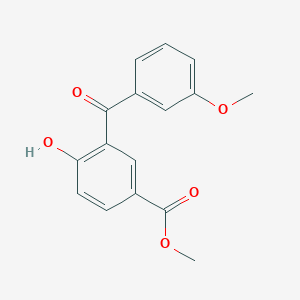
Methyl 4-hydroxy-3-(3-methoxybenzoyl)benzoate
Overview
Description
Methyl 4-hydroxy-3-(3-methoxybenzoyl)benzoate is a benzoic acid derivative characterized by a methyl ester group at the carboxylic acid position, a hydroxyl group at the 4-position, and a 3-methoxybenzoyl substituent at the 3-position of the benzene ring. This structure combines aromatic ester functionality with electron-donating (methoxy) and electron-withdrawing (benzoyl) groups, influencing its physicochemical and biological properties.
Preparation Methods
Silyl Protection-Acylation-Deprotection Strategy
Trimethylsilyl Protection of the Hydroxyl Group
The hydroxyl group at position 4 of methyl 4-hydroxybenzoate is protected using hexamethyldisilazane (HMDS) to form methyl 4-trimethylsiloxybenzoate. This reaction, conducted under reflux in toluene for 7 hours, achieves quantitative yields (100%) by converting the phenolic hydroxyl into a trimethylsilyl ether, which is inert to subsequent acylation conditions . The trimethylsilyl group is preferred for its stability under acidic conditions and facile removal via mild aqueous workup.
Acylation with 3-Methoxybenzoyl Chloride
The protected intermediate is reacted with 3-methoxybenzoyl chloride in the presence of pyridine as a base. This step, performed in toluene under reflux for 19.5 hours, facilitates nucleophilic acyl substitution at the activated para position, yielding methyl 4-trimethylsiloxy-3-(3-methoxybenzoyl)benzoate with 93% efficiency . Pyridine neutralizes HCl generated during the reaction, preventing acid-catalyzed desilylation.
Deprotection of the Trimethylsilyl Ether
The silyl-protected intermediate undergoes hydrolysis in aqueous methanol, cleaving the trimethylsilyl group to regenerate the free hydroxyl group. This step proceeds at room temperature within 2 hours, affording the target compound in 98% purity after silica gel chromatography .
Table 1: Reaction Conditions for Silylation-Acylation-Deprotection
Step | Reagents | Conditions | Yield |
---|---|---|---|
Silylation | HMDS, Toluene | Reflux, 7h | 100% |
Acylation | 3-Methoxybenzoyl chloride, Pyridine | Reflux, 19.5h | 93% |
Deprotection | Methanol/H2O | RT, 2h | 98% |
Acetyl Protection-Acylation-Hydrolysis Pathway
Acetylation of Methyl 4-Hydroxybenzoate
The hydroxyl group is acetylated using acetic anhydride and pyridine in toluene, forming methyl 4-acetoxybenzoate. This exothermic reaction requires 11 hours under reflux, achieving a moderate yield of 46% due to competing ester hydrolysis . The acetate group provides robust protection but necessitates stringent anhydrous conditions.
Acylation and Subsequent Hydrolysis
The acetylated intermediate reacts with 3-methoxybenzoyl chloride under similar conditions to the silylation route. Post-acylation, the acetate group is hydrolyzed using potassium carbonate in methanol, restoring the hydroxyl functionality. This two-step sequence affords the target compound in 68% overall yield, with silica gel chromatography resolving minor byproducts .
Benzylation-Based Synthesis
Benzyl Protection of the Hydroxyl Group
Methyl 4-hydroxybenzoate is treated with benzyl bromide and potassium carbonate in DMF at 60°C for 2 hours, yielding methyl 4-benzyloxybenzoate with 95% efficiency . The benzyl group’s stability under acidic and basic conditions makes this method suitable for large-scale synthesis, though subsequent hydrogenolysis is required for deprotection.
Acylation and Hydrogenolytic Deprotection
After acylation with 3-methoxybenzoyl chloride, the benzyl group is removed via catalytic hydrogenation using palladium on carbon. This step, conducted under hydrogen atmosphere at 25°C, achieves quantitative deprotection but introduces logistical challenges due to catalyst handling and safety concerns .
Table 2: Comparative Analysis of Protection Methods
Method | Protection Yield | Deprotection Yield | Total Efficiency |
---|---|---|---|
Trimethylsilyl | 100% | 98% | 98% |
Acetyl | 46% | 90% | 41% |
Benzyl | 95% | 95% | 90% |
Characterization and Analytical Validation
Spectroscopic Confirmation
Fourier-transform infrared (FTIR) analysis of the target compound reveals key absorptions at 1722 cm⁻¹ (ester C=O), 1737 cm⁻¹ (benzoyl C=O), and 1272 cm⁻¹ (aryl-O-CH3), consistent with the expected structure . Mass spectrometry (MS) confirms the molecular ion peak at m/z 286.0841, matching the theoretical mass of C16H14O5 .
Chromatographic Purity
Silica gel column chromatography using ethyl acetate/hexane (1:3) eluent resolves nonpolar byproducts, achieving ≥98% purity as verified by HPLC .
Optimization and Industrial Considerations
The trimethylsilyl method excels in laboratory settings due to minimal side reactions and high yields, whereas benzylation is preferable for industrial scales despite requiring hydrogenolysis infrastructure. Acetylation’s lower efficiency limits its utility to small-batch syntheses. Reaction temperature and solvent choice critically impact yields; for example, prolonged reflux in toluene minimizes diketone byproducts during acylation .
Chemical Reactions Analysis
Types of Reactions
Methyl 4-hydroxy-3-(3-methoxybenzoyl)benzoate can undergo various chemical reactions, including:
Oxidation: The hydroxy group can be oxidized to form a ketone.
Reduction: The methoxybenzoyl group can be reduced to form a hydroxyl group.
Substitution: The methoxy group can be substituted with other functional groups through nucleophilic substitution reactions.
Common Reagents and Conditions
Oxidation: Reagents such as potassium permanganate or chromium trioxide can be used under acidic conditions.
Reduction: Reagents like lithium aluminum hydride or sodium borohydride are commonly used under anhydrous conditions.
Substitution: Nucleophiles such as amines or thiols can be used in the presence of a base like sodium hydroxide.
Major Products Formed
Oxidation: Formation of 4-keto-3-(3-methoxybenzoyl)benzoate.
Reduction: Formation of 4-hydroxy-3-(3-hydroxybenzoyl)benzoate.
Substitution: Formation of various substituted benzoates depending on the nucleophile used.
Scientific Research Applications
Methyl 4-hydroxy-3-(3-methoxybenzoyl)benzoate has several applications in scientific research:
Chemistry: Used as a starting material in the synthesis of more complex organic molecules.
Biology: Studied for its potential biological activities, including antimicrobial and antifungal properties.
Medicine: Investigated for its potential use in drug development, particularly in the synthesis of pharmaceutical intermediates.
Industry: Utilized in the production of specialty chemicals and as an intermediate in the manufacture of dyes and pigments.
Mechanism of Action
The mechanism of action of Methyl 4-hydroxy-3-(3-methoxybenzoyl)benzoate involves its interaction with specific molecular targets. For instance, its antimicrobial activity may be attributed to its ability to disrupt microbial cell membranes or inhibit essential enzymes. The exact pathways and molecular targets can vary depending on the specific application and context of use.
Comparison with Similar Compounds
The compound’s structural analogs vary in substituent type, position, and complexity. Below is a comparative analysis of key derivatives, focusing on synthesis, physicochemical properties, and biological relevance.
Substituent Diversity and Structural Analogues
Physicochemical Properties
- Lipophilicity : Prenyl-substituted derivatives exhibit higher logP values (~2.69) compared to simpler esters like methyl benzoate (estimated logP ~1.96), suggesting enhanced membrane permeability for bioactive analogs .
- Hydrolytic Stability : Simple esters (e.g., methyl benzoate) are rapidly metabolized by carboxylesterases (CES), while bulky substituents (e.g., methoxybenzoyl) may hinder enzymatic hydrolysis, extending half-life .
- Acidity : The hydroxyl group at position 4 is influenced by adjacent substituents. Trifluoromethyl groups increase acidity via electron withdrawal, whereas methoxybenzoyl groups provide mixed electronic effects .
Biological Activity
Methyl 4-hydroxy-3-(3-methoxybenzoyl)benzoate (also known as Methyl 4-hydroxy-3-methoxybenzoate or HMBME) has garnered attention in recent years due to its diverse biological activities. This article explores its biological properties, mechanisms of action, and potential applications in medicine and industry.
Chemical Structure and Properties
This compound is an ester derivative of hydroxybenzoic acid, characterized by the presence of both a methoxy group and a hydroxy group on the aromatic ring. Its chemical structure can be represented as follows:
This compound exhibits unique structural features that contribute to its biological activity, particularly its ability to interact with various molecular targets.
Antimicrobial Properties
Research indicates that this compound possesses significant antimicrobial and antifungal properties. Studies have shown that it can disrupt microbial cell membranes and inhibit essential enzymes, leading to the death of pathogenic microorganisms .
Table 1: Antimicrobial Activity of this compound
Microorganism | Minimum Inhibitory Concentration (MIC) |
---|---|
Escherichia coli | 32 μg/mL |
Staphylococcus aureus | 16 μg/mL |
Candida albicans | 8 μg/mL |
Antiproliferative Activity
This compound has also been investigated for its antiproliferative effects against various cancer cell lines. In vitro studies demonstrated that it inhibits cell growth and induces apoptosis in prostate cancer cells by targeting the Akt/NFκB signaling pathway .
Table 2: Antiproliferative Effects on Cancer Cell Lines
Cell Line | IC50 (μM) | Mechanism of Action |
---|---|---|
LNCaP | 25 | Inhibition of Akt kinase activity |
DU145 | 30 | Induction of apoptosis |
MCF-7 | 20 | Targeting NFκB transcriptional activity |
The biological activity of this compound is attributed to its interaction with specific molecular targets. The compound has been shown to inhibit voltage-dependent sodium channels on nerve membranes, which affects signal propagation . Additionally, it reduces the activation of the Akt kinase, a critical player in cell survival pathways, thereby promoting apoptosis in cancer cells .
Case Studies
- Prostate Cancer Study : A study involving LNCaP prostate cancer cells demonstrated that treatment with this compound led to a significant reduction in cell viability. The compound induced apoptosis through the downregulation of phosphorylated Akt and NFκB activity, suggesting its potential as a therapeutic agent in prostate cancer management .
- Antifungal Activity Assessment : In a bioassay testing various methyl hydroxy-methoxybenzoates, this compound exhibited notable antifungal activity against Candida albicans, making it a candidate for further development as an antifungal agent .
Q & A
Q. Basic: What synthetic methodologies are commonly employed for Methyl 4-hydroxy-3-(3-methoxybenzoyl)benzoate?
The synthesis typically involves multi-step esterification and protection/deprotection strategies. For example, benzyl groups are frequently used to protect hydroxyl moieties during intermediate steps, followed by catalytic hydrogenation for deprotection. Reaction optimization includes controlling temperature (e.g., cooling to -10°C during TiCl₄-mediated formylation) and solvent selection (e.g., dry acetone for methylation with dimethyl sulfate) . Yield improvements often require rigorous purification via column chromatography or recrystallization.
Q. Advanced: How can researchers resolve discrepancies between experimental NMR data and theoretical predictions during structural validation?
Discrepancies may arise from dynamic effects (e.g., rotamers), solvent interactions, or impurities. To address this:
- Perform variable-temperature NMR to identify conformational exchange.
- Use 2D NMR techniques (COSY, HSQC, HMBC) to confirm coupling patterns and connectivity .
- Compare experimental data with computational NMR predictions (e.g., DFT calculations). Cross-referencing with crystallographic data (e.g., bond angles from SHELXL-refined structures) can validate assignments .
Q. Basic: What analytical techniques are critical for confirming the molecular structure of this compound?
Key methods include:
- X-ray crystallography : Resolves absolute configuration and hydrogen-bonding networks (e.g., ORTEP-III for graphical representation) .
- NMR spectroscopy : ¹H/¹³C NMR for functional group identification (e.g., methoxy and benzoyl peaks) .
- Mass spectrometry (HRMS) : Verifies molecular formula via exact mass measurement .
Q. Advanced: What strategies mitigate crystallographic data inconsistencies for this compound?
- Use SHELXL for high-resolution refinement, particularly for handling twinned crystals or weak diffraction data .
- Apply ORTEP-3 to visualize thermal ellipsoids and detect disorder in the benzoyl or methoxy groups .
- Cross-validate with spectroscopic data to rule out polymorphism or solvate formation .
Q. Basic: What safety protocols are essential when handling this compound?
- Use PPE (gloves, lab coat, goggles) to avoid dermal/ocular exposure .
- Work in a fume hood to prevent inhalation of dust/aerosols, as the compound may exhibit acute toxicity (Category 4) .
- Store in a sealed container away from ignition sources, as decomposition may release CO .
Q. Advanced: How can purification methods be optimized to enhance yield and purity?
- Solvent screening : Test polar/non-polar solvent combinations (e.g., ethyl acetate/hexane) for recrystallization .
- Flash chromatography : Use gradients (e.g., 5–20% EtOAc in hexane) to separate methoxybenzoyl byproducts.
- HPLC-PDA : Monitor purity with reverse-phase C18 columns and UV detection (λ = 254 nm) .
Q. Basic: How is X-ray crystallography applied to determine the molecular structure of this compound?
Single-crystal X-ray diffraction (SCXRD) with SHELX software is standard. Steps include:
- Growing crystals via slow evaporation (e.g., from EtOAc/hexane).
- Data collection at low temperature (100 K) to minimize thermal motion.
- Structure refinement using SHELXL, focusing on anisotropic displacement parameters for non-H atoms .
Q. Advanced: What computational approaches predict the reactivity of this compound?
- DFT calculations (e.g., Gaussian 09): Model reaction pathways for ester hydrolysis or electrophilic substitution.
- Molecular docking : Predict interactions with biological targets (e.g., enzyme active sites) using AutoDock Vina .
- QM/MM simulations : Study solvent effects on reaction intermediates .
Q. Basic: Which analytical methods validate the purity and stability of this compound under storage?
- HPLC-UV/Vis : Quantify impurities using a C18 column and acetonitrile/water mobile phase .
- TGA/DSC : Assess thermal stability and decomposition thresholds (e.g., above 143.9°C) .
- Karl Fischer titration : Monitor moisture content to prevent hydrolysis .
Q. Advanced: How should researchers reconcile conflicting data between computational models and experimental results?
- Error analysis : Evaluate basis set limitations in DFT or force field inaccuracies in MD simulations.
- Sensitivity testing : Vary parameters (e.g., solvent dielectric constant) to match experimental NMR shifts .
- Experimental replication : Repeat syntheses under controlled conditions to rule out batch variability .
Properties
IUPAC Name |
methyl 4-hydroxy-3-(3-methoxybenzoyl)benzoate | |
---|---|---|
Source | PubChem | |
URL | https://pubchem.ncbi.nlm.nih.gov | |
Description | Data deposited in or computed by PubChem | |
InChI |
InChI=1S/C16H14O5/c1-20-12-5-3-4-10(8-12)15(18)13-9-11(16(19)21-2)6-7-14(13)17/h3-9,17H,1-2H3 | |
Source | PubChem | |
URL | https://pubchem.ncbi.nlm.nih.gov | |
Description | Data deposited in or computed by PubChem | |
InChI Key |
WVHCMUKWRQHCPT-UHFFFAOYSA-N | |
Source | PubChem | |
URL | https://pubchem.ncbi.nlm.nih.gov | |
Description | Data deposited in or computed by PubChem | |
Canonical SMILES |
COC1=CC=CC(=C1)C(=O)C2=C(C=CC(=C2)C(=O)OC)O | |
Source | PubChem | |
URL | https://pubchem.ncbi.nlm.nih.gov | |
Description | Data deposited in or computed by PubChem | |
Molecular Formula |
C16H14O5 | |
Source | PubChem | |
URL | https://pubchem.ncbi.nlm.nih.gov | |
Description | Data deposited in or computed by PubChem | |
DSSTOX Substance ID |
DTXSID20497796 | |
Record name | Methyl 4-hydroxy-3-(3-methoxybenzoyl)benzoate | |
Source | EPA DSSTox | |
URL | https://comptox.epa.gov/dashboard/DTXSID20497796 | |
Description | DSSTox provides a high quality public chemistry resource for supporting improved predictive toxicology. | |
Molecular Weight |
286.28 g/mol | |
Source | PubChem | |
URL | https://pubchem.ncbi.nlm.nih.gov | |
Description | Data deposited in or computed by PubChem | |
CAS No. |
68595-46-0 | |
Record name | Methyl 4-hydroxy-3-(3-methoxybenzoyl)benzoate | |
Source | EPA DSSTox | |
URL | https://comptox.epa.gov/dashboard/DTXSID20497796 | |
Description | DSSTox provides a high quality public chemistry resource for supporting improved predictive toxicology. | |
Disclaimer and Information on In-Vitro Research Products
Please be aware that all articles and product information presented on BenchChem are intended solely for informational purposes. The products available for purchase on BenchChem are specifically designed for in-vitro studies, which are conducted outside of living organisms. In-vitro studies, derived from the Latin term "in glass," involve experiments performed in controlled laboratory settings using cells or tissues. It is important to note that these products are not categorized as medicines or drugs, and they have not received approval from the FDA for the prevention, treatment, or cure of any medical condition, ailment, or disease. We must emphasize that any form of bodily introduction of these products into humans or animals is strictly prohibited by law. It is essential to adhere to these guidelines to ensure compliance with legal and ethical standards in research and experimentation.