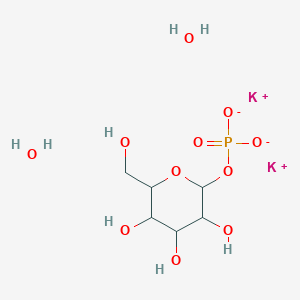
Dipotassium
Overview
Description
Dipotassium compounds are a class of chemical salts characterized by the presence of two potassium cations (K⁺) paired with a polyatomic or organic anion. These compounds exhibit diverse chemical and physical properties depending on the anion's structure, influencing their applications in industries such as agriculture, pharmaceuticals, food additives, and materials science. Common examples include:
- This compound Phosphate (K₂HPO₄): A buffering agent in food and fertilizers.
- Rhodizonic Acid this compound Salt (C₆O₆K₂): Used in analytical chemistry and industrial processes.
- This compound Glycyrrhizinate: A bioactive compound in cosmetics and pharmaceuticals.
This article focuses on comparing these this compound compounds with structurally or functionally similar salts, such as monopotassium, disodium, and other alkali metal salts, to highlight key differences in properties and applications.
Preparation Methods
Synthetic Routes and Reaction Conditions
Galactose 1-phosphate Potassium salt can be synthesized enzymatically through the phosphorolysis of lactose using lactose phosphorylase enzymes . This method involves the use of permeabilized Escherichia coli cells containing the lactose phosphorylase enzyme, which catalyzes the reaction to produce α-D-Galactose-1-phosphate and glucose . The product is then purified from the reaction mixture by anion-exchange chromatography and ethanol precipitation .
Industrial Production Methods
Industrial production of Galactose 1-phosphate Potassium salt typically involves the use of biocatalysts and fermentation processes. The use of engineered microorganisms, such as Escherichia coli, allows for the efficient production of the compound from inexpensive starting materials like lactose .
Chemical Reactions Analysis
Types of Reactions
Galactose 1-phosphate Potassium salt undergoes various chemical reactions, including:
Phosphorolysis: The enzymatic cleavage of lactose to produce α-D-Galactose-1-phosphate and glucose.
Substitution Reactions: Involving the exchange of phosphate groups with other functional groups.
Common Reagents and Conditions
Lactose Phosphorylase: Used in the enzymatic production of α-D-Galactose-1-phosphate.
Anion-Exchange Chromatography: For the purification of the product.
Ethanol Precipitation: To isolate the pure compound.
Major Products Formed
The primary product formed from the phosphorolysis of lactose is α-D-Galactose-1-phosphate, along with glucose as a byproduct .
Scientific Research Applications
Agricultural Applications
Fertilizer Use
Dipotassium phosphate serves as an effective fertilizer due to its high content of essential nutrients—potassium (K) and phosphorus (P). It provides approximately 41% available phosphate (P₂O₅) and 54% soluble potash (K₂O), making it a valuable nutrient source for plant growth. Its applications include:
- Foliar Application : Enhances nutrient uptake through leaves, promoting rapid growth.
- Soil Drench : Delivers nutrients directly to the root zone, improving root development.
- Disease Resistance : Enhances plant defense mechanisms against fungal diseases, particularly in crops like ornamentals and non-bearing fruit trees.
Table 1: Nutritional Composition of this compound Phosphate
Nutrient | Percentage |
---|---|
Available Phosphate (P₂O₅) | 41% |
Soluble Potash (K₂O) | 54% |
Food Industry Applications
This compound phosphate is extensively used in the food industry for its functional properties, including stabilizing, emulsifying, and leavening agents. Key applications include:
- Buffering Agent : Maintains pH levels in dairy products such as cheese and yogurt, ensuring consistent texture and flavor.
- Non-Dairy Creamers : Prevents coagulation, providing a smooth texture in dairy alternatives.
- Baked Goods : Acts as a leavening agent to improve the rise and texture of bread and cakes.
Case Study: Dairy Products
Numerous studies have shown that the inclusion of this compound phosphate in dairy products enhances quality by improving texture and shelf life. For instance, its use in cheese production has been linked to better moisture retention and flavor stability .
Table 2: Functions of this compound Phosphate in Food Products
Function | Application |
---|---|
Stabilizer | Preserves texture in sauces and dressings |
Emulsifier | Blends oil and water in food products |
Leavening Agent | Contributes to rise in baked goods |
Pharmaceutical Applications
In the pharmaceutical sector, this compound phosphate is utilized primarily as a buffering agent in drug formulations. Its role ensures that medications maintain optimal pH levels for efficacy and stability. Additionally, it is included in nutrient solutions for intravenous therapies to provide essential electrolytes.
Case Study: Nutrient Solutions
Research has demonstrated that this compound phosphate is critical in total parenteral nutrition (TPN), where it supports patients unable to consume food orally by providing vital nutrients .
Health Supplements
This compound phosphate is found in various health supplements, particularly protein powders and energy drinks. Its potassium content is beneficial for athletes, aiding muscle recovery and performance enhancement.
Table 3: Applications of this compound Phosphate in Health Supplements
Product Type | Benefit |
---|---|
Protein Powders | Supports muscle recovery |
Energy Drinks | Enhances performance |
Total Parenteral Nutrition | Provides essential nutrients for patients |
Mechanism of Action
Galactose 1-phosphate Potassium salt exerts its effects through its role as an intermediate in the galactose metabolism pathway. It is produced from galactose by the enzyme galactokinase and is further converted to glucose-1-phosphate and UDP-galactose by the enzyme galactose-1-phosphate uridyltransferase . This conversion is crucial for the proper metabolism of galactose and the synthesis of nucleotide sugars .
Comparison with Similar Compounds
Comparison with Monopotassium Compounds
Dipotassium Phosphate (K₂HPO₄) vs. Monopotassium Phosphate (KH₂PO₄)
Both are phosphate salts but differ in potassium content and acidity:
Key Insight : K₂HPO₄’s higher alkalinity makes it suitable for neutralizing acidic systems, whereas KH₂PO₄ is preferred in acidic environments.
Comparison with Disodium Salts
Structural and Functional Differences
compares this compound and disodium salts of the dianion dbcot²⁻. Key findings include:
- Ionic Radius Effects : Potassium ions (K⁺, 1.38 Å) are larger than sodium ions (Na⁺, 0.95 Å), leading to distinct crystal lattice structures and stability profiles.
- Magnetic and Aromatic Properties : this compound salts exhibit weaker anti-aromaticity than disodium salts due to cation-anion interactions, as shown by Nuclear Independent Chemical Shift (NICS) calculations.
Rhodizonic Acid this compound Salt vs. Sodium Analogs
- Rhodizonic Acid this compound Salt (CAS 13021-40-4) : Priced at ~US$2,200 per ton (2024), it is used in dye synthesis and metal detection.
- Sodium Rhodizonate : Less thermally stable but cheaper, limiting its use in high-temperature applications.
This compound Glycyrrhizinate in Pharmaceuticals
This compound dominates the anti-inflammatory market due to higher bioavailability compared to sodium glycyrrhizinate, with a projected CAGR of 5.8% (2024–2030).
Biological Activity
Dipotassium compounds, particularly This compound Glycyrrhizinate (DPG) , have garnered attention in recent years due to their diverse biological activities, including anti-inflammatory, antioxidant, and potential anticancer properties. This article explores the biological activity of DPG, supported by case studies and research findings.
Overview of this compound Glycyrrhizinate (DPG)
This compound Glycyrrhizinate is a salt derived from glycyrrhizic acid, primarily found in licorice root. It is known for its therapeutic benefits, particularly in dermatological applications and inflammation management. The compound has been studied for its effects on various biological processes, including wound healing and cancer cell proliferation.
Anti-inflammatory Effects
Research indicates that DPG possesses significant anti-inflammatory properties. A study demonstrated that DPG treatment led to a notable reduction in inflammatory markers in rat models. Specifically, it decreased the expression of pro-inflammatory cytokines such as TNF-α, IL-1β, and IL-6 while increasing anti-inflammatory markers like IL-10. This modulation of cytokine expression suggests that DPG can effectively mitigate inflammation during wound healing processes .
Table 1: Effects of DPG on Inflammatory Markers
Cytokine | Control Group | DPG Treatment (Day 3) | DPG Treatment (Day 7) |
---|---|---|---|
TNF-α | High | Reduced | Further reduced |
IL-1β | High | Reduced | Further reduced |
IL-6 | High | Reduced | Further reduced |
IL-10 | Low | Increased | Significantly increased |
Wound Healing Properties
DPG has been shown to enhance skin wound healing. In a controlled study, animals treated with DPG exhibited faster wound closure and reduced inflammation compared to control groups. The absence of active hyperemia by day 14 in the DPG-treated group indicates its potential to facilitate tissue repair by reducing vascular permeability and leukocyte infiltration .
Anticancer Activity
Recent studies have also explored the potential anticancer effects of DPG. Research involving glioblastoma cell lines (U87MG and T98G) revealed that DPG significantly inhibited cell proliferation and induced apoptosis through the suppression of the NF-κB signaling pathway. The compound was found to enhance the efficacy of chemotherapeutic agents like temozolomide (TMZ), suggesting its role as a promising adjunct in cancer therapy .
Table 2: Effects of DPG on Glioblastoma Cell Lines
Cell Line | Control Viability (%) | DPG Treatment Viability (%) | Apoptosis Rate (%) |
---|---|---|---|
U87MG | 100 | 45 | 30 |
T98G | 100 | 40 | 35 |
The mechanisms underlying the biological activities of DPG are multifaceted:
- Cytokine Modulation : DPG inhibits the release of HMGB1, a protein involved in inflammation, thereby reducing the levels of pro-inflammatory cytokines .
- NF-κB Pathway Suppression : By affecting NF-κB signaling, DPG decreases cell viability in cancer cells and enhances apoptosis .
- Collagen Production : DPG promotes collagen synthesis, which is crucial for tissue remodeling during wound healing .
Case Studies
Several case studies have highlighted the effectiveness of DPG in clinical settings:
- A study involving patients with atopic dermatitis showed that topical application of DPG resulted in significant improvements in skin condition by reducing Staphylococcus aureus colonization and associated inflammation .
- In another case, patients undergoing treatment for inflammatory bowel disease experienced symptom relief when administered DPG, correlating with decreased inflammatory markers in stool samples .
Q & A
Basic Research Questions
Q. What are the standard methodologies for synthesizing dipotassium compounds with high purity, and how can researchers validate their synthesis protocols?
- Methodological Guidance :
- Use controlled precipitation or crystallization techniques under inert atmospheres to minimize contamination .
- Validate purity via X-ray diffraction (XRD) for crystallinity, inductively coupled plasma mass spectrometry (ICP-MS) for elemental composition, and nuclear magnetic resonance (NMR) for structural confirmation .
- Include negative controls (e.g., unreacted precursors) to isolate synthesis artifacts .
Q. How do temperature and storage conditions influence the stability of this compound salts in aqueous solutions?
- Methodological Guidance :
- Design stability studies with systematic variables: pH (4–10), temperature (4°C–40°C), and ionic strength (0.1–1.0 M). Monitor degradation via high-performance liquid chromatography (HPLC) and ion chromatography .
- Use Arrhenius plots to predict shelf life under accelerated conditions (e.g., 40°C/75% RH) .
Q. What spectroscopic techniques are most effective for characterizing this compound complexes in biological matrices?
- Methodological Guidance :
- Employ Fourier-transform infrared spectroscopy (FTIR) for ligand-binding analysis and UV-Vis spectroscopy for electronic transitions. For trace detection in tissues, pair ICP-MS with laser ablation sampling .
Advanced Research Questions
Q. How can researchers resolve contradictions in reported dissociation constants (pKa) of this compound phosphate buffers across studies?
- Methodological Guidance :
- Conduct a systematic review using PRISMA guidelines to identify methodological discrepancies (e.g., ionic strength adjustments, temperature control) .
- Replicate experiments under standardized conditions (e.g., 25°C, 0.15 M NaCl) and validate with potentiometric titrations .
- Apply meta-analysis to quantify heterogeneity sources (e.g., random-effects models) .
Q. What mechanistic insights explain the role of this compound ions in modulating enzyme kinetics, and how can these be experimentally verified?
- Methodological Guidance :
- Use stopped-flow spectroscopy to measure real-time enzyme activity under varying this compound concentrations. Apply Michaelis-Menten and Lineweaver-Burk plots to distinguish competitive vs. non-competitive inhibition .
- Mutagenesis studies (e.g., K⁺-binding site mutations) can isolate ion-specific effects .
Q. How can computational models predict the solvation dynamics of this compound salts in non-aqueous solvents, and what experimental data are needed for validation?
- Methodological Guidance :
- Use molecular dynamics (MD) simulations with force fields (e.g., AMBER) to model ion-pair interactions. Validate with neutron scattering data and dielectric relaxation spectroscopy .
- Compare simulated radial distribution functions (RDFs) with experimental X-ray absorption fine structure (EXAFS) results .
Q. Data Analysis & Contradiction Resolution
Q. What statistical approaches are recommended for reconciling conflicting bioavailability data of this compound supplements in human trials?
- Methodological Guidance :
- Perform subgroup analysis by demographic factors (age, renal function) and dose regimens. Use multivariate regression to adjust for covariates (e.g., dietary K⁺ intake) .
- Apply sensitivity analysis to assess robustness against outliers and measurement biases .
Q. How should researchers design experiments to distinguish between synergistic and antagonistic effects of this compound and magnesium ions in cellular uptake studies?
- Methodological Guidance :
- Use factorial design (2×2 matrix: ±K₂⁺, ±Mg²⁺) with fluorescence-based ion flux assays (e.g., Fluo-4 AM dye). Analyze interactions via two-way ANOVA and Tukey’s post hoc tests .
Q. Literature & Framework Integration
Q. What frameworks (e.g., PICO, FINER) are optimal for structuring hypothesis-driven studies on this compound’s role in metabolic pathways?
- Methodological Guidance :
- Apply PICO : Population (e.g., hepatocytes), Intervention (this compound exposure), Comparison (Na⁺ or Li⁺ controls), Outcome (ATPase activity) .
- Evaluate feasibility via FINER : Ensure access to knockout models, ethical approval for animal studies, and relevance to potassium homeostasis disorders .
Q. How can researchers critically assess the quality of existing literature on this compound’s environmental bioavailability?
- Methodological Guidance :
- Use Cochrane Risk of Bias Tool for experimental studies and GRADE for observational data. Prioritize studies with controlled confounding variables (e.g., soil pH, organic matter) .
Q. Experimental Design Pitfalls
Q. What are common pitfalls in designing longitudinal studies on this compound’s nephrotoxic effects, and how can they be mitigated?
Properties
CAS No. |
19046-60-7 |
---|---|
Molecular Formula |
C6H11K2O9P |
Molecular Weight |
336.32 g/mol |
IUPAC Name |
dipotassium;[(2R,3R,4S,5R,6R)-3,4,5-trihydroxy-6-(hydroxymethyl)oxan-2-yl] phosphate |
InChI |
InChI=1S/C6H13O9P.2K/c7-1-2-3(8)4(9)5(10)6(14-2)15-16(11,12)13;;/h2-10H,1H2,(H2,11,12,13);;/q;2*+1/p-2/t2-,3+,4+,5-,6-;;/m1../s1 |
InChI Key |
KCIDZIIHRGYJAE-YGFYJFDDSA-L |
SMILES |
C(C1C(C(C(C(O1)OP(=O)([O-])[O-])O)O)O)O.[K+].[K+] |
Isomeric SMILES |
C([C@@H]1[C@@H]([C@@H]([C@H]([C@H](O1)OP(=O)([O-])[O-])O)O)O)O.[K+].[K+] |
Canonical SMILES |
C(C1C(C(C(C(O1)OP(=O)([O-])[O-])O)O)O)O.[K+].[K+] |
Appearance |
Assay:≥95%A crystalline solid |
Key on ui other cas no. |
19046-60-7 |
Synonyms |
α-D-Galactopyranose 1-(Dihydrogen Phosphate) Dipotassium Salt; α-D-Galactopyranose-1 1-Phosphate Dipotassium Salt; D-Galactose 1-Phosphate Dipotassium Salt; α-D-Galactopyranosyl Phosphate Dipotassium Salt; α-D-Galactose 1-Phosphate Dipotassium Salt; |
Origin of Product |
United States |
Retrosynthesis Analysis
AI-Powered Synthesis Planning: Our tool employs the Template_relevance Pistachio, Template_relevance Bkms_metabolic, Template_relevance Pistachio_ringbreaker, Template_relevance Reaxys, Template_relevance Reaxys_biocatalysis model, leveraging a vast database of chemical reactions to predict feasible synthetic routes.
One-Step Synthesis Focus: Specifically designed for one-step synthesis, it provides concise and direct routes for your target compounds, streamlining the synthesis process.
Accurate Predictions: Utilizing the extensive PISTACHIO, BKMS_METABOLIC, PISTACHIO_RINGBREAKER, REAXYS, REAXYS_BIOCATALYSIS database, our tool offers high-accuracy predictions, reflecting the latest in chemical research and data.
Strategy Settings
Precursor scoring | Relevance Heuristic |
---|---|
Min. plausibility | 0.01 |
Model | Template_relevance |
Template Set | Pistachio/Bkms_metabolic/Pistachio_ringbreaker/Reaxys/Reaxys_biocatalysis |
Top-N result to add to graph | 6 |
Feasible Synthetic Routes
Disclaimer and Information on In-Vitro Research Products
Please be aware that all articles and product information presented on BenchChem are intended solely for informational purposes. The products available for purchase on BenchChem are specifically designed for in-vitro studies, which are conducted outside of living organisms. In-vitro studies, derived from the Latin term "in glass," involve experiments performed in controlled laboratory settings using cells or tissues. It is important to note that these products are not categorized as medicines or drugs, and they have not received approval from the FDA for the prevention, treatment, or cure of any medical condition, ailment, or disease. We must emphasize that any form of bodily introduction of these products into humans or animals is strictly prohibited by law. It is essential to adhere to these guidelines to ensure compliance with legal and ethical standards in research and experimentation.