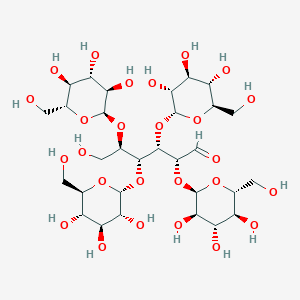
Isomaltopentaose
Overview
Description
Synthesis Analysis
The synthesis of isomaltopentaose and other IMOs has been achieved using various microbial enzymes. One method involves the cell-bound α-glucosidase from a novel strain of Microbacterium sp., utilizing maltose as a glycosyl donor. This process achieved a yield of 85 g/l at a 40% initial substrate concentration, with a notable increase in yield upon the addition of LiCl. The products, including isomaltopentaose, were analyzed using electrospray ionization mass spectrometry and confirmed by NMR spectroscopy (Ojha, Mishra, & Chand, 2015). Another approach employed a thermostable α-glucosidase from Geobacillus sp. strain HTA-462, expressed in Escherichia coli, for IMO synthesis, showing potential for industrial applications due to its efficiency and stability (Zhang et al., 2019).
Scientific Research Applications
Application 1: Functional Food Ingredient
- Summary of the Application : Isomaltooligosaccharides (IMOs), including Isomaltopentaose, are used as functional food ingredients . They serve as alternate sweeteners and are composed of α- (1→6) and α- (1→4)-linked glucose oligomers . They are synthesized by an enzymatic reaction from starch (corn, tapioca) .
- Methods of Application : IMOs are added to food products during the manufacturing process as a sweetener . The specific amount and method of addition would depend on the particular food product being manufactured.
- Results or Outcomes : IMOs are partially indigestible and dietary IMOs stimulate beneficial members of intestinal microbiota, including lactobacilli and bifidobacteria . This makes them a valuable addition to functional foods .
Application 2: Metabolism by Lactobacillus reuteri and Bifidobacteria
- Summary of the Application : Isomaltooligosaccharides, including Isomaltopentaose, are metabolized by certain bacteria, such as Lactobacillus reuteri and bifidobacteria . This metabolism is of interest in the study of gut microbiota and its impact on health .
- Methods of Application : In a laboratory setting, these bacteria are exposed to IMOs and their metabolic activity is studied . The specific methods would depend on the particular experimental design.
- Results or Outcomes : Lactobacilli metabolize isomaltose preferentially over oligosaccharides with higher degree of polymerization (DP). Bifidobacteria preferentially metabolize oligosaccharides with higher DP and accumulate glucose . This metabolism of IMO by lactobacilli is limited to short-chain oligosaccharides, while bifidobacteria preferentially metabolize oligosaccharides with higher DP .
Application 3: Glycemic Response Study
- Summary of the Application : Isomaltooligosaccharides (IMOs), including Isomaltopentaose, have been studied for their impact on glycemic response in healthy adults .
- Methods of Application : In a randomized, double-blind, placebo-controlled, cross-over human study, participants’ glycemic and insulinemic response to IMOs were measured . The IMO syrup dose was matched for 50 g of total carbohydrates and was consumed by mixing in water .
- Results or Outcomes : The study found no significant difference in glycemic response between the control and IMO . This demonstrates that IMO is digestible and can be used to replace sugars in product formulations .
Application 4: Prebiotic Properties
- Summary of the Application : Isomaltooligosaccharides, including Isomaltopentaose, have been studied for their prebiotic properties .
- Methods of Application : In a laboratory setting, the prebiotic properties of IMOs are studied by observing their impact on the growth and activity of beneficial gut bacteria .
- Results or Outcomes : The study found that IMOs can stimulate the growth of beneficial gut bacteria, demonstrating their potential as a prebiotic .
Safety And Hazards
The safety data sheet for Isomaltopentaose suggests avoiding dust formation, breathing mist, gas or vapours, and contacting with skin and eye. It is advised to use personal protective equipment, wear chemical impermeable gloves, ensure adequate ventilation, remove all sources of ignition, evacuate personnel to safe areas, and keep people away from and upwind of spill/leak .
Future Directions
The high-degree polymerization of isomaltooligosaccharide (IMO) not only effectively promotes the growth and reproduction of Bifidobacterium in the human body but also renders it resistant to rapid degradation by gastric acid and can stimulate insulin secretion . The findings provide an important theoretical basis for the future marketable production of IMO4 and analysis of the structure of dextranase .
properties
IUPAC Name |
(2R,3S,4R,5R)-6-hydroxy-2,3,4,5-tetrakis[[(2R,3R,4S,5S,6R)-3,4,5-trihydroxy-6-(hydroxymethyl)oxan-2-yl]oxy]hexanal | |
---|---|---|
Source | PubChem | |
URL | https://pubchem.ncbi.nlm.nih.gov | |
Description | Data deposited in or computed by PubChem | |
InChI |
InChI=1S/C30H52O26/c31-1-7-13(37)17(41)21(45)27(49-7)53-11(5-35)25(55-29-23(47)19(43)15(39)9(3-33)51-29)26(56-30-24(48)20(44)16(40)10(4-34)52-30)12(6-36)54-28-22(46)18(42)14(38)8(2-32)50-28/h5,7-34,36-48H,1-4,6H2/t7-,8-,9-,10-,11+,12-,13-,14-,15-,16-,17+,18+,19+,20+,21-,22-,23-,24-,25-,26-,27-,28-,29-,30-/m1/s1 | |
Source | PubChem | |
URL | https://pubchem.ncbi.nlm.nih.gov | |
Description | Data deposited in or computed by PubChem | |
InChI Key |
ZFGVMMVMFNPHAQ-XAKZEXHGSA-N | |
Source | PubChem | |
URL | https://pubchem.ncbi.nlm.nih.gov | |
Description | Data deposited in or computed by PubChem | |
Canonical SMILES |
C(C1C(C(C(C(O1)OC(CO)C(C(C(C=O)OC2C(C(C(C(O2)CO)O)O)O)OC3C(C(C(C(O3)CO)O)O)O)OC4C(C(C(C(O4)CO)O)O)O)O)O)O)O | |
Source | PubChem | |
URL | https://pubchem.ncbi.nlm.nih.gov | |
Description | Data deposited in or computed by PubChem | |
Isomeric SMILES |
C([C@@H]1[C@H]([C@@H]([C@H]([C@H](O1)O[C@H](CO)[C@H]([C@@H]([C@H](C=O)O[C@@H]2[C@@H]([C@H]([C@@H]([C@H](O2)CO)O)O)O)O[C@@H]3[C@@H]([C@H]([C@@H]([C@H](O3)CO)O)O)O)O[C@@H]4[C@@H]([C@H]([C@@H]([C@H](O4)CO)O)O)O)O)O)O)O | |
Source | PubChem | |
URL | https://pubchem.ncbi.nlm.nih.gov | |
Description | Data deposited in or computed by PubChem | |
Molecular Formula |
C30H52O26 | |
Source | PubChem | |
URL | https://pubchem.ncbi.nlm.nih.gov | |
Description | Data deposited in or computed by PubChem | |
DSSTOX Substance ID |
DTXSID90209661 | |
Record name | Isomaltopentaose | |
Source | EPA DSSTox | |
URL | https://comptox.epa.gov/dashboard/DTXSID90209661 | |
Description | DSSTox provides a high quality public chemistry resource for supporting improved predictive toxicology. | |
Molecular Weight |
828.7 g/mol | |
Source | PubChem | |
URL | https://pubchem.ncbi.nlm.nih.gov | |
Description | Data deposited in or computed by PubChem | |
Product Name |
(2R,3S,4R,5R)-6-hydroxy-2,3,4,5-tetrakis[[(2R,3R,4S,5S,6R)-3,4,5-trihydroxy-6-(hydroxymethyl)oxan-2-yl]oxy]hexanal | |
CAS RN |
6082-32-2 | |
Record name | Isomaltopentaose | |
Source | EPA DSSTox | |
URL | https://comptox.epa.gov/dashboard/DTXSID90209661 | |
Description | DSSTox provides a high quality public chemistry resource for supporting improved predictive toxicology. | |
Citations
Disclaimer and Information on In-Vitro Research Products
Please be aware that all articles and product information presented on BenchChem are intended solely for informational purposes. The products available for purchase on BenchChem are specifically designed for in-vitro studies, which are conducted outside of living organisms. In-vitro studies, derived from the Latin term "in glass," involve experiments performed in controlled laboratory settings using cells or tissues. It is important to note that these products are not categorized as medicines or drugs, and they have not received approval from the FDA for the prevention, treatment, or cure of any medical condition, ailment, or disease. We must emphasize that any form of bodily introduction of these products into humans or animals is strictly prohibited by law. It is essential to adhere to these guidelines to ensure compliance with legal and ethical standards in research and experimentation.