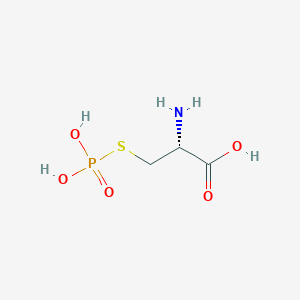
S-Phosphocysteine
Overview
Description
S-Phosphocysteine is a post-translational modification (PTM) where a phosphate group is covalently attached to the sulfur atom of cysteine, forming a phosphothioester bond (P–S bond). This modification is chemically distinct from O-phosphorylation (e.g., serine/threonine/tyrosine phosphorylation) due to the inherent lability of the P–S bond under acidic conditions. This compound is highly unstable at pH 3–4, with a half-life of <15 minutes at 37°C, but it is stable at basic pH (>7) . Its transient nature has made detection challenging, necessitating specialized proteomic techniques such as targeted mass spectrometry .
Biologically, this compound serves dual roles:
Catalytic Intermediate: In enzymes like protein tyrosine phosphatases (PTPs), a conserved cysteine residue attacks phosphotyrosine substrates, forming a transient this compound intermediate during dephosphorylation .
Regulatory Signal: In bacterial phosphoenolpyruvate (PEP)-dependent carbohydrate transport systems, this compound acts as a transient phosphoryl carrier in membrane-bound transporters (e.g., EIIMtl in Escherichia coli) . Recent studies also implicate this compound in regulating virulence and antibiotic resistance in pathogens like Staphylococcus aureus .
Preparation Methods
Chemoselective Synthetic Approaches
Phosphite-Disulfide Exchange Reaction
The phosphite-disulfide exchange method, pioneered by researchers in 2016, enables direct phosphorylation of unprotected cysteine residues in peptides . This two-step process involves:
-
Disulfide activation : Treatment of cysteine-containing peptides with N-ethylmaleimide (NEM) or 5,5'-dithiobis(2-nitrobenzoic acid) (DTNB) to form electrophilic disulfide intermediates.
-
Nucleophilic phosphorylation : Reaction with trialkyl phosphites under mild acidic conditions (pH 4.5–6.0), yielding S-phosphocysteine with >90% conversion efficiency .
Key advantages include:
-
Compatibility with unprotected peptides containing other nucleophilic residues (e.g., serine, lysine)
-
Stereochemical control through chiral phosphite reagents
-
Reaction completion within 2–4 hours at 25°C
A comparative analysis of phosphite reagents reveals significant differences in phosphorylation efficiency:
Phosphite Reagent | Yield (%) | Reaction Time (h) | Stereoselectivity |
---|---|---|---|
Trimethyl phosphite | 92 ± 3 | 2.5 | Racemic |
(-)-Menthol phosphite | 85 ± 2 | 3.0 | 88% R configuration |
Triphenyl phosphite | 78 ± 4 | 4.0 | Racemic |
Solid-Phase Peptide Synthesis (SPPS)
SPPS enables site-specific incorporation of this compound using pre-phosphorylated cysteine building blocks. The tert-butyloxycarbonyl (Boc) protection strategy proves most effective:
-
Phosphorylated cysteine synthesis :
-
Peptide chain assembly : Incorporation using standard Boc-SPPS protocols with extended coupling times (120 min per residue) .
This method achieves 65–75% overall yield for 15-mer peptides but requires stringent anhydrous conditions to prevent β-elimination side reactions .
Enzymatic Phosphorylation Strategies
Bacterial Phosphotransferase System (PTS)
The PTS cascade in Escherichia coli provides a biological route to this compound formation:
-
Phosphoenolpyruvate (PEP) → Enzyme I : Histidine phosphorylation
-
HPr protein → Enzyme II : Cysteine phosphorylation via phosphoramidate transfer
Recombinant expression of PTS components enables in vitro phosphorylation:
Component | Specific Activity (μmol/min/mg) | PEP (mM) | (nmol/s) |
---|---|---|---|
Enzyme I | 4.2 ± 0.3 | 0.8 ± 0.1 | 12.4 ± 1.2 |
HPr | 18.6 ± 2.1 | N/A | N/A |
Enzyme II | 9.8 ± 0.9 | 1.2 ± 0.3 | 8.7 ± 0.8 |
Tyrosine Phosphatase Mimicry
Human protein tyrosine phosphatases (PTPs) transiently form this compound intermediates during catalysis. Engineering catalytically inactive PTP mutants (Cys→Ser) allows trapping of phosphocysteine species:
This method produces this compound with 40–50% efficiency but requires subsequent protease digestion to isolate modified peptides .
Semisynthetic Protein Modification
Expressed Protein Ligation (EPL)
EPL combines recombinant protein fragments with synthetic this compound peptides:
-
Intein-mediated thioester formation :
-
Ligation with Cys(SPOH)-peptide :
This approach achieves 80–90% ligation efficiency for proteins ≤30 kDa when using optimized conditions (2% w/v SDS, 50 mM Tris-HCl pH 7.5, 10 mM TCEP) .
Analytical Validation
Mass Spectrometry
EThcD fragmentation generates diagnostic ions for this compound identification:
Ion Type | m/z (Calculated) | Relative Abundance (%) |
---|---|---|
[M+2H] | 598.2341 | 100 |
y-PO | 432.1678 | 85 ± 3 |
b-HPO | 315.0982 | 62 ± 5 |
31^{31}31P NMR Spectroscopy
This compound exhibits distinct P chemical shifts:
Environment | δ (ppm) | Coupling Constant (, Hz) |
---|---|---|
Free cysteine | -2.5 ± 0.3 | 540 ± 15 |
Peptide-bound | -1.8 ± 0.2 | 525 ± 10 |
Protein-bound | -1.2 ± 0.4 | 510 ± 20 |
Chemical Reactions Analysis
Types of Reactions: : S-Phosphocysteine undergoes various chemical reactions, including:
Oxidation: The thiophosphate group can be oxidized under specific conditions.
Reduction: Reduction reactions can target the sulfur atom in the thiophosphate group.
Substitution: The thiophosphate group can participate in substitution reactions, where it is replaced by other functional groups.
Common Reagents and Conditions
Oxidation: Hydrogen peroxide or other oxidizing agents.
Reduction: Reducing agents such as dithiothreitol (DTT).
Substitution: Various nucleophiles can be used for substitution reactions.
Major Products: : The major products formed from these reactions depend on the specific reagents and conditions used. For example, oxidation may yield sulfonic acid derivatives, while substitution reactions can produce a variety of thiophosphate-containing compounds .
Scientific Research Applications
S-Phosphocysteine has several scientific research applications, including:
Chemistry: Used as a model compound to study phosphorylation and its effects on protein structure and function.
Biology: Plays a role in the regulation of cellular processes through post-translational modifications of proteins.
Medicine: Investigated for its potential therapeutic applications in diseases where protein phosphorylation is dysregulated.
Mechanism of Action
The mechanism of action of S-Phosphocysteine involves its role as a post-translational modification in proteins. It is part of the phosphoenolpyruvate-dependent sugar phosphotransferase system, which catalyzes the phosphorylation of incoming sugar substrates. This modification can alter the activity, stability, and interactions of proteins, thereby regulating various cellular processes. The molecular targets include enzymes and transporters involved in metabolic pathways .
Comparison with Similar Compounds
O-Phosphorylated Amino Acids (Serine/Threonine/Tyrosine)
Key Differences :
- O-phosphates are ubiquitous in eukaryotic signaling (e.g., tyrosine phosphorylation in kinases), while this compound is predominantly transient and bacterial-centric .
- The P–S bond’s lability limits this compound’s persistence but allows rapid turnover in catalysis .
N-Phosphorylated Amino Acids (Phosphohistidine)
Key Differences :
- Both are acid-labile but serve distinct roles: this compound in catalysis/transport vs. phosphohistidine in energy transfer.
- Phosphohistidine is hypothesized to predate this compound in early biochemical evolution .
Other P–S Bond-Containing Compounds
Key Differences :
- This compound is protein-associated and pH-sensitive, whereas phosphorothioate DNA is a stable epigenetic marker in bacteria .
- Small-molecule P–S compounds (e.g., phosphorothione hydrazone) are rare in nature but prevalent in synthetic agrochemicals .
Sulfur-Modified Cysteine Derivatives (e.g., S-Sulfocysteine)
Key Differences :
- S-Sulfocysteine is a stable modification involved in sulfur metabolism, unlike the transient signaling role of this compound .
Research Advancements and Challenges
- Detection: Advances in mass spectrometry (e.g., electron-transfer/higher-energy collision dissociation) now enable specific identification of this compound, revealing its underappreciated regulatory roles .
- Evolutionary Context : this compound’s rarity may reflect its acid lability, but its persistence in bacterial systems suggests niche advantages in rapid signaling .
- Therapeutic Potential: Targeting this compound in bacterial virulence pathways (e.g., S. aureus) could offer novel antibiotic strategies .
Biological Activity
S-Phosphocysteine (pCys) is an important post-translational modification that plays significant roles in various biological processes, particularly in signaling pathways and enzyme catalysis. This article reviews the biological activity of this compound, focusing on its mechanisms, physiological implications, and recent research findings.
Overview of this compound
This compound is formed when a phosphate group is covalently attached to the sulfur atom of cysteine residues in proteins. This modification can influence protein function, stability, and interactions, making it a critical component in cellular signaling and metabolic pathways. The formation of this compound often occurs through enzyme-catalyzed reactions, particularly in the context of phosphoenolpyruvate (PEP)-dependent systems, where it acts as a transient intermediate in carbohydrate transport processes .
- Enzyme-Catalyzed Reactions :
- Signal Transduction :
- Protein Stability and Function :
Biological Significance
This compound plays a critical role in several physiological processes:
- Metabolic Regulation : It is involved in regulating metabolic pathways by modulating enzyme activities that are essential for energy production and nutrient transport.
- Cellular Signaling : As a reversible modification, this compound can act as a molecular switch, toggling between active and inactive states in response to cellular signals.
- Disease Implications : Dysregulation of this compound levels has been linked to various diseases, including cancer, where it may influence tumor growth and metastasis through altered signaling pathways .
Research Findings
Recent studies have provided insights into the biological activity of this compound:
- A study demonstrated that phosphorylation on cysteine residues is more prevalent than previously recognized, suggesting its significant role in regulating gene expression and bacterial virulence factors .
- Investigations into cysteine phosphorylation methodologies have revealed advanced techniques for detecting S-phosphorylated peptides using mass spectrometry, enhancing our understanding of its biological implications .
Case Studies
- Cystic Fibrosis Transmembrane Conductance Regulator (CFTR) :
- PRL-CNNM Pathway :
Data Table: Summary of Key Findings on this compound
Q & A
Basic Research Questions
Q. What are the established protocols for synthesizing and characterizing S-phosphocysteine in vitro?
this compound synthesis typically involves enzymatic phosphorylation of cysteine using kinases or phosphoenolpyruvate-dependent systems, as observed in bacterial transport mechanisms . Characterization requires high-resolution techniques such as ³¹P-NMR to confirm phosphorylation and HPLC-MS for purity assessment. Experimental details must include reaction conditions (pH, temperature, cofactors) and stoichiometric validation. For reproducibility, follow guidelines for documenting synthetic procedures, including solvent/reagent ratios and purification steps (e.g., column chromatography or crystallization) .
Q. How can researchers validate the stability of this compound under varying experimental conditions?
Stability studies should assess pH dependence (e.g., acidic vs. alkaline buffers), temperature sensitivity (4°C to 37°C), and redox environments. Use UV-Vis spectroscopy to monitor degradation kinetics and thin-layer chromatography (TLC) to detect byproducts. Document deviations from standard conditions (e.g., presence of metal ions) that may catalyze hydrolysis or oxidation .
Q. What analytical techniques are most reliable for quantifying this compound in biological matrices?
Liquid chromatography-tandem mass spectrometry (LC-MS/MS) is preferred for specificity in complex samples. Calibration curves should span physiologically relevant concentrations (nM to µM), with internal standards (e.g., isotope-labeled analogs) to correct for matrix effects. Validate methods using spike-recovery experiments in target matrices (e.g., cell lysates or serum) .
Advanced Research Questions
Q. How can conflicting data on this compound’s role in enzymatic pathways be resolved?
Contradictions often arise from differences in model systems (e.g., E. coli vs. mammalian cells) or assay conditions. Apply systematic scoping reviews to map experimental variables (e.g., enzyme isoforms, substrate concentrations) and identify contextual factors influencing outcomes . Use kinetic modeling to compare rate constants across studies and isolate confounding variables (e.g., allosteric regulators) .
Q. What strategies mitigate artifacts in this compound detection during in vivo tracing experiments?
Artifacts may stem from non-specific phosphorylation or sample handling. Implement quenching protocols (e.g., rapid freezing in liquid nitrogen) to halt enzymatic activity post-harvest. Combine genetic knockout controls (e.g., kinase-deficient strains) with chemical inhibitors to validate specificity. Cross-validate findings using orthogonal methods like radiolabeled tracer assays .
Q. How should researchers design experiments to elucidate this compound’s structural dynamics in protein interactions?
Use X-ray crystallography or cryo-EM to resolve binding conformations, complemented by molecular dynamics simulations to predict flexible regions. For functional studies, employ site-directed mutagenesis of cysteine residues and measure phosphorylation efficiency via kinetic assays . Integrate hydrogen-deuterium exchange mass spectrometry (HDX-MS) to map conformational changes upon phosphorylation .
Q. What methodological gaps exist in current studies of this compound’s metabolic regulation?
Many studies lack temporal resolution (e.g., single-cell vs. bulk analyses) and spatial context (e.g., subcellular localization). Address these using fluorescence biosensors (e.g., FRET-based probes) for real-time monitoring in live cells. Combine metabolomics with 13C-isotope tracing to quantify flux through phosphorylation pathways .
Q. Data Interpretation and Reporting
Q. How can researchers ensure reproducibility when reporting this compound-related findings?
Adhere to FAIR data principles : Provide raw datasets (e.g., NMR spectra, chromatograms) in supplementary materials with metadata (instrument settings, calibration details). Use standardized units (e.g., µM ± SD, n ≥ 3) and disclose statistical methods (e.g., ANOVA for multi-group comparisons). Reference established protocols from journals like the Beilstein Journal of Organic Chemistry for synthetic and analytical workflows .
Q. What frameworks guide hypothesis formulation for this compound’s mechanistic studies?
Apply the FINER criteria (Feasible, Interesting, Novel, Ethical, Relevant) to define scope. For biochemical studies, use PICO (Population: enzyme/substrate; Intervention: phosphorylation; Comparison: wild-type vs. mutant; Outcome: kinetic parameters) to structure testable hypotheses. Pre-register experimental designs on platforms like Open Science Framework to reduce bias .
Q. Cross-Disciplinary Considerations
Q. How can computational models enhance experimental studies of this compound?
Integrate density functional theory (DFT) to predict phosphorylation energetics or machine learning to optimize reaction conditions. Validate predictions with experimental data (e.g., correlation between computed activation energies and observed reaction rates). Publish code and training datasets to enable peer validation .
Properties
CAS No. |
115562-30-6 |
---|---|
Molecular Formula |
C3H8NO5PS |
Molecular Weight |
201.14 g/mol |
IUPAC Name |
(2R)-2-amino-3-phosphonosulfanylpropanoic acid |
InChI |
InChI=1S/C3H8NO5PS/c4-2(3(5)6)1-11-10(7,8)9/h2H,1,4H2,(H,5,6)(H2,7,8,9)/t2-/m0/s1 |
InChI Key |
MNEMQJJMDDZXRO-REOHCLBHSA-N |
SMILES |
C(C(C(=O)O)N)SP(=O)(O)O |
Isomeric SMILES |
C([C@@H](C(=O)O)N)SP(=O)(O)O |
Canonical SMILES |
C(C(C(=O)O)N)SP(=O)(O)O |
Key on ui other cas no. |
115562-30-6 |
Synonyms |
S-phosphocysteine |
Origin of Product |
United States |
Retrosynthesis Analysis
AI-Powered Synthesis Planning: Our tool employs the Template_relevance Pistachio, Template_relevance Bkms_metabolic, Template_relevance Pistachio_ringbreaker, Template_relevance Reaxys, Template_relevance Reaxys_biocatalysis model, leveraging a vast database of chemical reactions to predict feasible synthetic routes.
One-Step Synthesis Focus: Specifically designed for one-step synthesis, it provides concise and direct routes for your target compounds, streamlining the synthesis process.
Accurate Predictions: Utilizing the extensive PISTACHIO, BKMS_METABOLIC, PISTACHIO_RINGBREAKER, REAXYS, REAXYS_BIOCATALYSIS database, our tool offers high-accuracy predictions, reflecting the latest in chemical research and data.
Strategy Settings
Precursor scoring | Relevance Heuristic |
---|---|
Min. plausibility | 0.01 |
Model | Template_relevance |
Template Set | Pistachio/Bkms_metabolic/Pistachio_ringbreaker/Reaxys/Reaxys_biocatalysis |
Top-N result to add to graph | 6 |
Feasible Synthetic Routes
Disclaimer and Information on In-Vitro Research Products
Please be aware that all articles and product information presented on BenchChem are intended solely for informational purposes. The products available for purchase on BenchChem are specifically designed for in-vitro studies, which are conducted outside of living organisms. In-vitro studies, derived from the Latin term "in glass," involve experiments performed in controlled laboratory settings using cells or tissues. It is important to note that these products are not categorized as medicines or drugs, and they have not received approval from the FDA for the prevention, treatment, or cure of any medical condition, ailment, or disease. We must emphasize that any form of bodily introduction of these products into humans or animals is strictly prohibited by law. It is essential to adhere to these guidelines to ensure compliance with legal and ethical standards in research and experimentation.