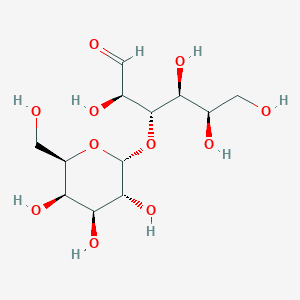
D-Galactose, 3-O-alpha-D-galactopyranosyl-
Overview
Description
D-Galactose, 3-O-alpha-D-galactopyranosyl- (3-O-α-D-galactosyl-D-galactose) is a disaccharide derivative where an α-D-galactopyranosyl group is linked via an α-1,3 glycosidic bond to the hydroxyl group at the C3 position of D-galactose. This compound is structurally related to other galactose-containing oligosaccharides but differs in linkage position and stereochemistry. While D-galactose itself is a fundamental monosaccharide in lactose and glycoconjugates , its 3-O-α-linked derivative plays specialized roles in glycobiology, particularly in studies of carbohydrate-protein interactions and enzymatic specificity .
Preparation Methods
Chemical Synthesis Approaches
Chemical synthesis remains the cornerstone for producing D-galactose, 3-O-α-D-galactopyranosyl-, with methodologies focusing on glycosylation efficiency and stereochemical control.
Glycosylation Using Trichloroacetimidate Donors
Trichloroacetimidate derivatives are widely employed as glycosyl donors due to their high reactivity and stereoselectivity. For instance, 2,3,4,6-tetra-O-benzyl-α-D-galactopyranosyl trichloroacetimidate, when activated by trimethylsilyl triflate (TMSOTf), facilitates α-selective glycosylation with acceptors such as 1,2:5,6-di-O-cyclohexylidene-α-D-galactofuranose . This method yields protected disaccharides with α-(1→3) linkages, which are subsequently deprotected via hydrogenolysis (for benzyl groups) and acid hydrolysis (for cyclohexylidene groups) . The reaction typically proceeds in anhydrous dichloromethane at −20°C to room temperature, achieving yields of 65–75% .
Thioglycoside-Based Glycosylation
Phenyl thioglycosides offer stability and compatibility with diverse reaction conditions. In one approach, phenyl 2,3,4,6-tetra-O-benzyl-1-thio-β-D-galactopyranoside was coupled with 1,2:5,6-di-O-cyclohexylidene-α-D-galactofuranose using N-iodosuccinimide (NIS) and triflic acid (TfOH) as promoters . This method favors α-anomer formation through neighboring group participation, with yields comparable to trichloroacetimidate-based routes (70–78%) .
Halide Donors in Stereoselective Synthesis
Galactopyranosyl bromides, such as 2,3,4,6-tetra-O-benzyl-α-D-galactopyranosyl bromide, have been utilized in Koenigs-Knorr reactions. Activation with silver triflate (AgOTf) in the presence of molecular sieves enables glycosylation of acceptors like allyl 2,6-di-O-benzyl-α-D-galactopyranoside . The reaction proceeds in dichloromethane at 0°C, yielding 60–68% of the α-(1→3)-linked product after column chromatography .
Enzymatic Synthesis Strategies
Enzymatic methods provide an alternative to chemical synthesis, leveraging the specificity of glycosidases and glycosyltransferases.
β-Galactosidase-Catalyzed Transglycosylation
β-Galactosidase from Bacillus circulans catalyzes transglycosylation using β-D-galactopyranosyl-p-nitrophenol (β-D-Gal-pNP) as a donor and D-galactose as an acceptor . Notably, this enzyme exhibits dual regioselectivity, producing both β-(1→3)- and β-(1→6)-linked disaccharides. However, when D-GlcNAc or D-GalNAc serves as the acceptor, β-(1→3) linkage predominates (95:5 ratio) . Reaction optimization at pH 6.0 and 37°C yields 40–50% conversion, with purification via HPLC on a Carbopac PA1 column .
Glycosyltransferase-Mediated Synthesis
While glycosyltransferases are less commonly reported for α-(1→3) linkages, recombinant α-1,3-galactosyltransferases have been explored. These enzymes utilize UDP-galactose as a donor, transferring the galactosyl moiety to C3-OH of acceptor substrates. Although yields are modest (30–40%), this method avoids protective group manipulations .
Purification and Characterization
Chromatographic Techniques
Purification challenges arise from regioisomer separation and residual substrate removal. High-performance liquid chromatography (HPLC) with hydrophilic interaction liquid chromatography (HILIC) or C18 columns effectively resolves α-(1→3) and α-(1→6) isomers . Gradient elution (acetonitrile/water) with detection at 190–210 nm optimizes carbohydrate visibility .
Spectroscopic Confirmation of Structure
Nuclear magnetic resonance (NMR) spectroscopy is indispensable for confirming the α-(1→3) linkage. Key diagnostic signals include:
-
¹³C-NMR : Glycosidic carbon (C1) at δ 95–100 ppm and C3 of the acceptor galactose at δ 75–78 ppm .
Mass spectrometry (MALDI-TOF) further validates molecular weight, with [M+Na]⁺ peaks at m/z 365.1 for the disaccharide .
Comparative Analysis of Synthetic Routes
Chemical Reactions Analysis
Types of Reactions: D-Galactose, 3-O-alpha-D-galactopyranosyl- undergoes various chemical reactions, including:
Oxidation: This reaction can convert the hydroxyl groups to carbonyl groups, forming aldehydes or ketones.
Reduction: Reduction reactions can convert carbonyl groups back to hydroxyl groups.
Substitution: The hydroxyl groups can be substituted with other functional groups, such as acetyl or methyl groups.
Common Reagents and Conditions:
Oxidation: Reagents like periodic acid (HIO4) or bromine water (Br2/H2O) are commonly used.
Reduction: Sodium borohydride (NaBH4) or lithium aluminum hydride (LiAlH4) are typical reducing agents.
Substitution: Acetylation can be performed using acetic anhydride (Ac2O) in the presence of a base like pyridine.
Major Products:
Oxidation: Produces galactaric acid or galactonic acid.
Reduction: Yields galactitol.
Substitution: Forms acetylated or methylated derivatives.
Scientific Research Applications
Biochemical Applications
1.1. Glycobiology and Cell Signaling
D-Galactose derivatives play a crucial role in glycobiology, particularly in cell signaling pathways. The presence of galactose residues in glycoproteins and glycolipids influences cellular interactions and recognition processes. For instance, the incorporation of D-galactose into glycan structures can enhance the binding affinity of lectins, which are proteins that recognize specific carbohydrate moieties on cell surfaces .
1.2. Synthesis of Glycoconjugates
The compound is utilized in synthesizing glycoconjugates, which are essential for studying protein-carbohydrate interactions. Recent advancements have demonstrated that D-Galactose derivatives can be used to create complex glycosylated structures that mimic natural glycans, aiding in the investigation of biological functions and disease mechanisms .
Pharmaceutical Applications
2.1. Drug Development
D-Galactose, 3-O-alpha-D-galactopyranosyl- has been explored in drug formulation due to its potential immunomodulatory effects. Research indicates that galactosylated compounds can enhance the immune response by targeting specific receptors on immune cells, making them promising candidates for vaccine development and immunotherapy .
2.2. Antiviral Agents
Studies have shown that certain galactosylated compounds exhibit antiviral properties by inhibiting viral entry into host cells. For example, modifications involving D-galactose have been investigated as potential treatments for viral infections like HIV and influenza, where they interfere with viral glycoprotein interactions .
Food Science Applications
3.1. Prebiotic Effects
D-Galactose oligosaccharides are recognized for their prebiotic properties, promoting beneficial gut microbiota growth. The consumption of foods enriched with D-galactose can enhance gut health by selectively stimulating the growth of probiotics such as Bifidobacteria and Lactobacillus species .
3.2. Flavor Enhancement
In the food industry, D-Galactose derivatives are utilized to improve the flavor profile of various products. Their sweet taste can enhance the palatability of low-calorie foods and beverages without significantly increasing caloric content .
Case Studies
Mechanism of Action
The mechanism of action of D-Galactose, 3-O-alpha-D-galactopyranosyl- involves its interaction with specific enzymes and receptors in biological systems. It acts as a substrate for galactosidases, which hydrolyze the glycosidic bond to release free galactose molecules . These galactose molecules can then enter metabolic pathways such as the Leloir pathway, where they are converted to glucose-1-phosphate and subsequently utilized for energy production .
Comparison with Similar Compounds
Structural and Functional Comparison with Similar Compounds
Table 1: Key Structural and Functional Differences
Key Insights:
Linkage Position :
- The α-1,3 linkage in 3-O-α-D-galactosyl-D-galactose distinguishes it from the α-1,4-linked analog (4-O-α-D-galactosyl-D-galactose). This difference impacts enzymatic recognition; for example, α-galactosidases and galactosyltransferases exhibit specificity toward distinct linkage positions .
- highlights that 4-O-α-linked galactose is used to study galactosyltransferases, suggesting that the 3-O-α variant may interact with different isoforms or exhibit altered binding kinetics.
Functional Groups: Unlike acetylated derivatives (e.g., D-galactose pentaacetate), the 3-O-α-linked compound retains free hydroxyl groups, making it more hydrophilic and suitable for aqueous enzymatic assays . The absence of acetyl or acetylamino groups (as seen in and ) may render 3-O-α-D-galactosyl-D-galactose more susceptible to hydrolysis by glycosidases compared to its acetylated counterparts .
Biological Applications :
- While 4-O-α-D-galactosyl-D-galactose is utilized in lectin-binding studies and carbohydrate microarrays , the 3-O-α-linked derivative could serve as a substrate for α-1,3-specific galactosyltransferases involved in synthesizing blood group antigens or microbial glycans.
- D-Galactose pentaacetate, with enhanced stability, is preferred in synthetic chemistry for constructing glycoconjugates , whereas the 3-O-α-linked compound may be more relevant in native biochemical contexts.
Research Findings and Mechanistic Insights
Enzymatic Interactions: α-Galactosidases, which hydrolyze α-linked galactose residues, show varying tolerance to D-galactose derivatives. notes that α-galactosidase from Streptomyces griseoloalbus tolerates D-galactose up to 100 mM, but the inhibitory effect on the 3-O-α-linked compound remains unstudied. The α-1,3 linkage may confer resistance to certain α-galactosidases, analogous to how β-1,4 linkages in lactose require specific β-galactosidases for cleavage .
Metabolic Pathways :
- D-Galactose metabolism typically occurs via the Leloir pathway, but alternative routes exist, such as the conversion to L-sorbose in Aspergillus nidulans . The 3-O-α-linked derivative may bypass standard pathways, requiring specialized enzymes for catabolism.
Pharmacological Potential: While D-galactose exhibits anti-inflammatory effects in atopic dermatitis models (e.g., reduced IgE levels in mice; ), the 3-O-α-linked compound’s bioactivity is unexplored in the provided evidence.
Biological Activity
D-Galactose, specifically in its 3-O-alpha-D-galactopyranosyl form, is a disaccharide that has garnered attention for its biological activities and potential applications in various fields, including nutrition and immunology. This article provides a detailed overview of the biological activity associated with this compound, supported by data tables, case studies, and research findings.
Chemical Structure and Properties
D-Galactose, 3-O-alpha-D-galactopyranosyl- is a disaccharide composed of two galactose units linked by an alpha (1→3) glycosidic bond. This structure influences its biological functions, particularly in immunological responses.
Biological Functions
1. Immunomodulatory Effects
Research indicates that D-Galactose derivatives can stimulate immune responses. The α-gal epitope, which is present in this compound, is known to elicit strong immune reactions due to its recognition by natural antibodies in humans. This has implications for vaccine development and cancer therapies, where enhancing immune responses is critical.
2. Nutritional Benefits
Studies have shown that oligosaccharides containing D-Galactose can serve as prebiotics, promoting the growth of beneficial gut bacteria. This prebiotic effect contributes to improved gut health and overall well-being.
Case Studies
-
Case Study 1: Immunogenicity of α-Gal Epitopes
A study explored the immunogenicity of α-Gal epitopes derived from D-Galactose in a vaccine context. The findings revealed that these epitopes could enhance antibody production against specific pathogens, indicating their potential use in vaccine formulations aimed at improving immune responses against infections . -
Case Study 2: Prebiotic Effects
Another investigation assessed the prebiotic effects of D-Galactose-containing oligosaccharides on gut microbiota composition in humans. Results showed a significant increase in beneficial bacteria such as Bifidobacteria and Lactobacilli, highlighting the compound's role in promoting gut health .
Table 1: Summary of Biological Activities of D-Galactose Derivatives
The biological activity of D-Galactose is largely attributed to its structural characteristics that facilitate interactions with immune cells. The α-gal epitope binds to receptors on immune cells, triggering signaling pathways that lead to enhanced immune responses. This mechanism underlies both its immunomodulatory effects and its potential as a prebiotic.
Q & A
Basic Research Questions
Q. Q1. What are the primary mechanisms by which D-galactose induces oxidative stress and advanced glycation end products (AGEs) in experimental models?
D-Galactose undergoes enzymatic oxidation via galactose oxidase, producing reactive oxygen species (ROS) like hydrogen peroxide (H₂O₂) and hydroxyl radicals (OH⁻). These radicals damage cellular structures and initiate glycation reactions, where D-galactose binds to free amino groups of proteins, forming AGEs. AGE accumulation disrupts cellular function and accelerates aging phenotypes. Methodologically, researchers quantify ROS using fluorometric assays (e.g., DCFH-DA) and AGEs via ELISA or fluorescence spectroscopy .
Q. Q2. How is D-galactose utilized to establish aging or neurodegenerative disease models in rodents?
A standardized protocol involves administering D-galactose subcutaneously (300 mg/kg body weight) or orally (100 mg/kg) daily for 6–15 weeks. This induces oxidative stress, mitochondrial dysfunction, and cognitive decline, mimicking natural aging or Alzheimer’s disease. Key biomarkers include elevated thiobarbituric acid reactive substances (TBARS), reduced superoxide dismutase (SOD), and glutathione (GSH). Validation requires behavioral tests (e.g., Morris water maze) and histopathological analysis of brain tissue .
Q. Q3. How do contradictory findings in D-galactose models arise across species (e.g., rodents vs. insects)?
Discrepancies stem from species-specific metabolic rates and antioxidant systems. For example, Drosophila melanogaster exposed to D-galactose show a biphasic SOD activity curve (initial increase followed by decline), whereas rodents exhibit sustained SOD reduction. Methodological adjustments, such as dose scaling (e.g., 200 mM in cell cultures vs. 300 mg/kg in rats) and exposure duration, are critical to reconcile data .
Q. Q4. What experimental strategies mitigate confounding variables in D-galactose-induced glycation studies?
To isolate glycation effects:
- Use galactose oxidase inhibitors (e.g., quercetin) to block ROS production.
- Employ AGE-specific antibodies (e.g., anti-CML) in Western blotting.
- Pair with antioxidant treatments (e.g., melatonin at 10 mg/kg) to dissect oxidative vs. glycation pathways .
Q. Q5. How can glycosidic linkage specificity (e.g., 3-O-α vs. 4-O-β) impact D-galactose’s biological activity?
The 3-O-α-D-galactopyranosyl linkage may alter enzyme recognition (e.g., galactosidases) and metabolic turnover rates compared to 4-O-β derivatives. Structural analysis via NMR or X-ray crystallography is essential to map binding affinities. For example, β-linked galactose in lactose (4-O-β-D-galactopyranosyl-D-glucose) is hydrolyzed by lactase, whereas α-linked forms resist digestion, influencing bioavailability .
Q. Methodological Challenges
Q. Q6. What are the limitations of using D-galactose to model age-related diseases in vitro?
- Dose Dependency: High concentrations (>200 mM) in cell cultures (e.g., SH-SY5Y neurons) may induce non-physiological osmotic stress.
- Time Course: Acute exposure (48 hours) primarily triggers ROS, while chronic models (>8 weeks) better replicate AGE accumulation.
- Validation: Combine with genetic models (e.g., APP/PS1 mice for Alzheimer’s) to confirm pathological relevance .
Q. Q7. How can researchers optimize D-galactose administration routes for tissue-specific effects?
- Subcutaneous Injection: Ensures systemic distribution, ideal for whole-body aging phenotypes.
- Oral Gavage: Mimics dietary intake but varies in bioavailability due to gut microbiota metabolism.
- Intraperitoneal Delivery: Achieves rapid plasma peaks but risks localized inflammation .
Q. Emerging Directions
Q. Q8. What novel biotechnological applications leverage D-galactose’s structural properties?
Properties
IUPAC Name |
(2R,3S,4S,5R)-2,4,5,6-tetrahydroxy-3-[(2R,3R,4S,5R,6R)-3,4,5-trihydroxy-6-(hydroxymethyl)oxan-2-yl]oxyhexanal | |
---|---|---|
Source | PubChem | |
URL | https://pubchem.ncbi.nlm.nih.gov | |
Description | Data deposited in or computed by PubChem | |
InChI |
InChI=1S/C12H22O11/c13-1-4(16)7(18)11(5(17)2-14)23-12-10(21)9(20)8(19)6(3-15)22-12/h2,4-13,15-21H,1,3H2/t4-,5+,6-,7+,8+,9+,10-,11-,12-/m1/s1 | |
Source | PubChem | |
URL | https://pubchem.ncbi.nlm.nih.gov | |
Description | Data deposited in or computed by PubChem | |
InChI Key |
YGEHCIVVZVBCLE-FSYGUOKUSA-N | |
Source | PubChem | |
URL | https://pubchem.ncbi.nlm.nih.gov | |
Description | Data deposited in or computed by PubChem | |
Canonical SMILES |
C(C1C(C(C(C(O1)OC(C(C=O)O)C(C(CO)O)O)O)O)O)O | |
Source | PubChem | |
URL | https://pubchem.ncbi.nlm.nih.gov | |
Description | Data deposited in or computed by PubChem | |
Isomeric SMILES |
C([C@@H]1[C@@H]([C@@H]([C@H]([C@H](O1)O[C@H]([C@H](C=O)O)[C@H]([C@@H](CO)O)O)O)O)O)O | |
Source | PubChem | |
URL | https://pubchem.ncbi.nlm.nih.gov | |
Description | Data deposited in or computed by PubChem | |
Molecular Formula |
C12H22O11 | |
Source | PubChem | |
URL | https://pubchem.ncbi.nlm.nih.gov | |
Description | Data deposited in or computed by PubChem | |
DSSTOX Substance ID |
DTXSID30927317 | |
Record name | 3-O-alpha-D-Galactopyranosyl-D-galactose | |
Source | EPA DSSTox | |
URL | https://comptox.epa.gov/dashboard/DTXSID30927317 | |
Description | DSSTox provides a high quality public chemistry resource for supporting improved predictive toxicology. | |
Molecular Weight |
342.30 g/mol | |
Source | PubChem | |
URL | https://pubchem.ncbi.nlm.nih.gov | |
Description | Data deposited in or computed by PubChem | |
CAS No. |
13168-24-6 | |
Record name | Galactosyl-(1-3)galactose | |
Source | ChemIDplus | |
URL | https://pubchem.ncbi.nlm.nih.gov/substance/?source=chemidplus&sourceid=0013168246 | |
Description | ChemIDplus is a free, web search system that provides access to the structure and nomenclature authority files used for the identification of chemical substances cited in National Library of Medicine (NLM) databases, including the TOXNET system. | |
Record name | 3-O-alpha-D-Galactopyranosyl-D-galactose | |
Source | EPA DSSTox | |
URL | https://comptox.epa.gov/dashboard/DTXSID30927317 | |
Description | DSSTox provides a high quality public chemistry resource for supporting improved predictive toxicology. | |
Record name | GALACTOBIOSE, 3.ALPHA.- | |
Source | FDA Global Substance Registration System (GSRS) | |
URL | https://gsrs.ncats.nih.gov/ginas/app/beta/substances/XW90KQ841P | |
Description | The FDA Global Substance Registration System (GSRS) enables the efficient and accurate exchange of information on what substances are in regulated products. Instead of relying on names, which vary across regulatory domains, countries, and regions, the GSRS knowledge base makes it possible for substances to be defined by standardized, scientific descriptions. | |
Explanation | Unless otherwise noted, the contents of the FDA website (www.fda.gov), both text and graphics, are not copyrighted. They are in the public domain and may be republished, reprinted and otherwise used freely by anyone without the need to obtain permission from FDA. Credit to the U.S. Food and Drug Administration as the source is appreciated but not required. | |
Disclaimer and Information on In-Vitro Research Products
Please be aware that all articles and product information presented on BenchChem are intended solely for informational purposes. The products available for purchase on BenchChem are specifically designed for in-vitro studies, which are conducted outside of living organisms. In-vitro studies, derived from the Latin term "in glass," involve experiments performed in controlled laboratory settings using cells or tissues. It is important to note that these products are not categorized as medicines or drugs, and they have not received approval from the FDA for the prevention, treatment, or cure of any medical condition, ailment, or disease. We must emphasize that any form of bodily introduction of these products into humans or animals is strictly prohibited by law. It is essential to adhere to these guidelines to ensure compliance with legal and ethical standards in research and experimentation.