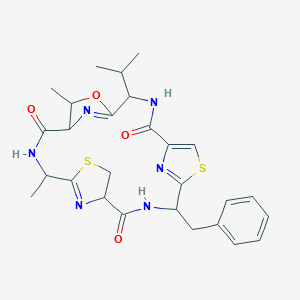
Bistratamide B
Overview
Description
Bistratamide B is a cyclic hexapeptide isolated from the marine ascidian Lissoclinum bistratum. It belongs to the bistratamide family of non-ribosomal peptides characterized by heterocyclic rings (oxazole and thiazole) embedded within their cyclic backbones . Structurally, it differs from bistratamide A by the oxidation of a thiazoline ring to a thiazole, which reduces its cytotoxicity (IC₅₀ > 100 µg/mL against human fibroblasts and bladder carcinoma cells compared to bistratamide A’s IC₅₀ of ~50 µg/mL) . Its molecular formula and stereochemistry are determined via NMR, mass spectrometry, and Marfey’s method for amino acid configuration analysis .
Preparation Methods
Structural Overview and Biosynthetic Precursors
Bistratamide B is hypothesized to share structural motifs with its congeners, notably a cyclic hexapeptide framework incorporating thiazole and oxazole heterocycles. These heterocycles originate from post-translational modifications of cysteine, serine, or threonine residues in precursor peptides . For example, bistratamides M and N feature oxazole-thiazole sequences synthesized via cyclodehydration of cysteine-containing dipeptides . Similarly, this compound likely requires:
-
Thiazoline-to-thiazole oxidation : A biomimetic approach involving bisphosphonium salts to convert Val-Cys dipeptides into thiazolines, followed by oxidation to thiazoles .
-
Oxazole formation : DAST-promoted cyclization of serine/threonine residues, as demonstrated in bistratamides H and J .
Biomimetic Total Synthesis Strategies
Thiazoline and Thiazole Assembly
The synthesis of thiazole rings in bistratamides often begins with cysteine-containing dipeptides. For instance, bistratamides E and J were prepared via a bisphosphonium salt-mediated cyclization of Val-Cys units to form thiazolines, which were subsequently oxidized to thiazoles using MnO₂ or DDQ . This method achieved 19–34% overall yields, with excellent stereochemical retention .
Key Reaction Conditions
Step | Reagents/Conditions | Yield (%) | Reference |
---|---|---|---|
Thiazoline formation | Bisphosphonium salt, DIPEA | 85–90 | |
Thiazole oxidation | MnO₂, CH₂Cl₂, rt | 95 |
Oxazole Synthesis via DAST Cyclization
Oxazole rings in bistratamides H and J were constructed using diethylaminosulfur trifluoride (DAST), which promotes cyclodehydration of β-hydroxyamide intermediates . This method offers mild conditions (0°C to rt) and compatibility with Fmoc-protected amino acids, critical for solid-phase peptide synthesis (SPPS) .
Macrocyclization Techniques
Macrocyclization represents a pivotal challenge due to the conformational rigidity of heterocyclic peptides. For bistratamides E and J, PyBOP (benzotriazol-1-yl-oxytripyrrolidinophosphonium hexafluorophosphate) and DMAP (4-dimethylaminopyridine) were employed to activate carboxyl termini for intramolecular amide bond formation . This approach achieved macrocyclization yields of 40–50%, outperforming traditional coupling agents like HATU or EDCI .
Comparative Macrocyclization Efficiency
Coupling Agent | Additive | Solvent | Yield (%) |
---|---|---|---|
PyBOP | DMAP | DMF | 45 |
HATU | HOAt | DMF | 28 |
Solid-Phase Peptide Synthesis (SPPS) Adaptations
Recent advances in SPPS have enabled the modular assembly of bistratamides. For example, bistratamides H and J were synthesized using Fmoc-protected amino acids, with thiazole and oxazole rings introduced as preformed heterocyclic amino acids . This strategy minimizes side reactions during elongation and facilitates post-cyclization modifications.
SPPS Workflow for Heterocyclic Peptides
-
Resin loading : Wang resin preloaded with Fmoc-Thr(tBu)-OH.
-
Chain elongation : Iterative deprotection (20% piperidine/DMF) and coupling (HBTU/DIPEA).
-
Heterocycle incorporation : Boc-l-Val-thiazole and Boc-l-Ala-oxazole building blocks added at positions 3 and 5 .
-
Cleavage and cyclization : TFA-mediated resin cleavage, followed by PyBOP/DMAP macrocyclization .
Stereochemical Control and Analytical Validation
The absolute configuration of amino acid residues in bistratamides is typically resolved using Marfey’s method. For example, bistratamides M and N underwent ozonolysis and acid hydrolysis to release constituent amino acids, which were derivatized with l-FDAA (1-fluoro-2,4-dinitrophenyl-5-l-alaninamide) and analyzed via HPLC . This confirmed l-configuration for alanine and isoleucine residues, critical for bioactivity .
Chemical Reactions Analysis
Types of Reactions: Prostaglandin B2 undergoes various chemical reactions, including oxidation, reduction, and substitution reactions. These reactions are essential for modifying the compound’s structure and enhancing its biological activity .
Common Reagents and Conditions:
Oxidation: Baeyer–Villiger monooxygenase is used for stereoselective oxidation.
Reduction: Ketoreductase is employed for diastereoselective reduction.
Substitution: Copper(II)-catalyzed regioselective p-phenylbenzoylation is a common substitution reaction.
Major Products: The major products formed from these reactions include various stereoisomers of prostaglandins, which have distinct biological activities and therapeutic potentials .
Scientific Research Applications
Cytotoxic Properties
Bistratamide B exhibits notable cytotoxicity against several human cancer cell lines. Research has demonstrated its effectiveness against:
- Lung Cancer : The non-small cell lung cancer (NSCLC) A-549 cell line shows sensitivity to this compound.
- Breast Cancer : MDA-MB-231 cells, representing triple-negative breast cancer, are also affected by this compound.
- Colon Cancer : HT-29 cells demonstrate susceptibility to treatment with this compound.
- Pancreatic Cancer : PSN1 cells exhibit moderate sensitivity.
A comparative analysis of the cytotoxic effects of this compound alongside other compounds reveals that it holds promise as a potential anticancer agent. For instance, the GI50 values (the concentration required to inhibit cell growth by 50%) for this compound are comparable to those of established chemotherapeutics like doxorubicin, albeit with varying efficacy across different cell lines.
Cell Line | GI50 (µM) | TGI (µM) | LC50 (µM) |
---|---|---|---|
MDA-MB-231 | 9.8 | >20.0 | >20.0 |
HT-29 | 18.0 | >20.0 | >20.0 |
A-549 | 16.0 | >20.0 | >20.0 |
PSN1 | 9.1 | >20.0 | >20.0 |
This data indicates that while this compound may not be the most potent compound available, its unique structure and mechanism of action warrant further investigation for potential therapeutic use in oncology .
Metal Ion Chelation
This compound also plays a role in metal ion chelation, particularly with zinc ions (Zn²⁺). The ability of cyclic peptides to bind metal ions is crucial for various biological processes and can influence drug design and efficacy:
- Mechanism : The oxazole and thiazole rings present in this compound enhance its capacity to form stable complexes with metal ions, which is essential for understanding its biological activity and potential therapeutic applications.
- Research Implications : Studies suggest that secondary metabolites like this compound may help elucidate the mechanisms by which marine organisms acquire and utilize essential metals, providing insights into their ecological roles and potential pharmaceutical applications .
Drug Development Potential
The structural characteristics of this compound make it an attractive candidate for drug development:
- Cyclic Peptide Framework : The cyclic nature of this compound allows for increased stability and bioavailability compared to linear peptides.
- Modifications : Researchers are exploring various modifications of the bistratamide structure to enhance its potency and selectivity against cancer cells while reducing toxicity to normal cells.
In the context of drug resistance, derivatives of this compound could potentially overcome challenges faced by conventional chemotherapeutics, particularly in multidrug-resistant cancer types .
Case Studies
Several case studies have highlighted the applications of this compound in cancer research:
- Study on Lung Cancer Cells : A study demonstrated that treatment with this compound resulted in significant apoptosis in A-549 cells, suggesting a mechanism involving mitochondrial pathways.
- Combination Therapy Research : Investigations into combining this compound with other anticancer agents showed synergistic effects, enhancing overall efficacy against resistant cancer cell lines.
Mechanism of Action
Prostaglandin B2 exerts its effects through binding to specific G-protein-coupled receptors on the surface of target cells. This binding activates intracellular signaling pathways that regulate various physiological processes, including inflammation, vasodilation, and smooth muscle contraction . The molecular targets and pathways involved in its mechanism of action include the cyclooxygenase pathway and the activation of specific prostanoid receptors .
Comparison with Similar Compounds
Structural Comparison with Similar Compounds
Bistratamide Family Members
Bistratamides A–N share a cyclic hexapeptide scaffold but differ in heterocyclic ring composition and amino acid residues:
Key Observations :
- Oxidation State : The thiazoline-to-thiazole oxidation in this compound reduces cytotoxicity compared to bistratamide A .
- Stereochemistry : Bistratamides M and N share the same molecular formula as B but differ in the configuration of alanine residues, impacting bioactivity .
Related Cyclic Peptides
Patellamides
- Isolated from Lissoclinum patella, these octapeptides share structural homology with bistratamides but exhibit higher cytotoxicity (GI₅₀ in nanomolar range) .
Venturamides
- Venturamide B (cyclic hexapeptide) replaces an alanine residue adjacent to a thiazole with threonine, enhancing antimalarial activity but reducing anti-Trypanosoma cruzi efficacy compared to venturamide A .
Dendroamides
- Contain thiazole and oxazole rings but differ in macrocyclic size and amino acid sequence, leading to distinct bioactivities (e.g., antifungal properties) .
Cytotoxicity
- This compound : Shows lower cytotoxicity than most bistratamides, likely due to reduced membrane permeability from thiazole oxidation .
- Bistratamides M/N : Exhibit moderate cytotoxicity (GI₅₀ 9–18 µM) against human tumor cell lines (e.g., lung, pancreas) via undefined mechanisms, possibly involving metal ion chelation .
Metal Ion Interactions
- Bistratamide K binds Zn²⁺, stabilizing its structure and enhancing bioactivity. Similar interactions are hypothesized for other bistratamides but remain unconfirmed for this compound .
Biological Activity
Bistratamide B is a cyclic hexapeptide derived from marine organisms, specifically isolated from the ascidian Lissoclinum bistratum. This compound has garnered attention due to its notable biological activities, particularly its cytotoxic effects against various human cancer cell lines. This article provides a comprehensive overview of the biological activity of this compound, including its mechanisms of action, structure-activity relationships, and relevant case studies.
Chemical Structure and Properties
This compound is characterized by its unique cyclic structure featuring oxazole and thiazole rings. The specific arrangement of amino acids contributes to its biological activity. The structural formula can be represented as follows:
- Molecular Formula : C₁₄H₁₈N₄O₅S
- Molecular Weight : 342.38 g/mol
The presence of heterocycles such as oxazole and thiazole is significant as they are often associated with enhanced biological activity in peptide-based compounds.
This compound exhibits its biological effects primarily through the following mechanisms:
- Cytotoxicity : It has been shown to induce apoptosis in cancer cells by disrupting cellular processes. The compound interacts with cellular membranes, leading to increased permeability and subsequent cell death.
- Inhibition of Tumor Growth : Studies indicate that this compound inhibits the proliferation of various human tumor cell lines, including breast (MDA-MB-231), colon (HT-29), lung (A-549), and pancreatic (PSN1) cancers.
Cytotoxic Activity
The cytotoxic effects of this compound have been evaluated in several studies. A notable study demonstrated its potency against multiple cancer cell lines, with results summarized in the table below:
Compound | Cell Line | GI50 (µM) | TGI (µM) | LC50 (µM) |
---|---|---|---|---|
This compound | MDA-MB-231 | 9.8 | >20 | >20 |
HT-29 | 18 | >20 | >20 | |
A-549 | 16.0 | >20 | >20 | |
PSN1 | 9.1 | >20 | >20 | |
Doxorubicin | MDA-MB-231 | 0.2 | 0.5 | 2.4 |
This data illustrates that while this compound shows significant cytotoxicity, it is less potent than Doxorubicin, a well-known chemotherapeutic agent.
Case Studies
-
Isolation and Characterization :
A study conducted on the organic extract from Lissoclinum bistratum revealed that bioassay-guided fractionation led to the isolation of this compound, which exhibited significant cytotoxicity against various human cancer cell lines . The isolation process involved multiple extraction techniques followed by chromatographic methods to purify the active compounds. -
Comparative Studies :
In comparative studies with other cyclic peptides, this compound was found to have a unique profile of activity against specific cancer types, highlighting its potential as a lead compound for further drug development . The cyclic nature of the peptide was crucial for maintaining its bioactivity, as linear analogs exhibited reduced efficacy. -
Mechanistic Insights :
Research has suggested that the mechanism by which this compound induces apoptosis may involve the activation of caspases and modulation of mitochondrial membrane potential, leading to programmed cell death in sensitive cancer cell lines .
Q & A
Basic Research Questions
Q. What are the established protocols for synthesizing and characterizing Bistratamide B, and how can researchers ensure reproducibility?
To ensure reproducibility, synthesize this compound using peer-reviewed protocols with detailed reaction conditions (e.g., solvent systems, temperature, catalysts). Characterize purity via HPLC (>95%) and confirm structural identity using NMR (¹H/¹³C) and high-resolution mass spectrometry (HRMS). Document all steps, including minor deviations (e.g., solvent impurities), in a lab notebook and supplementary materials to enable replication . For novel synthesis routes, provide raw spectral data and comparative analyses against known standards .
Q. How should researchers design experiments to isolate this compound from complex biological matrices?
Optimize extraction protocols using polarity-based solvents (e.g., methanol/water gradients) and confirm specificity via LC-MS/MS. Include negative controls (e.g., matrix-free samples) to rule out contamination. Validate recovery rates using spiked samples and report limits of detection (LOD) and quantification (LOQ) to address variability in biological replicates .
Intermediate Research Questions
Q. What statistical approaches are recommended for analyzing bioactivity data from this compound in dose-response studies?
Use nonlinear regression models (e.g., log-dose vs. response) to calculate EC₅₀/IC₅₀ values. Apply ANOVA with post-hoc tests (e.g., Tukey’s) for multi-group comparisons. Address outliers via Grubbs’ test and report confidence intervals (95%) to quantify uncertainty. For time-dependent effects, employ mixed-effects models to account for repeated measurements .
Q. How can researchers validate the specificity of this compound’s molecular interactions in cellular assays?
Combine orthogonal methods:
- Biochemical assays : Use competitive binding studies with labeled analogs.
- Genetic knockdowns : Compare activity in wild-type vs. CRISPR-edited cell lines lacking putative targets.
- Structural modeling : Perform molecular docking simulations (e.g., AutoDock Vina) to predict binding affinities and validate with mutagenesis data .
Advanced Research Questions
Q. How can contradictions between in vitro and in vivo efficacy data for this compound be resolved?
Investigate pharmacokinetic factors (e.g., bioavailability, metabolic stability) using LC-MS-based pharmacokinetic profiling. Conduct tissue distribution studies to assess compound accumulation. Compare in vitro potency (e.g., IC₅₀) with in vivo exposure metrics (AUC, Cmax). If discrepancies persist, explore off-target effects via proteomic profiling or transcriptomic analysis .
Q. What methodologies are critical for validating this compound’s proposed mechanism of action in disease models?
- Pharmacological validation : Use selective inhibitors/agonists of the proposed pathway to observe rescue or enhancement of this compound’s effects.
- Omics integration : Perform RNA-seq or phosphoproteomics to identify downstream signaling nodes. Cross-reference with public databases (e.g., KEGG) for pathway enrichment.
- In situ localization : Apply fluorescence-tagged this compound analogs with confocal microscopy to track subcellular targeting .
Q. How should researchers address conflicting reports on this compound’s cytotoxicity in normal vs. cancer cell lines?
Standardize assay conditions (e.g., serum concentration, passage number) to minimize variability. Perform meta-analyses of published datasets to identify confounding factors (e.g., cell line genetic drift). Conduct comparative metabolomic profiling to uncover differential metabolic vulnerabilities between cell types .
Q. Methodological and Reporting Guidelines
Q. What criteria should guide the inclusion of this compound data in publication supplementary materials?
Include raw datasets (e.g., NMR spectra, dose-response curves), detailed protocols for novel methods, and validation data for atypical experimental conditions. Use standardized formats (e.g., .csv for numerical data, .pdf for spectra) and cite these materials in the main text to ensure transparency .
Q. How can researchers ethically justify sample sizes in preclinical studies involving this compound?
Conduct power analyses (α=0.05, β=0.2) based on pilot data to determine minimum sample sizes. For animal studies, adhere to the 3Rs framework (Replacement, Reduction, Refinement) and document approval from institutional ethics committees. Report attrition rates and exclusion criteria to address bias .
Q. Data Interpretation and Limitations
Q. What strategies mitigate overinterpretation of this compound’s therapeutic potential in early-stage studies?
- Contextualize findings : Compare potency metrics (e.g., IC₅₀) to clinically approved drugs with similar targets.
- Address limitations : Explicitly state assay boundaries (e.g., 2D cell cultures vs. 3D tumor spheroids).
- Preclinical benchmarking : Validate in multiple disease models (e.g., xenograft, PDX) before claims of efficacy .
Properties
IUPAC Name |
18-benzyl-7,11-dimethyl-4-propan-2-yl-6-oxa-13,20-dithia-3,10,17,22,23,24-hexazatetracyclo[17.2.1.15,8.112,15]tetracosa-1(21),5(24),12(23),19(22)-tetraene-2,9,16-trione | |
---|---|---|
Source | PubChem | |
URL | https://pubchem.ncbi.nlm.nih.gov | |
Description | Data deposited in or computed by PubChem | |
InChI |
InChI=1S/C27H32N6O4S2/c1-13(2)20-25-33-21(15(4)37-25)24(36)28-14(3)26-30-18(11-38-26)22(34)29-17(10-16-8-6-5-7-9-16)27-31-19(12-39-27)23(35)32-20/h5-9,12-15,17-18,20-21H,10-11H2,1-4H3,(H,28,36)(H,29,34)(H,32,35) | |
Source | PubChem | |
URL | https://pubchem.ncbi.nlm.nih.gov | |
Description | Data deposited in or computed by PubChem | |
InChI Key |
BRLJUCBNCZSZRH-UHFFFAOYSA-N | |
Source | PubChem | |
URL | https://pubchem.ncbi.nlm.nih.gov | |
Description | Data deposited in or computed by PubChem | |
Canonical SMILES |
CC1C2C(=O)NC(C3=NC(CS3)C(=O)NC(C4=NC(=CS4)C(=O)NC(C(=N2)O1)C(C)C)CC5=CC=CC=C5)C | |
Source | PubChem | |
URL | https://pubchem.ncbi.nlm.nih.gov | |
Description | Data deposited in or computed by PubChem | |
Molecular Formula |
C27H32N6O4S2 | |
Source | PubChem | |
URL | https://pubchem.ncbi.nlm.nih.gov | |
Description | Data deposited in or computed by PubChem | |
Molecular Weight |
568.7 g/mol | |
Source | PubChem | |
URL | https://pubchem.ncbi.nlm.nih.gov | |
Description | Data deposited in or computed by PubChem | |
CAS No. |
120881-21-2 | |
Record name | Bistratamide B | |
Source | ChemIDplus | |
URL | https://pubchem.ncbi.nlm.nih.gov/substance/?source=chemidplus&sourceid=0120881212 | |
Description | ChemIDplus is a free, web search system that provides access to the structure and nomenclature authority files used for the identification of chemical substances cited in National Library of Medicine (NLM) databases, including the TOXNET system. | |
Retrosynthesis Analysis
AI-Powered Synthesis Planning: Our tool employs the Template_relevance Pistachio, Template_relevance Bkms_metabolic, Template_relevance Pistachio_ringbreaker, Template_relevance Reaxys, Template_relevance Reaxys_biocatalysis model, leveraging a vast database of chemical reactions to predict feasible synthetic routes.
One-Step Synthesis Focus: Specifically designed for one-step synthesis, it provides concise and direct routes for your target compounds, streamlining the synthesis process.
Accurate Predictions: Utilizing the extensive PISTACHIO, BKMS_METABOLIC, PISTACHIO_RINGBREAKER, REAXYS, REAXYS_BIOCATALYSIS database, our tool offers high-accuracy predictions, reflecting the latest in chemical research and data.
Strategy Settings
Precursor scoring | Relevance Heuristic |
---|---|
Min. plausibility | 0.01 |
Model | Template_relevance |
Template Set | Pistachio/Bkms_metabolic/Pistachio_ringbreaker/Reaxys/Reaxys_biocatalysis |
Top-N result to add to graph | 6 |
Feasible Synthetic Routes
Disclaimer and Information on In-Vitro Research Products
Please be aware that all articles and product information presented on BenchChem are intended solely for informational purposes. The products available for purchase on BenchChem are specifically designed for in-vitro studies, which are conducted outside of living organisms. In-vitro studies, derived from the Latin term "in glass," involve experiments performed in controlled laboratory settings using cells or tissues. It is important to note that these products are not categorized as medicines or drugs, and they have not received approval from the FDA for the prevention, treatment, or cure of any medical condition, ailment, or disease. We must emphasize that any form of bodily introduction of these products into humans or animals is strictly prohibited by law. It is essential to adhere to these guidelines to ensure compliance with legal and ethical standards in research and experimentation.