exposure .
- Electrophysiology : Perform patch-clamp recordings to assess channel activation/inhibition thresholds.
- Competitive binding : Co-incubate with known TRP ligands (e.g., capsaicin) to identify allosteric effects .
Q. Advanced: How should contradictions in reported tissue-specific concentrations of this compound be resolved?
Answer:
- Standardized protocols : Ensure consistent sample collection (e.g., rapid freezing to prevent enzymatic degradation) and lipid extraction methods .
- Inter-laboratory validation : Share reference samples between labs to calibrate LC-MS/MS systems.
- Model adjustments : Account for regional differences in lipid metabolism (e.g., brain vs. peripheral tissues) using multivariate statistical models .
Q. Basic: What are critical handling considerations for this compound in experimental settings?
Answer:
- Solubility : Prepare stock solutions in ethanol or DMSO (purged with inert gas) to prevent oxidation. For aqueous buffers, use colloidal suspensions (e.g., 1:1 ethanol:PBS) with sonication .
- Storage : Store at −80°C under nitrogen to avoid hydrolysis. Avoid freeze-thaw cycles .
Q. Advanced: How can receptor binding assays be optimized to study this compound’s affinity for cannabinoid receptors?
Answer:
- Radioligand displacement : Use [³H]CP-55,940 in synaptosomal membranes. Calculate IC₅₀ values via competitive binding curves .
- Cell-based assays : Transfect CHO cells with CB1/CB2 receptors and measure cAMP inhibition (ELISA) or β-arrestin recruitment (BRET) .
- Negative controls : Include anandamide (AEA) and 2-AG as reference ligands to validate assay specificity .
Q. Advanced: What methodologies assess the stability of this compound under varying physiological conditions?
Answer:
- pH stability : Incubate the compound in buffers (pH 4–9) and quantify degradation via LC-MS/MS over time.
- Enzymatic profiling : Expose to esterases, lipases, or serum to identify susceptibility pathways .
- Oxidative stress tests : Measure lipid peroxidation products (e.g., malondialdehyde) under high oxygen tension .
Properties
CAS No. |
183718-70-9 |
---|---|
Molecular Formula |
C23H39NO3 |
Molecular Weight |
377.6 g/mol |
IUPAC Name |
N-(1,3-dihydroxypropan-2-yl)icosa-5,8,11,14-tetraenamide |
InChI |
InChI=1S/C23H39NO3/c1-2-3-4-5-6-7-8-9-10-11-12-13-14-15-16-17-18-19-23(27)24-22(20-25)21-26/h6-7,9-10,12-13,15-16,22,25-26H,2-5,8,11,14,17-21H2,1H3,(H,24,27) |
InChI Key |
QHELXIATGZYOIB-UHFFFAOYSA-N |
SMILES |
CCCCCC=CCC=CCC=CCC=CCCCC(=O)NC(CO)CO |
Isomeric SMILES |
CCCCC/C=C\C/C=C\C/C=C\C/C=C\CCCC(=O)NC(CO)CO |
Canonical SMILES |
CCCCCC=CCC=CCC=CCC=CCCCC(=O)NC(CO)CO |
Appearance |
Assay:≥98%A solution in ethanol |
physical_description |
Solid |
Synonyms |
N-[(2-hydroxy-1-hydroxymethyl)ethyl]-5Z,8Z,11Z,14Z-eicosatetraenamide |
Origin of Product |
United States |
Retrosynthesis Analysis
AI-Powered Synthesis Planning: Our tool employs the Template_relevance Pistachio, Template_relevance Bkms_metabolic, Template_relevance Pistachio_ringbreaker, Template_relevance Reaxys, Template_relevance Reaxys_biocatalysis model, leveraging a vast database of chemical reactions to predict feasible synthetic routes.
One-Step Synthesis Focus: Specifically designed for one-step synthesis, it provides concise and direct routes for your target compounds, streamlining the synthesis process.
Accurate Predictions: Utilizing the extensive PISTACHIO, BKMS_METABOLIC, PISTACHIO_RINGBREAKER, REAXYS, REAXYS_BIOCATALYSIS database, our tool offers high-accuracy predictions, reflecting the latest in chemical research and data.
Strategy Settings
Precursor scoring | Relevance Heuristic |
---|---|
Min. plausibility | 0.01 |
Model | Template_relevance |
Template Set | Pistachio/Bkms_metabolic/Pistachio_ringbreaker/Reaxys/Reaxys_biocatalysis |
Top-N result to add to graph | 6 |
Feasible Synthetic Routes
Disclaimer and Information on In-Vitro Research Products
Please be aware that all articles and product information presented on BenchChem are intended solely for informational purposes. The products available for purchase on BenchChem are specifically designed for in-vitro studies, which are conducted outside of living organisms. In-vitro studies, derived from the Latin term "in glass," involve experiments performed in controlled laboratory settings using cells or tissues. It is important to note that these products are not categorized as medicines or drugs, and they have not received approval from the FDA for the prevention, treatment, or cure of any medical condition, ailment, or disease. We must emphasize that any form of bodily introduction of these products into humans or animals is strictly prohibited by law. It is essential to adhere to these guidelines to ensure compliance with legal and ethical standards in research and experimentation.