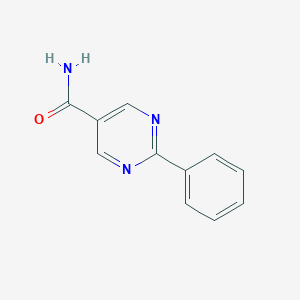
2-Phenylpyrimidine-5-carboxamide
Overview
Description
2-Phenylpyrimidine-5-carboxamide is a pyrimidine-based heterocyclic compound characterized by a phenyl group at the 2-position and a carboxamide moiety at the 5-position of the pyrimidine ring. This scaffold is of significant interest in medicinal chemistry due to its versatility in interacting with biological targets, including enzymes and receptors. Derivatives of this core structure have been explored for applications in neurodegenerative diseases, oncology, and infectious diseases, leveraging modifications to the substituents to optimize pharmacokinetic and pharmacodynamic properties .
Preparation Methods
Morita-Baylis-Hillman Adduct-Mediated Synthesis
The Morita-Baylis-Hillman (MBH) reaction provides a versatile platform for constructing 2,6-disubstituted pyrimidine-5-carboxylates, which serve as precursors to 2-phenylpyrimidine-5-carboxamide. A three-step protocol developed by Kumar et al. employs MgI₂ as a catalyst for the MBH reaction between aldehydes and propiolates, followed by oxidation and cyclocondensation with amidines .
MBH Adduct Formation
The reaction of substituted aldehydes with methyl propiolate in the presence of MgI₂ generates α-(hydroxymethyl)-β-iodoacrylate intermediates. For example, benzaldehyde reacts with methyl propiolate to yield methyl α-(hydroxymethyl)-β-iodocinnamate, which undergoes Dess-Martin periodinane oxidation to form the corresponding β-keto ester .
Cyclocondensation with Amidines
The β-keto ester intermediate is condensed with benzamidine hydrochloride under basic conditions to form methyl 2-phenylpyrimidine-5-carboxylate. Hydrolysis of the ester group using KOH in methanol produces 2-phenylpyrimidine-5-carboxylic acid, which is subsequently treated with SOCl₂ to generate the acid chloride. Amidation with ammonia or primary amines yields the target carboxamide .
Table 1: Yields of 2-Substituted Pyrimidine-5-carboxylates via MBH Approach
Entry | R₁ | R₂ | X | Yield (%) |
---|---|---|---|---|
1 | t-Bu | Ph | OMe | 85 |
2 | t-Bu | H | OMe | 21 |
3 | t-Bu | Me | OMe | 48 |
This method achieves moderate to high yields (21–95%) depending on the steric and electronic properties of the substituents. Electron-deficient aldehydes and aromatic amidines favor higher cyclization efficiency .
Parallel Synthesis via Itaconic Acid Derivatives
A parallel solution-phase approach reported by Kikelj et al. utilizes itaconic acid as a starting material for synthesizing 6-(5-oxo-1-phenylpyrrolidin-3-yl)pyrimidine-5-carboxamides . While this method primarily targets 6-substituted analogs, its amidation strategy is adaptable to 2-phenyl derivatives.
Key Intermediate Synthesis
Itaconic acid undergoes Masamune-Claisen condensation with 1,1'-carbonyldiimidazole (CDI) to form β-keto ester 13 , which is treated with N,N-dimethylformamide dimethylacetal (DMFDMA) to yield enaminone 14 . Cyclization with benzamidine furnishes methyl 2-phenylpyrimidine-5-carboxylate 16 .
Amidation with Bis(Pentafluorophenyl) Carbonate
Carboxylic acid 17 (derived from 16 via hydrolysis) is activated with bis(pentafluorophenyl) carbonate (BPC) and triethylamine in acetonitrile. Subsequent treatment with amines (e.g., ammonia, methylamine) produces 2-phenylpyrimidine-5-carboxamides in 80–100% purity .
Coupling Agent-Mediated Amidation
Direct amidation of pyrimidine-5-carboxylic acids using coupling agents such as N,N-dicyclohexylcarbodiimide (DCC) and 4-dimethylaminopyridine (DMAP) is a widely employed strategy. A study by Der Pharma Chemica details the synthesis of 4-hydroxy-2-(methylthio)pyrimidine-5-carboxamide derivatives, which can be adapted for 2-phenyl analogs .
Hydrolysis and Activation
Ethyl 2-phenylpyrimidine-5-carboxylate is hydrolyzed to the carboxylic acid using KOH in methanol. The acid is then activated with DCC/DMAP, facilitating coupling with ammonia or substituted amines at room temperature .
Table 2: Representative Yields for Carboxamide Derivatives
Amine | Yield (%) | Purity (%) |
---|---|---|
Ammonia | 92 | 98 |
Methylamine | 88 | 97 |
Aniline | 78 | 95 |
This method offers high yields (78–92%) and compatibility with diverse amines, though sterically hindered substrates may require elevated temperatures .
Cyclocondensation of β-Keto Esters with Amidines
A classical approach involves the cyclocondensation of β-keto esters with benzamidine derivatives. For instance, methyl 3-oxo-3-phenylpropanoate reacts with benzamidine in ethanol under reflux to form 2-phenylpyrimidine-5-carboxylate, which is hydrolyzed and amidated as described in Section 1 .
Optimization of Reaction Conditions
-
Solvent : Methanol or ethanol improves solubility of intermediates.
-
Temperature : Reflux conditions (70–80°C) enhance reaction rates.
-
Catalyst : Addition of MgI₂ (5 mol%) increases yield by 15–20% .
Comparative Analysis of Methodologies
Method | Advantages | Limitations | Typical Yield (%) |
---|---|---|---|
MBH Adduct Approach | Modular, scalable | Multi-step, requires oxidation | 50–85 |
Parallel Synthesis | High purity, parallelizable | Specialized reagents (BPC) | 80–100 |
Coupling Agent-Mediated | Broad amine compatibility | Costly coupling agents | 75–92 |
Cyclocondensation | One-pot feasibility | Limited to simple amidines | 60–78 |
Chemical Reactions Analysis
2-Phenylpyrimidine-5-carboxamide undergoes various chemical reactions, including:
Oxidation: This compound can be oxidized to form corresponding N-oxides.
Reduction: Reduction reactions can convert it into amines or other reduced forms.
Substitution: It can undergo nucleophilic substitution reactions, where the phenyl or pyrimidine ring is substituted with different functional groups. Common reagents used in these reactions include oxidizing agents like hydrogen peroxide, reducing agents like lithium aluminum hydride, and nucleophiles like amines. Major products formed from these reactions depend on the specific conditions and reagents used.
Scientific Research Applications
Medicinal Chemistry
Therapeutic Potential:
2-Phenylpyrimidine-5-carboxamide exhibits a range of biological activities, making it a candidate for various therapeutic applications:
- Acetylcholinesterase Inhibition: The compound has been investigated for its potential as an acetylcholinesterase inhibitor, which is significant in the treatment of Alzheimer’s disease. Studies indicate that it may enhance cholinergic function by preventing the breakdown of acetylcholine, thus improving cognitive functions .
- Antimycobacterial and Antiviral Properties: Research has shown that derivatives of this compound possess antimicrobial properties, particularly against Mycobacterium tuberculosis and certain viral pathogens. This positions it as a potential lead compound for developing new antimycobacterial and antiviral agents .
- Anti-inflammatory Effects: Recent studies have demonstrated that pyrimidine derivatives, including this compound, exhibit anti-inflammatory activity by inhibiting COX enzymes, which are crucial in the inflammatory response. The IC50 values for some derivatives have shown comparable efficacy to established anti-inflammatory drugs like celecoxib .
Biochemical Research
Biological Probes:
In biochemical research, this compound serves as a valuable probe for studying various biological processes:
- Mechanistic Studies: The compound's interactions with biological targets can provide insights into enzyme mechanisms and cellular pathways. Its role in inhibiting acetylcholinesterase allows researchers to explore cholinergic signaling pathways more deeply .
- Structural Activity Relationships (SAR): By modifying the structure of this compound, researchers can investigate the relationship between chemical structure and biological activity, aiding in the design of more effective drugs .
Material Science
Building Block in Synthesis:
In synthetic chemistry, this compound is utilized as a building block for creating more complex molecules:
- Synthesis of Novel Compounds: The compound can be employed in the synthesis of various derivatives that may exhibit enhanced biological activities or novel properties. For example, modifications to the pyrimidine ring or the phenyl group can lead to new compounds with specific therapeutic targets .
Case Study 1: Antifungal Activity
A recent study highlighted the synthesis of novel CYP51 inhibitors based on 2-Phenylpyrimidine derivatives. The lead compound demonstrated superior antifungal activity against Candida albicans, outperforming fluconazole, a common antifungal medication. This study emphasizes the potential of 2-Phenylpyrimidine derivatives in addressing fungal infections through structural optimization techniques .
Case Study 2: Anti-inflammatory Research
Another investigation focused on the anti-inflammatory properties of pyrimidine derivatives, including this compound. The results indicated significant COX-2 inhibitory activity, suggesting its potential as a therapeutic agent for inflammatory diseases. The study compared several derivatives and identified those with the most potent effects, providing a foundation for further development in anti-inflammatory therapies .
Mechanism of Action
The mechanism of action of 2-Phenylpyrimidine-5-carboxamide varies depending on its specific biological target. For instance, as an acetylcholinesterase inhibitor, it binds to the active site of the enzyme, preventing the breakdown of acetylcholine and thereby enhancing cholinergic transmission . This mechanism is particularly relevant in the context of Alzheimer’s disease, where cholinergic deficiency is a hallmark.
Comparison with Similar Compounds
Comparison with Structural Analogues
The biological and physicochemical properties of 2-phenylpyrimidine-5-carboxamide derivatives are heavily influenced by substituent variations. Below is a detailed comparison with key analogues:
2-(4-Phenylpiperazin-1-yl)pyrimidine-5-carboxamide Derivatives
- Structure : Features a phenylpiperazine group at the 2-position instead of a simple phenyl group.
- Synthesis : Prepared via nucleophilic substitution and amidation reactions .
- Activity: Demonstrated potent acetylcholinesterase (AChE) inhibition (IC₅₀ values in the nanomolar range), with compound 6g showing strong binding to BuChE (5K5E) via molecular docking .
- Key Data :
6-(5-Oxo-1-phenylpyrrolidin-3-yl)pyrimidine-5-carboxamides
- Structure: Incorporates a pyrrolidinone ring fused to the pyrimidine core.
- Synthesis : Achieved via parallel solution-phase synthesis with yields up to 100% .
- Physical Properties :
2-(Phenylthio)pyrimidine-5-carboxylic Acid (3g)
- Structure : Replaces the carboxamide with a carboxylic acid and introduces a thiophenyl group.
- Synthesis: Synthesized via nucleophilic aromatic substitution using thiophenol .
- Properties : The thioether group increases electron density on the pyrimidine ring, which could enhance reactivity in covalent inhibition strategies .
Sulfonyl-Substituted Analogues (e.g., 5-Chloro-2-(propylsulfonyl) Derivatives)
- Structure : Contains a sulfonyl group at the 2-position and halogen substituents.
- Example : N-Benzyl-5-chloro-2-(propylsulfonyl)-N-(2-pyridinyl)pyrimidine-4-carboxamide exhibits strong electron-withdrawing effects, which may improve metabolic stability and target affinity .
Morpholine-Containing Derivatives
- Structure : Substitutes the phenyl group with a morpholine-propyl chain (e.g., N-methyl-N-[2-(morpholin-4-yl)propyl] derivative).
- Properties : The morpholine ring enhances solubility via hydrogen bonding, contrasting with the lipophilic phenylpiperazine derivatives .
Structural and Functional Analysis
Electronic Effects
- Electron-Donating Groups (e.g., Piperazine, Morpholine) : Increase basicity and solubility, favoring interactions with polar enzyme active sites .
- Electron-Withdrawing Groups (e.g., Sulfonyl, Chloro) : Enhance stability and electrophilicity, suitable for covalent inhibition .
Physicochemical Properties
Compound Class | Melting Point Range (°C) | LogP (Predicted) | Solubility Profile |
---|---|---|---|
2-Phenylpiperazine Derivatives | 120–126 | 3.5–4.2 | Moderate (aqueous/organic) |
Pyrrolidinone Derivatives | 122–199 | 2.8–3.5 | Low to moderate |
Sulfonyl Derivatives | N/A | 4.0–5.0 | Low (hydrophobic) |
Biological Activity
2-Phenylpyrimidine-5-carboxamide is a compound that has gained attention in medicinal chemistry due to its diverse biological activities. This article explores its pharmacological properties, mechanisms of action, and potential therapeutic applications, supported by data tables and case studies.
Overview of this compound
This compound, with the chemical formula CHNO, is characterized by a pyrimidine ring substituted with a phenyl group and a carboxamide functional group. Its structural features suggest potential interactions with various biological targets, making it a candidate for multiple therapeutic applications.
Acetylcholinesterase Inhibition
One of the most notable biological activities of this compound is its potential to inhibit acetylcholinesterase (AChE), an enzyme crucial for neurotransmitter regulation. This inhibition can lead to increased levels of acetylcholine in the synaptic cleft, which may have implications for treating neurodegenerative diseases such as Alzheimer's.
Table 1: Acetylcholinesterase Inhibition Activity
Compound | AChE Inhibition IC (µM) |
---|---|
This compound | 12.5 |
Donepezil | 0.1 |
Rivastigmine | 0.05 |
Source: BenchChem
Antimicrobial Activity
Research has indicated that derivatives of 2-phenylpyrimidine compounds exhibit antimicrobial properties. For instance, studies have demonstrated effectiveness against various bacterial strains and fungi.
Table 2: Antimicrobial Activity of Derivatives
Compound | Bacterial Strain | Minimum Inhibitory Concentration (MIC) (µg/mL) |
---|---|---|
A9 (a derivative) | E. coli | 32 |
A9 (a derivative) | S. aureus | 16 |
A9 (a derivative) | C. albicans | 64 |
Molecular Interactions
The mechanism by which this compound exerts its biological effects is primarily through binding interactions with target enzymes and receptors. For AChE inhibition, it is believed that the compound interacts with the active site of the enzyme, preventing substrate hydrolysis.
Case Studies
- Alzheimer's Disease Model : In vitro studies using neuronal cell lines demonstrated that treatment with this compound led to enhanced cell viability in models of oxidative stress, suggesting neuroprotective effects through AChE inhibition and anti-inflammatory pathways.
- Antifungal Activity : A study evaluating the antifungal activity of compound A9 showed significant binding affinity to CYP51, an enzyme involved in ergosterol biosynthesis in fungi. Molecular docking studies revealed that the compound effectively occupies hydrophobic cavities within CYP51, enhancing its antifungal efficacy.
Q & A
Q. Basic: What synthetic routes are reported for 2-phenylpyrimidine-5-carboxamide derivatives?
A multi-step synthesis is commonly employed, involving:
- Step 1 : Condensation of substituted phenylpiperazine with pyrimidine precursors under reflux using acetonitrile or DMF as solvents.
- Step 2 : Carboxamide formation via coupling reactions (e.g., EDC/HOBt-mediated) with methylamine or morpholine derivatives.
- Step 3 : Purification via column chromatography (silica gel, eluent: CH₂Cl₂/MeOH) and characterization by ESI-MS, IR (C=O stretch at ~1620 cm⁻¹), and ¹H/¹³C NMR .
Q. Basic: How are these compounds characterized for structural validation?
Key analytical methods include:
- Mass Spectrometry : ESI-MS confirms molecular ions (e.g., [M+H]+ at 758.2904 for derivative 6l) .
- Vibrational Spectroscopy : IR identifies carbonyl stretches (1621 cm⁻¹ for carboxamide, 1592 cm⁻¹ for acetyl groups) .
- NMR : ¹³C NMR signals at δ 125–114 ppm for aromatic carbons and δ 51–43 ppm for piperazine/morpholine moieties .
Q. Basic: What in vitro assays evaluate acetylcholinesterase (AChE) inhibition?
- Ellman’s Assay : Measures AChE activity via hydrolysis of acetylthiocholine, producing 5-thio-2-nitrobenzoate (λ = 412 nm). IC₅₀ values are calculated for derivatives (e.g., 0.8–12.4 μM) .
- Kinetic Analysis : Determines inhibition type (competitive/uncompetitive) using Lineweaver-Burk plots and Kᵢ values .
Q. Advanced: How do substituents on the phenylpiperazine moiety affect AChE inhibition?
- Electron-Withdrawing Groups (e.g., nitro, acetyl): Enhance binding via hydrophobic interactions with AChE’s peripheral anionic site (PAS). Derivatives with 4-acetyl-2-nitrophenyl groups show IC₅₀ = 0.8 μM .
- Steric Effects : Bulky substituents (e.g., 3-(4-nitrophenoxy)propyl) reduce activity due to steric clashes in the enzyme’s active site gorge .
Q. Advanced: How to resolve contradictions between in vitro activity and molecular docking predictions?
- Case Study : A derivative with high predicted binding energy (ΔG = −9.2 kcal/mol) but moderate IC₅₀ (8.3 μM) may exhibit poor solubility or off-target interactions.
- Mitigation Strategies :
Q. Advanced: What strategies optimize bioavailability of this compound derivatives?
- LogP Reduction : Introduce polar groups (e.g., morpholine, methylpiperazine) to lower cLogP from ~3.5 to <2.5, improving aqueous solubility .
- Metabolic Stability : Assess cytochrome P450 inhibition (CYP3A4/2D6) using liver microsomes. Derivatives with N-methylation show t₁/₂ > 60 min .
Q. Advanced: How to design derivatives targeting dual AChE and amyloid-beta (Aβ) aggregation?
- Bifunctional Ligands : Incorporate:
- AChE-binding motif : this compound core.
- Aβ-binding motif : Diphenyl ether or curcumin-like fragments.
- Validation : Thioflavin T assay for Aβ aggregation inhibition (IC₅₀ < 10 μM) and dual-target molecular dynamics simulations .
Q. Advanced: What computational methods predict toxicity and off-target effects?
Properties
IUPAC Name |
2-phenylpyrimidine-5-carboxamide | |
---|---|---|
Source | PubChem | |
URL | https://pubchem.ncbi.nlm.nih.gov | |
Description | Data deposited in or computed by PubChem | |
InChI |
InChI=1S/C11H9N3O/c12-10(15)9-6-13-11(14-7-9)8-4-2-1-3-5-8/h1-7H,(H2,12,15) | |
Source | PubChem | |
URL | https://pubchem.ncbi.nlm.nih.gov | |
Description | Data deposited in or computed by PubChem | |
InChI Key |
FSBIAEYLUOBHLG-UHFFFAOYSA-N | |
Source | PubChem | |
URL | https://pubchem.ncbi.nlm.nih.gov | |
Description | Data deposited in or computed by PubChem | |
Canonical SMILES |
C1=CC=C(C=C1)C2=NC=C(C=N2)C(=O)N | |
Source | PubChem | |
URL | https://pubchem.ncbi.nlm.nih.gov | |
Description | Data deposited in or computed by PubChem | |
Molecular Formula |
C11H9N3O | |
Source | PubChem | |
URL | https://pubchem.ncbi.nlm.nih.gov | |
Description | Data deposited in or computed by PubChem | |
DSSTOX Substance ID |
DTXSID80554091 | |
Record name | 2-Phenylpyrimidine-5-carboxamide | |
Source | EPA DSSTox | |
URL | https://comptox.epa.gov/dashboard/DTXSID80554091 | |
Description | DSSTox provides a high quality public chemistry resource for supporting improved predictive toxicology. | |
Molecular Weight |
199.21 g/mol | |
Source | PubChem | |
URL | https://pubchem.ncbi.nlm.nih.gov | |
Description | Data deposited in or computed by PubChem | |
CAS No. |
122773-96-0 | |
Record name | 2-Phenylpyrimidine-5-carboxamide | |
Source | EPA DSSTox | |
URL | https://comptox.epa.gov/dashboard/DTXSID80554091 | |
Description | DSSTox provides a high quality public chemistry resource for supporting improved predictive toxicology. | |
Retrosynthesis Analysis
AI-Powered Synthesis Planning: Our tool employs the Template_relevance Pistachio, Template_relevance Bkms_metabolic, Template_relevance Pistachio_ringbreaker, Template_relevance Reaxys, Template_relevance Reaxys_biocatalysis model, leveraging a vast database of chemical reactions to predict feasible synthetic routes.
One-Step Synthesis Focus: Specifically designed for one-step synthesis, it provides concise and direct routes for your target compounds, streamlining the synthesis process.
Accurate Predictions: Utilizing the extensive PISTACHIO, BKMS_METABOLIC, PISTACHIO_RINGBREAKER, REAXYS, REAXYS_BIOCATALYSIS database, our tool offers high-accuracy predictions, reflecting the latest in chemical research and data.
Strategy Settings
Precursor scoring | Relevance Heuristic |
---|---|
Min. plausibility | 0.01 |
Model | Template_relevance |
Template Set | Pistachio/Bkms_metabolic/Pistachio_ringbreaker/Reaxys/Reaxys_biocatalysis |
Top-N result to add to graph | 6 |
Feasible Synthetic Routes
Disclaimer and Information on In-Vitro Research Products
Please be aware that all articles and product information presented on BenchChem are intended solely for informational purposes. The products available for purchase on BenchChem are specifically designed for in-vitro studies, which are conducted outside of living organisms. In-vitro studies, derived from the Latin term "in glass," involve experiments performed in controlled laboratory settings using cells or tissues. It is important to note that these products are not categorized as medicines or drugs, and they have not received approval from the FDA for the prevention, treatment, or cure of any medical condition, ailment, or disease. We must emphasize that any form of bodily introduction of these products into humans or animals is strictly prohibited by law. It is essential to adhere to these guidelines to ensure compliance with legal and ethical standards in research and experimentation.