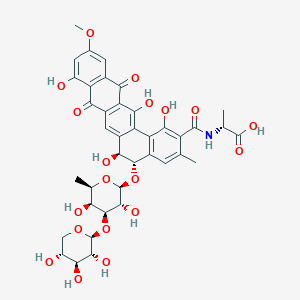
Benanomicin A
Overview
Description
Benanomicin A is a benzo[a]naphthacenequinone antibiotic first isolated from Actinomadura species . It consists of a polyketide-derived aglycon core decorated with a disaccharide moiety (D-olivose and D-amicetose) and a unique alanine-containing side chain . Its antifungal activity is attributed to calcium-dependent binding to D-mannoside residues in fungal cell wall mannoproteins, disrupting membrane integrity . This compound exhibits broad-spectrum activity against Candida spp., Aspergillus spp., and Pneumocystis carinii , with low mammalian toxicity and high water solubility .
Preparation Methods
Retrosynthetic Strategy for Benanomicin A
The synthesis of this compound’s tetracyclic core relies on a retrosynthetic approach that dissects the molecule into simpler precursors. As outlined in Scheme 1 of Hirosawa et al. (1997), the strategy involves constructing the 5,6-dihydrobenzo[a]naphthacenequinone skeleton through a Diels-Alder reaction between an outer-ring diene (A-B ring system) and a naphthoquinone (D-E ring) . This method emphasizes regioselectivity to ensure proper alignment of reactive sites, a critical factor given the molecule’s polycyclic architecture.
Key to this approach is the use of α-tetralone (2) as the starting material for the A-B ring system. α-Tetralone undergoes sequential modifications to form a diene capable of participating in the Diels-Alder reaction. The naphthoquinone component, derived from simpler aromatic precursors, introduces the quinone functionality essential for this compound’s bioactivity .
Synthesis of the A-B Ring System
Formation of the Keto-Amine Intermediate
The synthesis begins with α-tetralone (2), which is treated with paraformaldehyde and dimethylamine hydrochloride in ethanol under reflux conditions. This reaction yields the keto-amine intermediate (3) with an 86% efficiency . The dimethylamine group serves as a directing agent for subsequent olefination steps, while the ketone moiety primes the molecule for Wittig reactions.
Wittig Olefination to Generate Enol Methyl Ethers
The keto-amine (3) undergoes Wittig olefination using (methoxymethylene)phosphorane, generated in situ from phenyllithium and (methoxymethyl)triphenylphosphonium chloride. Conducted at –45°C in tetrahydrofuran (THF), this step produces a 6:1 mixture of enol methyl ether isomers (4) in 72% yield . The major isomer, characterized by a proton signal at δ 6.57 in its *¹H NMR spectrum, dominates the product distribution. Separation of the isomers proves challenging due to their structural similarity, necessitating careful chromatographic purification.
Diels-Alder Reaction with Naphthoquinone
The enol methyl ether (4) participates in a regioselective Diels-Alder reaction with naphthoquinone to form the 5,6-dihydrobenzo[a]naphthacenequinone skeleton (8). This step proceeds with 44% yield, achieving the desired regiochemistry without detectable side products . The reaction’s success hinges on the electron-rich diene and the electron-deficient quinone, which align to favor the endo transition state.
Table 1: Key Reaction Steps and Yields
Step | Reaction | Conditions | Yield |
---|---|---|---|
1 | Keto-amine formation | EtOH, reflux | 86% |
2 | Wittig olefination | THF, –45°C to 0°C | 72% |
3 | Diels-Alder reaction | Naphthoquinone, regioselective | 44% |
Alternative Methods and Derivatives
Desalaninethis compound as a Synthetic Intermediate
Ikeda et al. (1992) describe the cleavage of this compound’s amido bond using Meerwein’s reagent (trimethyloxonium tetrafluoroborate) to yield desalaninethis compound (5) . While this process is primarily used to generate amino acid analogs, it underscores the importance of protecting group strategies in handling this compound’s labile functional groups. The methyl ester of desalaninethis compound is obtained in 44% yield after hydrolysis and reflux in aqueous acetone .
Patent Literature and Structural Confirmation
A 1998 European Patent Office decision (T 0853/94) confirms the structural identity of this compound and its derivatives, citing prior art that validates the synthetic routes described in journal publications . Although the patent focuses on therapeutic applications, it indirectly supports the reproducibility of the preparation methods by referencing foundational synthetic studies .
Challenges and Optimization
Regioselectivity and Isomer Separation
The Wittig olefination step produces a mixture of enol methyl ether isomers, complicating purification . Advanced spectroscopic techniques (e.g., *¹H NMR) are required to monitor isomer ratios, and iterative crystallization may improve separation.
Yield Improvements
The Diels-Alder reaction’s moderate yield (44%) highlights opportunities for optimization. Catalyst screening (e.g., Lewis acids) and solvent engineering could enhance reaction efficiency while maintaining regioselectivity.
Chemical Reactions Analysis
Types of Reactions: Benanomicin A undergoes various chemical reactions, including:
Reduction: Involves the reduction of the quinone moiety to hydroquinone.
Substitution: Involves the substitution of functional groups on the benzo(a)naphthacenequinone skeleton.
Common Reagents and Conditions:
Oxidation: Cytochrome P450 enzymes, molecular oxygen, and cofactors such as NADPH.
Reduction: Reducing agents such as sodium borohydride or lithium aluminum hydride.
Substitution: Various nucleophiles and electrophiles under appropriate conditions.
Major Products:
Oxidation: 10-hydroxythis compound and 11-O-demethylthis compound.
Reduction: Hydroquinone derivatives.
Substitution: Various substituted benanomicin derivatives.
Scientific Research Applications
Benanomicin A has a wide range of scientific research applications, including:
Chemistry: Used as a model compound for studying the synthesis and reactivity of benzo(a)naphthacenequinone derivatives.
Biology: Investigated for its antifungal and antiviral activities.
Medicine: Potential therapeutic agent for treating fungal infections and certain viral infections.
Industry: Used in the development of new antifungal agents and as a lead compound for drug discovery.
Mechanism of Action
The mechanism of action of Benanomicin A involves binding to the fungal cell wall, leading to disruption of the cell permeability barrier and leakage of intracellular components such as potassium ions and adenosine triphosphate . This binding is suggested to deteriorate the normal structure and function of fungal cell membranes, particularly in metabolically active cells . The exact molecular targets and pathways involved are not fully confirmed but are believed to include mannoproteins constituting the cell wall and cell membrane .
Comparison with Similar Compounds
Structural Analogues of Benanomicin A
Benanomicin B
- Structure: Shares the same aglycon as this compound but differs in glycon components (sugar moieties) .
- Activity: Less potent than this compound due to altered glycosylation, which affects binding affinity to fungal mannans .
- Biosynthesis : Produced via a type II polyketide synthase pathway with post-polyketide modifications (e.g., glycosylation, methylation) .
10-Hydroxythis compound and 11-O-Demethylthis compound
- Structure: Generated via biotransformation using E. coli expressing actinomycete cytochrome P450 (CYP105C1). 10-Hydroxythis compound: Oxygenation at C-10 . 11-O-Demethylthis compound: Loss of the methoxy group at C-11 .
- Activity: Both analogues show reduced antifungal activity compared to this compound. 11-O-Demethylthis compound retains weak activity against Candida pseudotropicalis, while 10-hydroxythis compound is nearly inactive .
Table 1: Antifungal Activity of this compound and Analogues
Compound | MIC (μg/mL) Against Key Pathogens | Key Structural Features |
---|---|---|
This compound | 0.05–0.5 (Candida spp., Aspergillus) | Intact C-10 H, C-11 OCH₃ |
11-O-Demethylthis compound | 5–20 (C. pseudotropicalis) | C-11 demethylation |
10-Hydroxythis compound | >50 (most fungi) | C-10 hydroxylation |
Benanomicin B | 1–10 (Candida spp.) | Modified glycon |
Pradimicin Family
Pradimicins (e.g., pradimicin A, BMY-28864) share the same benzo[a]naphthacenequinone core and mannose-binding mechanism as this compound but differ in side-chain modifications .
- Key Differences: Pradimicins lack the alanine-containing side chain of this compound. They exhibit stronger fungicidal effects in vivo (e.g., against pulmonary candidiasis) but comparable in vitro potency .
- Biosynthetic Relationship : Both families originate from similar polyketide pathways but diverge in post-PKS modifications, such as methylation and glycosylation .
Madurahydroxylactone (MHL) Derivatives
- Structure: MHL derivatives belong to the benzo[a]naphthacenequinone family but target viral enzymes (HIV-1 integrase and RNase H) rather than fungal cells .
Structure-Activity Relationship (SAR) Insights
- Critical Functional Groups: C-10 Hydrogen and C-11 Methoxy: Essential for antifungal potency; demethylation or hydroxylation at these positions reduces activity . Glycon Moieties: Influence solubility and binding specificity; modifications in Benanomicin B lower efficacy .
- Calcium-Dependent Mechanism : Shared with pradimicins, but structural variations alter binding kinetics and spectrum .
Biological Activity
Benanomicin A is a potent antifungal compound isolated from the actinomycete Actinomadura spadix. Its unique structure, characterized by a benzo[a]naphthacenequinone skeleton, contributes to its broad spectrum of biological activities, particularly against various fungal pathogens. This article explores the biological activity of this compound, including its antifungal and antiviral properties, mechanisms of action, and recent research findings.
Antifungal Activity
This compound exhibits significant antifungal activity against a range of fungi, including clinically relevant pathogens such as Candida albicans, Aspergillus fumigatus, and Cryptococcus neoformans . The compound's effectiveness has been demonstrated in both in vitro and in vivo models. The following table summarizes the antifungal activity of this compound against various fungal species:
Fungal Species | Minimum Inhibitory Concentration (MIC) | Activity Level |
---|---|---|
Candida albicans | 0.5 µg/mL | Potent |
Aspergillus fumigatus | 2.0 µg/mL | Moderate |
Cryptococcus neoformans | 1.0 µg/mL | Potent |
Trichophyton rubrum | 4.0 µg/mL | Moderate |
Cochliobolus lunatus | 8.0 µg/mL | Weak |
The mechanism by which this compound exerts its antifungal effects involves binding to the mannan component of fungal cell walls in the presence of calcium ions (Ca²⁺). This binding is crucial for its antifungal activity, as it disrupts the integrity of the fungal cell wall, leading to cell lysis .
Research indicates that this compound interacts specifically with D-mannoside residues on the surface of fungal cells, similar to lectins . The presence of a carboxylic acid in its D-alanine moiety and a sugar moiety are essential for both binding and antifungal activities .
Antiviral Activity
In addition to its antifungal properties, this compound has shown antiviral activity against certain viruses. Studies suggest that it may inhibit viral replication through mechanisms similar to its antifungal action, although further research is needed to elucidate these pathways completely .
Case Studies and Research Findings
Recent studies have focused on enhancing the efficacy of this compound through biotransformation and the development of analogs. For example, derivatives such as 10-hydroxythis compound and 11-O-demethylthis compound were generated using engineered Escherichia coli expressing cytochrome P450 enzymes. However, these derivatives exhibited weaker antifungal activities compared to this compound itself .
Another study highlighted a novel method for purifying cell wall mannan from Candida krusei using this compound. This method preserved more O-linked sugar chains compared to traditional methods, indicating potential applications in improving the extraction of complex carbohydrates from fungal cells .
Q & A
Basic Research Questions
Q. What experimental methodologies are recommended for assessing the antifungal activity of Benanomicin A against pathogenic fungi?
- Methodology : Use standardized broth microdilution assays to determine minimum inhibitory concentrations (MICs) against fungal strains like C. albicans and A. niger. Include positive controls (e.g., amphotericin B) and validate results using colony-forming unit (CFU) counts. Ensure media pH and incubation conditions align with fungal growth requirements .
- Data Interpretation : MIC values ≤50 µg/ml indicate significant antifungal activity. Compare results with literature data to confirm consistency in potency across strains .
Q. How can researchers validate this compound’s anti-HIV activity in vitro?
- Protocol : Employ MT-4 cell lines infected with HIV-1 and measure viral inhibition using p24 antigen ELISA or reverse transcriptase activity assays. Include concentration-dependent treatments (e.g., 0.1–100 µM) and cytotoxicity controls (e.g., MTT assays) to ensure specificity .
- Statistical Analysis : Calculate IC50 values and compare with established antiviral agents (e.g., zidovudine) to benchmark efficacy .
Advanced Research Questions
Q. How do structural variations in this compound’s oligomannosyl side chains influence its bioactivity, and what analytical techniques resolve these differences?
- Experimental Design : Isolate this compound from Actinomycetes and purify mannan components using this compound-assisted mild extraction. Analyze side-chain distribution via <sup>1</sup>H/<sup>13</sup>C NMR and compare with Fehling reagent-based acetylated derivatives to detect cleavage artifacts .
- Contradiction Management : If NMR detects pentasaccharides/tetrasaccharides but not trisaccharides, consider whether harsh extraction methods (e.g., Fehling) artificially degrade labile β-1,2-mannose linkages, skewing results .
Q. What strategies resolve discrepancies in this compound’s reported antiviral mechanisms across studies?
- Hypothesis Testing : Use siRNA knockdown or CRISPR-Cas9 to target host factors (e.g., CD4 receptors) in HIV-infected cells. Compare this compound’s inhibitory effects in wild-type vs. modified cells to distinguish direct viral targeting from host-mediated mechanisms .
- Data Integration : Cross-reference findings with proteomic or transcriptomic datasets to identify pathways consistently modulated by this compound .
Q. How can researchers optimize this compound’s biosynthetic yield in Actinomycetes cultures?
- Methodology : Screen cytochrome P450 (CYP) gene clusters (e.g., CYP105C1) linked to this compound production using genomic mining. Overexpress regulatory genes (e.g., bldD) in Streptomyces strains to enhance secondary metabolite synthesis .
- Validation : Quantify yield via HPLC and correlate with transcriptional activation of biosynthetic genes under varied nutrient conditions (e.g., carbon/nitrogen ratios) .
Q. Experimental Design & Reproducibility
Q. What steps ensure reproducibility in this compound’s bioactivity assays across laboratories?
- Standardization : Adopt CLSI guidelines for antifungal testing, including strain sourcing (e.g., ATCC strains), media preparation, and endpoint criteria (e.g., 80% growth inhibition). Share raw data (e.g., absorbance readings) in supplementary materials .
- Inter-lab Validation : Participate in ring trials with independent labs to calibrate assay conditions and harmonize MIC interpretations .
Q. How should researchers address conflicting reports on this compound’s cytotoxicity in mammalian cells?
- Troubleshooting : Replicate studies using identical cell lines (e.g., HEK293 vs. MT-4) and culture conditions. Perform time-course assays to differentiate acute vs. chronic toxicity. Publish full cytotoxicity curves (IC50, LD50) alongside activity data .
Q. Structural & Mechanistic Insights
Q. What computational tools predict this compound’s binding interactions with fungal cell wall components?
- Approach : Use molecular docking (e.g., AutoDock Vina) to model interactions between this compound and β-1,3-glucan synthase. Validate predictions with surface plasmon resonance (SPR) to measure binding kinetics .
- Limitations : Address discrepancies between in silico and experimental data by refining force field parameters or incorporating membrane lipid effects .
Q. Ethical & Literature Review Practices
Q. How can researchers avoid redundancy in studying this compound’s known properties while exploring novel applications?
- Scoping Review : Conduct a systematic review using PRISMA guidelines to map existing studies on antifungal/antiviral mechanisms. Prioritize understudied areas (e.g., immunomodulatory effects) using FINER criteria (Feasible, Novel, Ethical) .
- Patent Analysis : Review granted patents (e.g., EP0886689B1) to identify unexplored claims, such as combinatorial therapies with antiretrovirals .
Properties
IUPAC Name |
(2R)-2-[[(5S,6S)-5-[(2S,3R,4S,5S,6R)-3,5-dihydroxy-6-methyl-4-[(2S,3R,4S,5R)-3,4,5-trihydroxyoxan-2-yl]oxyoxan-2-yl]oxy-1,6,9,14-tetrahydroxy-11-methoxy-3-methyl-8,13-dioxo-5,6-dihydrobenzo[a]tetracene-2-carbonyl]amino]propanoic acid | |
---|---|---|
Source | PubChem | |
URL | https://pubchem.ncbi.nlm.nih.gov | |
Description | Data deposited in or computed by PubChem | |
InChI |
InChI=1S/C39H41NO19/c1-10-5-17-23(30(48)20(10)36(52)40-11(2)37(53)54)22-15(8-16-24(31(22)49)27(45)14-6-13(55-4)7-18(41)21(14)26(16)44)28(46)34(17)58-39-33(51)35(25(43)12(3)57-39)59-38-32(50)29(47)19(42)9-56-38/h5-8,11-12,19,25,28-29,32-35,38-39,41-43,46-51H,9H2,1-4H3,(H,40,52)(H,53,54)/t11-,12-,19-,25+,28+,29+,32-,33-,34+,35+,38+,39+/m1/s1 | |
Source | PubChem | |
URL | https://pubchem.ncbi.nlm.nih.gov | |
Description | Data deposited in or computed by PubChem | |
InChI Key |
GOYUMGXIFMGKFN-NUVDETJMSA-N | |
Source | PubChem | |
URL | https://pubchem.ncbi.nlm.nih.gov | |
Description | Data deposited in or computed by PubChem | |
Canonical SMILES |
CC1C(C(C(C(O1)OC2C(C3=CC4=C(C(=C3C5=C2C=C(C(=C5O)C(=O)NC(C)C(=O)O)C)O)C(=O)C6=C(C4=O)C(=CC(=C6)OC)O)O)O)OC7C(C(C(CO7)O)O)O)O | |
Source | PubChem | |
URL | https://pubchem.ncbi.nlm.nih.gov | |
Description | Data deposited in or computed by PubChem | |
Isomeric SMILES |
C[C@@H]1[C@@H]([C@@H]([C@H]([C@@H](O1)O[C@@H]2[C@H](C3=CC4=C(C(=C3C5=C2C=C(C(=C5O)C(=O)N[C@H](C)C(=O)O)C)O)C(=O)C6=C(C4=O)C(=CC(=C6)OC)O)O)O)O[C@H]7[C@@H]([C@H]([C@@H](CO7)O)O)O)O | |
Source | PubChem | |
URL | https://pubchem.ncbi.nlm.nih.gov | |
Description | Data deposited in or computed by PubChem | |
Molecular Formula |
C39H41NO19 | |
Source | PubChem | |
URL | https://pubchem.ncbi.nlm.nih.gov | |
Description | Data deposited in or computed by PubChem | |
DSSTOX Substance ID |
DTXSID801317567 | |
Record name | Benanomicin A | |
Source | EPA DSSTox | |
URL | https://comptox.epa.gov/dashboard/DTXSID801317567 | |
Description | DSSTox provides a high quality public chemistry resource for supporting improved predictive toxicology. | |
Molecular Weight |
827.7 g/mol | |
Source | PubChem | |
URL | https://pubchem.ncbi.nlm.nih.gov | |
Description | Data deposited in or computed by PubChem | |
CAS No. |
116249-65-1 | |
Record name | Benanomicin A | |
Source | CAS Common Chemistry | |
URL | https://commonchemistry.cas.org/detail?cas_rn=116249-65-1 | |
Description | CAS Common Chemistry is an open community resource for accessing chemical information. Nearly 500,000 chemical substances from CAS REGISTRY cover areas of community interest, including common and frequently regulated chemicals, and those relevant to high school and undergraduate chemistry classes. This chemical information, curated by our expert scientists, is provided in alignment with our mission as a division of the American Chemical Society. | |
Explanation | The data from CAS Common Chemistry is provided under a CC-BY-NC 4.0 license, unless otherwise stated. | |
Record name | Benanomicin A | |
Source | ChemIDplus | |
URL | https://pubchem.ncbi.nlm.nih.gov/substance/?source=chemidplus&sourceid=0116249651 | |
Description | ChemIDplus is a free, web search system that provides access to the structure and nomenclature authority files used for the identification of chemical substances cited in National Library of Medicine (NLM) databases, including the TOXNET system. | |
Record name | Benanomicin A | |
Source | EPA DSSTox | |
URL | https://comptox.epa.gov/dashboard/DTXSID801317567 | |
Description | DSSTox provides a high quality public chemistry resource for supporting improved predictive toxicology. | |
Retrosynthesis Analysis
AI-Powered Synthesis Planning: Our tool employs the Template_relevance Pistachio, Template_relevance Bkms_metabolic, Template_relevance Pistachio_ringbreaker, Template_relevance Reaxys, Template_relevance Reaxys_biocatalysis model, leveraging a vast database of chemical reactions to predict feasible synthetic routes.
One-Step Synthesis Focus: Specifically designed for one-step synthesis, it provides concise and direct routes for your target compounds, streamlining the synthesis process.
Accurate Predictions: Utilizing the extensive PISTACHIO, BKMS_METABOLIC, PISTACHIO_RINGBREAKER, REAXYS, REAXYS_BIOCATALYSIS database, our tool offers high-accuracy predictions, reflecting the latest in chemical research and data.
Strategy Settings
Precursor scoring | Relevance Heuristic |
---|---|
Min. plausibility | 0.01 |
Model | Template_relevance |
Template Set | Pistachio/Bkms_metabolic/Pistachio_ringbreaker/Reaxys/Reaxys_biocatalysis |
Top-N result to add to graph | 6 |
Feasible Synthetic Routes
Disclaimer and Information on In-Vitro Research Products
Please be aware that all articles and product information presented on BenchChem are intended solely for informational purposes. The products available for purchase on BenchChem are specifically designed for in-vitro studies, which are conducted outside of living organisms. In-vitro studies, derived from the Latin term "in glass," involve experiments performed in controlled laboratory settings using cells or tissues. It is important to note that these products are not categorized as medicines or drugs, and they have not received approval from the FDA for the prevention, treatment, or cure of any medical condition, ailment, or disease. We must emphasize that any form of bodily introduction of these products into humans or animals is strictly prohibited by law. It is essential to adhere to these guidelines to ensure compliance with legal and ethical standards in research and experimentation.