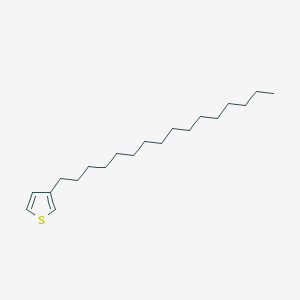
3-Hexadecylthiophene
Overview
Description
Synthesis Analysis
The synthesis of 3-Hexadecylthiophene-based polymers, such as poly(3-hexylthiophene) (P3HT), involves various methodologies, including one-pot synthesis techniques that utilize Ni(dppp)Cl2 as a catalyst. These methods allow for the controlled synthesis of block copolymers, which can self-assemble into well-defined supramolecular structures and exhibit microphase separation in solid state (Wu et al., 2013).
Molecular Structure Analysis
The molecular structure of regioregular P3HT has been elucidated through electron diffraction analysis of epitaxied thin films, revealing a monoclinic unit cell with specific dimensions and a stacking period indicative of the ordered arrangement of the polythiophene backbones (Kayunkid et al., 2010).
Chemical Reactions and Properties
Chemical reactions involving 3-Hexadecylthiophene derivatives, such as their polymerization and copolymerization, lead to materials with significant electrochemical and optical properties. The synthesis of specific oligo(3-hexylthiophene)s and their reactions have been studied for their electronic spectra and cyclic voltammetry, showing potential for electronic applications (Higuchi et al., 1995).
Physical Properties Analysis
The physical properties of 3-Hexadecylthiophene-based polymers, such as P3HT, include solubility in common organic solvents and regio-regularity confirmed by IR and NMR spectroscopy. These polymers exhibit semi-reversible electrochemical behavior, with distinctive anodic and cathodic peaks, and their optical properties are temperature-dependent, showing polaron formation (Calado et al., 2008).
Chemical Properties Analysis
The chemical properties of 3-Hexadecylthiophene-based polymers are closely related to their structure and synthesis method. The molecular dynamics and temperature-dependent optical absorption of regioregulated P3HT, for example, offer insights into the molecular structure and dynamics, indicating transitions induced by the aliphatic side group motion that affect the intermolecular pi-pi interaction (Yazawa et al., 2010).
Scientific Research Applications
-
Electroluminescent Devices
- Field : Material Science
- Application : 3-Hexadecylthiophene is used in the creation of electroluminescent devices .
- Method : The compound is deposited using Langmuir-Blodgett (LB) techniques to create the electroluminescent devices .
- Results : The specific outcomes of this application are not detailed in the source, but the creation of electroluminescent devices suggests potential use in display technologies .
-
Nonlinear-Optical Susceptibility
- Field : Optics
- Application : 3-Hexadecylthiophene is studied for its effects on the nonlinear-optical susceptibility .
- Method : The compound is used in experiments studying saturation effects in nonlinear-optical susceptibility .
- Results : The specific outcomes and quantitative data for this application are not detailed in the source .
-
Semiconducting Materials
- Field : Material Science
- Application : 3-Hexadecylthiophene is used in the creation of organic semiconducting materials .
- Method : The compound is polymerized and then doped to create the semiconducting materials . Doping involves the introduction of impurities into extremely pure semiconductor material to remove (p-doping) electrons from or add (n-doping) electrons to the p-system, resulting in a change to the electrical properties .
- Results : The electrical conductivity of polythiophenes results from the delocalization of electrons along the conjugated backbone of the polymer, through overlap of p-orbitals .
-
Light-Emitting Devices
- Field : Optics
- Application : 3-Hexadecylthiophene is used in the creation of light-emitting devices .
- Method : The compound is deposited using Langmuir-Blodgett techniques to create the light-emitting devices .
- Results : The specific outcomes of this application are not detailed in the source, but the creation of light-emitting devices suggests potential use in display technologies .
-
Thermoelectric Devices
- Field : Material Science
- Application : 3-Hexadecylthiophene is used in the creation of thermoelectric devices .
- Method : The compound is used in the development of conducting polymers, which are materials with a carbon-based π conjugated backbone. These polymers possess the electrical conductivity of metals and excellent mechanical properties of common plastics .
- Results : The use of 3-Hexadecylthiophene in thermoelectric devices holds great promise for improving energy utilization efficiency and recovery of waste heat energy .
-
Sensing Applications
- Field : Material Science
- Application : 3-Hexadecylthiophene has potential for use in sensing applications .
- Method : The compound is used in the development of Langmuir-Blodgett films .
- Results : The specific outcomes of this application are not detailed in the source, but the use in sensing applications suggests potential use in detection technologies .
Safety And Hazards
properties
IUPAC Name |
3-hexadecylthiophene | |
---|---|---|
Source | PubChem | |
URL | https://pubchem.ncbi.nlm.nih.gov | |
Description | Data deposited in or computed by PubChem | |
InChI |
InChI=1S/C20H36S/c1-2-3-4-5-6-7-8-9-10-11-12-13-14-15-16-20-17-18-21-19-20/h17-19H,2-16H2,1H3 | |
Source | PubChem | |
URL | https://pubchem.ncbi.nlm.nih.gov | |
Description | Data deposited in or computed by PubChem | |
InChI Key |
FRVZSODZVJPMKO-UHFFFAOYSA-N | |
Source | PubChem | |
URL | https://pubchem.ncbi.nlm.nih.gov | |
Description | Data deposited in or computed by PubChem | |
Canonical SMILES |
CCCCCCCCCCCCCCCCC1=CSC=C1 | |
Source | PubChem | |
URL | https://pubchem.ncbi.nlm.nih.gov | |
Description | Data deposited in or computed by PubChem | |
Molecular Formula |
C20H36S | |
Source | PubChem | |
URL | https://pubchem.ncbi.nlm.nih.gov | |
Description | Data deposited in or computed by PubChem | |
Related CAS |
119716-70-0 | |
Record name | Thiophene, 3-hexadecyl-, homopolymer | |
Source | CAS Common Chemistry | |
URL | https://commonchemistry.cas.org/detail?cas_rn=119716-70-0 | |
Description | CAS Common Chemistry is an open community resource for accessing chemical information. Nearly 500,000 chemical substances from CAS REGISTRY cover areas of community interest, including common and frequently regulated chemicals, and those relevant to high school and undergraduate chemistry classes. This chemical information, curated by our expert scientists, is provided in alignment with our mission as a division of the American Chemical Society. | |
Explanation | The data from CAS Common Chemistry is provided under a CC-BY-NC 4.0 license, unless otherwise stated. | |
DSSTOX Substance ID |
DTXSID10377811 | |
Record name | 3-hexadecylthiophene | |
Source | EPA DSSTox | |
URL | https://comptox.epa.gov/dashboard/DTXSID10377811 | |
Description | DSSTox provides a high quality public chemistry resource for supporting improved predictive toxicology. | |
Molecular Weight |
308.6 g/mol | |
Source | PubChem | |
URL | https://pubchem.ncbi.nlm.nih.gov | |
Description | Data deposited in or computed by PubChem | |
Product Name |
3-Hexadecylthiophene | |
CAS RN |
119269-24-8 | |
Record name | 3-hexadecylthiophene | |
Source | EPA DSSTox | |
URL | https://comptox.epa.gov/dashboard/DTXSID10377811 | |
Description | DSSTox provides a high quality public chemistry resource for supporting improved predictive toxicology. | |
Record name | 119269-24-8 | |
Source | European Chemicals Agency (ECHA) | |
URL | https://echa.europa.eu/information-on-chemicals | |
Description | The European Chemicals Agency (ECHA) is an agency of the European Union which is the driving force among regulatory authorities in implementing the EU's groundbreaking chemicals legislation for the benefit of human health and the environment as well as for innovation and competitiveness. | |
Explanation | Use of the information, documents and data from the ECHA website is subject to the terms and conditions of this Legal Notice, and subject to other binding limitations provided for under applicable law, the information, documents and data made available on the ECHA website may be reproduced, distributed and/or used, totally or in part, for non-commercial purposes provided that ECHA is acknowledged as the source: "Source: European Chemicals Agency, http://echa.europa.eu/". Such acknowledgement must be included in each copy of the material. ECHA permits and encourages organisations and individuals to create links to the ECHA website under the following cumulative conditions: Links can only be made to webpages that provide a link to the Legal Notice page. | |
Retrosynthesis Analysis
AI-Powered Synthesis Planning: Our tool employs the Template_relevance Pistachio, Template_relevance Bkms_metabolic, Template_relevance Pistachio_ringbreaker, Template_relevance Reaxys, Template_relevance Reaxys_biocatalysis model, leveraging a vast database of chemical reactions to predict feasible synthetic routes.
One-Step Synthesis Focus: Specifically designed for one-step synthesis, it provides concise and direct routes for your target compounds, streamlining the synthesis process.
Accurate Predictions: Utilizing the extensive PISTACHIO, BKMS_METABOLIC, PISTACHIO_RINGBREAKER, REAXYS, REAXYS_BIOCATALYSIS database, our tool offers high-accuracy predictions, reflecting the latest in chemical research and data.
Strategy Settings
Precursor scoring | Relevance Heuristic |
---|---|
Min. plausibility | 0.01 |
Model | Template_relevance |
Template Set | Pistachio/Bkms_metabolic/Pistachio_ringbreaker/Reaxys/Reaxys_biocatalysis |
Top-N result to add to graph | 6 |
Feasible Synthetic Routes
Citations
Disclaimer and Information on In-Vitro Research Products
Please be aware that all articles and product information presented on BenchChem are intended solely for informational purposes. The products available for purchase on BenchChem are specifically designed for in-vitro studies, which are conducted outside of living organisms. In-vitro studies, derived from the Latin term "in glass," involve experiments performed in controlled laboratory settings using cells or tissues. It is important to note that these products are not categorized as medicines or drugs, and they have not received approval from the FDA for the prevention, treatment, or cure of any medical condition, ailment, or disease. We must emphasize that any form of bodily introduction of these products into humans or animals is strictly prohibited by law. It is essential to adhere to these guidelines to ensure compliance with legal and ethical standards in research and experimentation.