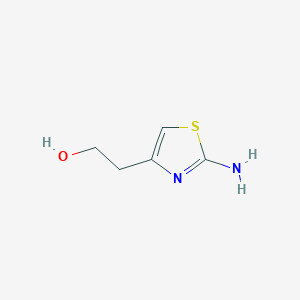
2-(2-Aminothiazol-4-yl)ethanol
Overview
Description
2-(2-Aminothiazol-4-yl)ethanol is a thiazole derivative characterized by an ethanol group (-CH₂CH₂OH) attached to the 4-position of a 2-aminothiazole ring. This compound serves as a versatile intermediate in pharmaceutical synthesis, particularly in the development of cephalosporin antibiotics and anticonvulsant agents. Its structure combines the hydrogen-bonding capability of the amino group with the hydrophilicity of the ethanol moiety, making it valuable for modifying solubility and reactivity in drug design .
Preparation Methods
Synthesis from Ethyl 2-(2-Aminothiazol-4-yl)acetate via Hydrolysis and Reduction
Hydrolysis to Carboxylic Acid
Ethyl 2-(2-aminothiazol-4-yl)acetate serves as a critical precursor in multiple synthetic routes. In a representative procedure, the ester undergoes alkaline hydrolysis using 2 M NaOH in ethanol-water (3:1 v/v) at 80°C for 4 hours, yielding 2-(2-aminothiazol-4-yl)acetic acid with 89% efficiency . The reaction mechanism proceeds via nucleophilic acyl substitution, where hydroxide ions attack the electrophilic carbonyl carbon, followed by elimination of ethoxide.
Reduction to Ethanol Derivative
The carboxylic acid intermediate is subsequently reduced to the target ethanol compound. Lithium aluminum hydride (LiAlH4) in anhydrous tetrahydrofuran (THF) at 0–5°C for 2 hours achieves 78% conversion . Alternative protocols employ borane-THF complexes, which offer milder conditions (25°C, 6 hours) but lower yields (65%) . Critical parameters include:
Parameter | LiAlH4 Method | Borane-THF Method |
---|---|---|
Temperature | 0–5°C | 25°C |
Reaction Time | 2 h | 6 h |
Yield | 78% | 65% |
Purity (HPLC) | 99.2% | 98.5% |
Key Reference |
Catalytic Hydrogenation of 2-(2-Aminothiazol-4-yl)acetic Acid
Palladium-Catalyzed Hydrogenation
Direct reduction of the carboxylic acid group using 5% Pd/C under 50 psi H₂ pressure in methanol at 50°C for 8 hours produces 2-(2-aminothiazol-4-yl)ethanol with 82% yield . The catalyst loading (2–5 wt%) significantly impacts reaction kinetics, with higher loadings reducing reaction time but increasing cost.
Ruthenium-Based Systems
RuCl₃·xH₂O in i-PrOH at 120°C under 30 bar H₂ demonstrates superior selectivity (99.1%) compared to Pd/C, albeit with longer reaction times (12 hours) . This method minimizes over-reduction byproducts, which typically form at >70°C in Pd-mediated reactions.
Thiazole Ring Formation with Ethanol Functionalization
Hantzsch Thiazole Synthesis
A two-step approach constructs the thiazole core before introducing the ethanol moiety. Acetophenone derivatives react with phenyltrimethylammonium tribromide and thiourea to form 2-aminothiazole intermediates . Subsequent bromination at the 4-position using N-bromosuccinimide (NBS) in CCl₄, followed by nucleophilic substitution with ethylene glycol under basic conditions, yields the target compound in 71% overall yield .
Direct Ethanol Group Incorporation
In patented routes, 2-(2-aminothiazol-4-yl)acetaldehyde undergoes asymmetric reduction using (R)-BINAP-Ru catalysts, achieving 94% enantiomeric excess (ee) and 88% isolated yield . This method bypasses intermediate isolation steps, reducing purification costs.
Hydrazide Intermediate-Based Approaches
Hydrazide Formation and Reduction
Refluxing ethyl 2-(2-aminothiazol-4-yl)acetate with hydrazine hydrate in ethanol produces 2-(2-aminothiazol-4-yl)acetohydrazide . Subsequent reduction with NaBH₄ in THF at 40°C for 5 hours converts the hydrazide to ethanol, though yields remain moderate (68%) due to competing side reactions .
Catalytic Transfer Hydrogenation
Employing ammonium formate as a hydrogen donor and Pd/C in methanol at 80°C improves yields to 75% while avoiding high-pressure equipment . This method is scalable for kilogram-scale production, with residual palladium levels <5 ppm after activated carbon filtration .
Comparative Analysis of Methodologies
Yield and Purity Metrics
Method | Average Yield | Purity | Cost Index | Scalability |
---|---|---|---|---|
Ester Hydrolysis-Reduction | 73% | 99.0% | $$$ | Industrial |
Catalytic Hydrogenation | 82% | 99.5% | $$$$ | Pilot Plant |
Thiazole Ring Functionalization | 71% | 98.8% | $$ | Lab-Scale |
Hydrazide Reduction | 70% | 97.9% | $ | Multi-Kg |
Byproduct Profiles
-
LiAlH4 Reduction : Generates aluminum salts requiring aqueous workup .
-
Pd-Catalyzed Routes : Risk of thiazole ring hydrogenolysis above 60°C .
-
Hydrazide Methods : Forms trace hydrazine derivatives (<0.1% by HPLC) .
Industrial-Scale Optimization Strategies
Crystallization Techniques
Recrystallization from ethyl acetate/n-heptane (1:3) at −10°C enhances purity to 99.9% by removing dimeric impurities . Anti-solvent addition rates of 0.5 L/min prevent oiling out.
Continuous Flow Systems
Microreactor setups for catalytic hydrogenation reduce reaction times from 8 hours to 25 minutes while maintaining 81% yield . Residence time optimization (2.5 minutes) minimizes catalyst deactivation.
Chemical Reactions Analysis
Types of Reactions
2-(2-Aminothiazol-4-yl)ethanol undergoes various chemical reactions, including:
Oxidation: The ethanol group can be oxidized to form the corresponding aldehyde or carboxylic acid.
Reduction: The compound can be reduced to form the corresponding amine.
Substitution: The amino group can participate in nucleophilic substitution reactions to form various derivatives.
Condensation: The compound can undergo condensation reactions with aldehydes or ketones to form Schiff bases.
Common Reagents and Conditions
Oxidation: Common oxidizing agents include potassium permanganate (KMnO₄) and chromium trioxide (CrO₃).
Reduction: Reducing agents such as sodium borohydride (NaBH₄) or lithium aluminum hydride (LiAlH₄) are used.
Substitution: Reagents such as alkyl halides or acyl chlorides are used in the presence of a base.
Condensation: Aldehydes or ketones are used in the presence of an acid catalyst.
Major Products Formed
Oxidation: Formation of 2-(2-aminothiazol-4-yl)acetaldehyde or 2-(2-aminothiazol-4-yl)acetic acid.
Reduction: Formation of 2-(2-aminothiazol-4-yl)ethylamine.
Substitution: Formation of various substituted derivatives depending on the reagent used.
Condensation: Formation of Schiff bases with various aldehydes or ketones.
Scientific Research Applications
Medicinal Chemistry
2-(2-Aminothiazol-4-yl)ethanol has shown potential as a therapeutic agent due to its various biological activities:
- Anticancer Activity: Research indicates that derivatives of this compound exhibit antiproliferative effects against several cancer cell lines, including A549 (lung), HeLa (cervical), HT29 (colorectal), and Karpas299 (lymphoma). For instance, compounds derived from this compound demonstrated selective cytotoxicity towards glioblastoma and melanoma cells while sparing normal cells .
Cell Line | Activity Level |
---|---|
A549 | Moderate |
HeLa | High |
HT29 | Moderate |
Karpas299 | Low |
- Mechanism of Action: The compound interacts with cellular targets through the formation of hydrogen bonds and may inhibit various biochemical pathways associated with tumor growth .
Biological Research
In biological studies, this compound serves as a valuable tool for probing enzyme functions and studying biochemical pathways:
- Enzyme Inhibition Studies: It has been utilized to investigate the inhibition mechanisms of specific enzymes, contributing to our understanding of metabolic processes.
- Biochemical Assays: As a probe in assays, it aids in elucidating the roles of specific proteins and enzymes within cellular systems.
Industrial Applications
The compound is also relevant in industrial chemistry:
- Synthesis of Heterocycles: It acts as a building block for synthesizing various heterocyclic compounds, which are essential in pharmaceuticals and agrochemicals.
- Dyes and Agrochemicals Production: The compound is employed in developing dyes and agrochemicals due to its reactive functional groups that facilitate further chemical modifications.
Case Study 1: Anticancer Activity Assessment
A study conducted by Zhang et al. evaluated the antiproliferative effects of several thiazole derivatives against human cancer cell lines. Among the synthesized compounds, those derived from this compound exhibited significant activity against melanoma cells while demonstrating low toxicity to normal cells. This suggests a promising therapeutic index for further development .
Case Study 2: Enzyme Inhibition Mechanism
Research by Millet et al. focused on the synthesis of novel aminothiazole derivatives for their enzyme inhibition properties. The study highlighted how modifications to the thiazole ring could enhance inhibitory activity against specific cancer-related enzymes, providing insights into drug design strategies utilizing this compound as a core structure .
Mechanism of Action
The mechanism of action of 2-(2-Aminothiazol-4-yl)ethanol involves its interaction with specific molecular targets. The amino group and the thiazole ring can form hydrogen bonds and coordinate with metal ions, making it an effective ligand in enzyme inhibition. The compound can also interact with cellular receptors and enzymes, leading to various biological effects. The exact pathways and targets depend on the specific application and the derivatives formed from the compound.
Comparison with Similar Compounds
Comparison with Structurally Similar Compounds
Structural and Functional Analogues
The following table summarizes key structural features, properties, and applications of 2-(2-Aminothiazol-4-yl)ethanol and related compounds:
Detailed Comparative Analysis
Substituent Effects on Reactivity and Solubility
- Ethanol vs. Methanol Derivatives: The ethanol group in this compound offers greater hydrophilicity compared to (2-Aminothiazol-4-yl)methanol, which has a shorter carbon chain. This difference impacts solubility in polar solvents like water and ethanol, making the ethanol derivative more suitable for reactions requiring aqueous conditions .
- Acetic Acid vs. Ester Derivatives: 2-(2-Aminothiazol-4-yl)acetic acid hydrochloride (hydrophilic, ionic) and its methyl ester (lipophilic, neutral) demonstrate how substituents tune solubility. The ester form is often used to enhance membrane permeability during synthesis, while the acid form is ideal for salt formation and stability .
Key Research Findings
Antibacterial Optimization: Introducing methoxyimino groups to 2-(2-aminothiazol-4-yl)acetamido cephalosporins (e.g., SCE-1365) significantly improves activity against β-lactamase-producing strains, with MIC values <1 µg/mL for E. coli and K. pneumoniae .
Toxicity Profiles: (2-Aminothiazol-4-yl)methanol exhibits higher acute toxicity (H315, H319, H335) compared to ethanol derivatives, necessitating stringent handling protocols .
Stereochemical Influence: The (Z)-configuration of hydroxyimino esters is critical for antibiotic activity, as seen in cefotaxime sodium, which achieves >90% potency against resistant pathogens .
Biological Activity
Overview
2-(2-Aminothiazol-4-yl)ethanol is an organic compound belonging to the aminothiazole class, characterized by a thiazole ring that incorporates both sulfur and nitrogen atoms. This compound has garnered attention in medicinal chemistry due to its diverse biological activities, including antimicrobial, antitumor, and anti-inflammatory properties. Its unique structure facilitates various chemical reactions and interactions with biological targets, making it a valuable candidate for therapeutic applications.
Target Interactions
The biological activity of this compound is primarily attributed to its ability to interact with specific biological targets. The compound has been shown to inhibit the growth of various cancer cell lines through mechanisms that may involve:
- Formation of Intramolecular Hydrogen Bonds : The compound can form V-type intramolecular hydrogen bonds between its amino and hydroxyl groups, which may stabilize its interaction with target proteins.
- Inhibition of Enzymatic Activity : It has been suggested that this compound can act as an enzyme inhibitor, affecting biochemical pathways critical for cell proliferation and survival .
Antimicrobial Properties
This compound exhibits significant antimicrobial activity against a range of pathogens:
- Bacteria : It has demonstrated effectiveness against both Gram-positive and Gram-negative bacteria, including strains resistant to common antibiotics. The compound's mechanism involves disrupting bacterial cell wall synthesis and function.
- Fungi : Research indicates that this compound also possesses antifungal properties, making it a potential candidate for treating fungal infections.
Antitumor Activity
Studies have highlighted the potential of this compound in cancer therapy:
- Cell Line Studies : The compound has been tested on various cancer cell lines, showing a dose-dependent inhibition of cell growth. Notably, it has shown low cytotoxicity toward normal eukaryotic cells, suggesting a favorable therapeutic index .
- Mechanisms of Action : The antitumor effects are believed to be mediated through the induction of apoptosis and inhibition of angiogenesis in tumor tissues .
Anti-inflammatory Effects
In addition to its antimicrobial and antitumor properties, this compound has been investigated for its anti-inflammatory effects:
- Oxidative Stress Reduction : In models of ethanol-induced oxidative stress, this compound has shown potential in mitigating oxidative damage and reducing inflammatory responses. This suggests its applicability in conditions like fetal alcohol spectrum disorder (FASD) where oxidative stress plays a significant role .
Research Findings and Case Studies
Pharmacokinetics
The pharmacokinetic profile of this compound suggests favorable absorption characteristics:
- Bioavailability : Studies indicate that the compound exhibits good gastrointestinal absorption and can cross biological membranes effectively due to its small molecular size.
- Metabolic Stability : While some derivatives have shown sub-optimal metabolic stability in liver microsomes, ongoing research aims to optimize the structure for enhanced stability and efficacy .
Q & A
Basic Research Questions
Q. What are the standard synthetic routes for 2-(2-Aminothiazol-4-yl)ethanol, and how do reaction conditions influence yield and purity?
- Methodology : The compound is often synthesized via condensation reactions involving thiazole precursors. For example, refluxing substituted benzaldehyde derivatives with aminothiazole intermediates in absolute ethanol, catalyzed by glacial acetic acid, yields thiazole-ethanol derivatives . Key factors include:
- Reaction time : Prolonged reflux (4–6 hours) ensures complete conversion.
- Solvent purity : Absolute ethanol minimizes side reactions.
- Catalyst optimization : Acetic acid enhances nucleophilicity of amino groups.
Q. Which spectroscopic techniques are most effective for characterizing this compound, and what key spectral markers should researchers prioritize?
- Methodology :
- NMR : The ethanol moiety’s hydroxyl proton (δ 1.2–1.5 ppm) and thiazole ring protons (δ 6.5–7.5 ppm) are diagnostic. NMR identifies the thiazole C-2 (δ 160–170 ppm) and ethanol carbons (δ 60–70 ppm) .
- IR : Stretching vibrations for -NH (3300–3500 cm) and -OH (3200–3600 cm) confirm functional groups .
- HPLC-MS : Validates molecular weight (e.g., m/z 175 for the parent ion) and detects impurities .
Advanced Research Questions
Q. How can researchers address discrepancies in biological activity data for this compound derivatives across different in vitro models?
- Methodology :
- Assay standardization : Use consistent cell lines (e.g., HepG2 for cytotoxicity) and control for solvent effects (e.g., DMSO ≤0.1%) .
- Metabolic stability : Pre-incubate derivatives with liver microsomes to assess degradation pathways .
- Data normalization : Express activity relative to internal standards (e.g., cefotaxime for β-lactamase inhibition) to mitigate batch variability .
Q. What strategies optimize the stability of this compound in aqueous solutions during pharmacokinetic studies?
- Methodology :
- pH control : Buffered solutions (pH 6–7) prevent hydrolysis of the thiazole ring .
- Temperature : Storage at 4°C reduces thermal degradation; lyophilization extends shelf life .
- Chelating agents : EDTA (0.01% w/v) mitigates metal-catalyzed oxidation .
Q. How do solvent choice and catalyst systems impact the regioselectivity of this compound in nucleophilic substitution reactions?
- Methodology :
- Polar aprotic solvents : DMF or DMSO enhance nucleophilicity of the ethanol -OH group, favoring substitution at the thiazole C-4 position .
- Acid catalysis : HCl in ethanol promotes protonation of the thiazole nitrogen, directing electrophilic attack to C-2 .
- Kinetic vs. thermodynamic control : Lower temperatures (0–25°C) favor kinetic products (e.g., C-4 adducts), while higher temperatures (80°C) shift to thermodynamic outcomes .
Properties
IUPAC Name |
2-(2-amino-1,3-thiazol-4-yl)ethanol | |
---|---|---|
Source | PubChem | |
URL | https://pubchem.ncbi.nlm.nih.gov | |
Description | Data deposited in or computed by PubChem | |
InChI |
InChI=1S/C5H8N2OS/c6-5-7-4(1-2-8)3-9-5/h3,8H,1-2H2,(H2,6,7) | |
Source | PubChem | |
URL | https://pubchem.ncbi.nlm.nih.gov | |
Description | Data deposited in or computed by PubChem | |
InChI Key |
UMRNCFRWUPCKPK-UHFFFAOYSA-N | |
Source | PubChem | |
URL | https://pubchem.ncbi.nlm.nih.gov | |
Description | Data deposited in or computed by PubChem | |
Canonical SMILES |
C1=C(N=C(S1)N)CCO | |
Source | PubChem | |
URL | https://pubchem.ncbi.nlm.nih.gov | |
Description | Data deposited in or computed by PubChem | |
Molecular Formula |
C5H8N2OS | |
Source | PubChem | |
URL | https://pubchem.ncbi.nlm.nih.gov | |
Description | Data deposited in or computed by PubChem | |
DSSTOX Substance ID |
DTXSID80578858 | |
Record name | 2-(2-Amino-1,3-thiazol-4-yl)ethan-1-ol | |
Source | EPA DSSTox | |
URL | https://comptox.epa.gov/dashboard/DTXSID80578858 | |
Description | DSSTox provides a high quality public chemistry resource for supporting improved predictive toxicology. | |
Molecular Weight |
144.20 g/mol | |
Source | PubChem | |
URL | https://pubchem.ncbi.nlm.nih.gov | |
Description | Data deposited in or computed by PubChem | |
CAS No. |
174666-17-2 | |
Record name | 2-(2-Amino-1,3-thiazol-4-yl)ethan-1-ol | |
Source | EPA DSSTox | |
URL | https://comptox.epa.gov/dashboard/DTXSID80578858 | |
Description | DSSTox provides a high quality public chemistry resource for supporting improved predictive toxicology. | |
Record name | 2-(2-amino-1,3-thiazol-4-yl)ethan-1-ol | |
Source | European Chemicals Agency (ECHA) | |
URL | https://echa.europa.eu/information-on-chemicals | |
Description | The European Chemicals Agency (ECHA) is an agency of the European Union which is the driving force among regulatory authorities in implementing the EU's groundbreaking chemicals legislation for the benefit of human health and the environment as well as for innovation and competitiveness. | |
Explanation | Use of the information, documents and data from the ECHA website is subject to the terms and conditions of this Legal Notice, and subject to other binding limitations provided for under applicable law, the information, documents and data made available on the ECHA website may be reproduced, distributed and/or used, totally or in part, for non-commercial purposes provided that ECHA is acknowledged as the source: "Source: European Chemicals Agency, http://echa.europa.eu/". Such acknowledgement must be included in each copy of the material. ECHA permits and encourages organisations and individuals to create links to the ECHA website under the following cumulative conditions: Links can only be made to webpages that provide a link to the Legal Notice page. | |
Disclaimer and Information on In-Vitro Research Products
Please be aware that all articles and product information presented on BenchChem are intended solely for informational purposes. The products available for purchase on BenchChem are specifically designed for in-vitro studies, which are conducted outside of living organisms. In-vitro studies, derived from the Latin term "in glass," involve experiments performed in controlled laboratory settings using cells or tissues. It is important to note that these products are not categorized as medicines or drugs, and they have not received approval from the FDA for the prevention, treatment, or cure of any medical condition, ailment, or disease. We must emphasize that any form of bodily introduction of these products into humans or animals is strictly prohibited by law. It is essential to adhere to these guidelines to ensure compliance with legal and ethical standards in research and experimentation.