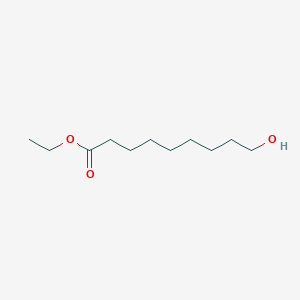
8-ETHOXYCARBONYLOCTANOL
Overview
Description
8-Ethoxycarbonyloctanol (CAS: 3639-34-7), also known as ethyl 9-hydroxynonanoate, is a branched ester-alcohol compound with the molecular formula C₁₁H₂₂O₃. Its structure comprises an eight-carbon chain terminated by a hydroxyl (-OH) group and an ethoxycarbonyl (-OCO₂C₂H₅) moiety at the terminal position. This dual functionalization enables unique reactivity, making it a versatile intermediate in organic synthesis. Applications include its use as a precursor in the production of plasticizers, surfactants, and specialty polymers . Its ester group facilitates hydrolysis to carboxylic acids, while the hydroxyl group allows for further derivatization, such as etherification or esterification.
Preparation Methods
Catalytic Glycosylation Using 8-Ethoxycarbonyloctanol
Stannic Tetrachloride-Mediated β-Glycoside Formation
This compound serves as a glycosyl acceptor in stannic tetrachloride (SnCl₄)-catalyzed reactions with disaccharide octaacetates, such as cellobiose, lactose, and maltose . The process involves generating a 1,2-acetoxonium ion intermediate at −10°C in dichloromethane, which facilitates β-glycosidic bond formation via 1,2-orthoacetate intermediates . For example, reacting trans-cellobiose octaacetate with this compound yields β-glycosides in >70% efficiency .
Reaction Conditions:
-
Temperature: −10°C to 25°C
-
Catalyst: SnCl₄ (5–10 mol%)
-
Solvent: Dichloromethane
-
Duration: 4–24 hours
Post-reaction anomerization at ambient temperature converts β-glycosides to α-anomers, enabling access to both stereoisomers .
Applications in Glycoconjugate Synthesis
Lewis-Y Tetrasaccharide Derivatives
This compound is functionalized as a glycoside in the synthesis of Lewis-Y (Le^Y) tetrasaccharides, which mimic tumor-associated antigens . The trichloroacetimidate method enables regioselective β-glycosylation under SN2 conditions, achieving >60% yields for Le^Y-glycoside conjugates .
Key Steps:
-
Protection: Dimethyl-(2,3-dimethyl-2-butyl)silyl groups shield hydroxyl moieties.
-
Glycosylation: Trichloroacetimidate donors react with this compound.
-
Deprotection: Fluoride-based cleavage removes silyl groups.
Chemical Reactions Analysis
Types of Reactions: D-(+)-Cellobiose undergoes various chemical reactions, including:
Oxidation: D-(+)-Cellobiose can be oxidized to form cellobionic acid.
Reduction: It can be reduced to form cellobiitol.
Hydrolysis: D-(+)-Cellobiose can be hydrolyzed to yield two molecules of D-glucose.
Common Reagents and Conditions:
Oxidation: Common oxidizing agents include bromine water and nitric acid.
Reduction: Reducing agents such as sodium borohydride are used.
Hydrolysis: Acidic or enzymatic hydrolysis using cellulase enzymes.
Major Products Formed:
Oxidation: Cellobionic acid.
Reduction: Cellobiitol.
Hydrolysis: D-glucose.
Scientific Research Applications
D-(+)-Cellobiose has a wide range of applications in scientific research:
Chemistry: It is used as a model compound to study the hydrolysis of cellulose and the action of cellulase enzymes.
Biology: D-(+)-Cellobiose is used in studies of carbohydrate metabolism and as a substrate for enzyme assays.
Medicine: It is used in diagnostic tests to assess intestinal permeability and malabsorption.
Industry: D-(+)-Cellobiose is used in the production of biofuels and biodegradable materials
Mechanism of Action
D-(+)-Cellobiose exerts its effects primarily through its role as an intermediate in the hydrolysis of cellulose. The β(1→4) glycosidic bond in D-(+)-Cellobiose is cleaved by cellulase enzymes, resulting in the formation of D-glucose. This process is crucial for the conversion of cellulose into fermentable sugars, which can be further utilized in various biochemical pathways .
Comparison with Similar Compounds
Comparative Analysis with Structurally Similar Compounds
Structural Comparison
Table 1: Key Structural Features
Compound Name | CAS Number | Molecular Formula | Functional Groups | Molecular Weight (g/mol) |
---|---|---|---|---|
8-Ethoxycarbonyloctanol | 3639-34-7 | C₁₁H₂₂O₃ | Ester (-OCO₂C₂H₅), Alcohol (-OH) | 202.29 |
1,2-Nonanediol | 42789-13-9 | C₉H₂₀O₂ | Diol (-OH at C1 and C2) | 160.25 |
Key Observations :
- Chain Length: this compound has a longer carbon backbone (C11 vs. C9 in 1,2-nonanediol), contributing to differences in hydrophobicity.
- Functionality: The ester and alcohol groups in this compound contrast with the dual hydroxyl groups in 1,2-nonanediol, leading to divergent reactivity and solubility profiles .
Physicochemical Properties
Table 2: Comparative Physicochemical Data
Property | This compound | 1,2-Nonanediol |
---|---|---|
Water Solubility | Low (ester dominance) | Moderate (polar diol) |
Boiling Point | ~250–270°C (estimated) | ~280–300°C (reported) |
Hydrogen Bonding Capacity | Moderate (1 -OH) | High (2 -OH groups) |
Analysis :
- Solubility: The ester group in this compound reduces water solubility compared to 1,2-nonanediol, which benefits from two hydrophilic hydroxyl groups.
- Boiling Point: 1,2-Nonanediol’s higher boiling point is attributed to stronger intermolecular hydrogen bonding, despite its lower molecular weight .
Reactivity Profiles:
- This compound: Ester Hydrolysis: Reacts with aqueous acids or bases to yield 9-hydroxynonanoic acid and ethanol. Alcohol Derivatization: The -OH group can be oxidized to ketones or esterified for polymer applications.
- 1,2-Nonanediol: Oxidation: Forms ketones or carboxylic acids under strong oxidizing conditions. Crosslinking: Used in polyurethane production due to dual -OH groups.
Biological Activity
8-Ethoxycarbonyloctanol, a compound belonging to the class of fatty alcohols, has garnered attention for its potential biological activities. This article reviews the existing literature on its biological properties, including cytotoxicity, antioxidant effects, and antimicrobial activity.
Chemical Structure and Properties
This compound is characterized by an ethoxycarbonyl group attached to an octanol backbone. This structure may influence its solubility and interaction with biological membranes, which are critical for its biological activity.
1. Antioxidant Activity
Research indicates that compounds with similar structures exhibit significant antioxidant properties. For instance, studies on fatty alcohols have demonstrated their ability to scavenge free radicals in vitro. The DPPH (2,2-diphenyl-1-picrylhydrazyl) assay is commonly used to evaluate the radical scavenging capacity of such compounds.
Compound | IC50 (µg/mL) | Assay Type |
---|---|---|
This compound | TBD | DPPH |
3,7-Dimethyl-1-octanol | 31.25–500 | DPPH |
The antioxidant activity is crucial for preventing oxidative stress-related diseases, suggesting that this compound may possess similar protective effects .
2. Cytotoxicity
Cytotoxic effects of fatty alcohols can vary significantly based on their structure. In studies involving various alcohols, it was observed that while some exhibited low cytotoxicity at concentrations up to 500 µg/mL, others showed significant effects at lower doses. The specific cytotoxic profile of this compound remains to be fully elucidated but warrants further investigation.
3. Antimicrobial Activity
The antimicrobial potential of fatty alcohols has been documented extensively. For example, derivatives of octanol have shown efficacy against a range of microbial strains. The mechanism often involves disruption of microbial cell membranes.
Microbial Strain | Minimum Inhibitory Concentration (MIC) |
---|---|
Staphylococcus aureus | TBD |
Escherichia coli | TBD |
Studies indicate that fatty alcohols can inhibit bacterial growth effectively, suggesting that this compound may also exhibit similar antimicrobial properties .
Case Studies
A notable study explored the effects of various fatty alcohol derivatives on microbial inhibition and cytotoxicity. The findings indicated that structural modifications significantly influenced both the efficacy against pathogens and the safety profile in mammalian cells.
- Case Study 1 : A comparative analysis of octanol derivatives revealed that modifications at the hydroxyl group enhanced antimicrobial activity while maintaining low cytotoxicity levels.
- Case Study 2 : In vitro assays using human cell lines demonstrated that certain fatty alcohols could reduce oxidative stress markers without inducing apoptosis at therapeutic concentrations.
Q & A
Basic Research Questions
Q. What are the recommended methodologies for synthesizing and characterizing 8-ethoxycarbonyloctanol in laboratory settings?
- Answer : Synthesis typically involves esterification of octanol with ethyl chloroformate under controlled conditions (e.g., inert atmosphere, temperature <50°C). Characterization should include NMR (¹H/¹³C) for structural confirmation, HPLC for purity assessment (>98%), and FT-IR to verify functional groups. For known compounds, cross-reference spectral data with established literature . Novel compounds require elemental analysis and high-resolution mass spectrometry (HRMS) to confirm molecular composition .
Q. How should researchers design experiments to assess the compound’s purity and stability under varying storage conditions?
- Answer : Use accelerated stability studies (e.g., ICH guidelines) with parameters like temperature (25°C, 40°C), humidity (60% RH), and light exposure. Monitor degradation via HPLC or GC-MS at intervals (0, 1, 3, 6 months). Include control samples and validate methods per ICH Q2(R1) . For purity, employ Karl Fischer titration for water content and differential scanning calorimetry (DSC) to detect polymorphic changes .
Q. What are the best practices for documenting experimental procedures to ensure reproducibility?
- Answer : Follow journal-specific guidelines (e.g., Beilstein Journal of Organic Chemistry):
- Detail reaction stoichiometry, catalysts, solvents, and purification steps.
- Report yields, melting/boiling points, and spectral data in tabular format.
- For reproducibility, include raw data (e.g., NMR spectra) in supplementary materials and reference them explicitly in the main text .
Advanced Research Questions
Q. How can researchers resolve contradictions in spectroscopic data for this compound derivatives?
- Answer : Cross-validate findings using orthogonal techniques:
- Compare NMR data with computational simulations (e.g., DFT-based chemical shift predictions).
- Use 2D NMR (COSY, HSQC) to resolve overlapping signals.
- If discrepancies persist, reassess synthetic pathways for unintended side reactions (e.g., transesterification) and consult crystallographic data (if available) .
Q. What statistical approaches are suitable for optimizing reaction conditions to maximize yield of this compound?
- Answer : Apply design of experiments (DoE) methodologies:
- Use response surface methodology (RSM) to model variables (temperature, catalyst loading, solvent ratio).
- Validate models via ANOVA and confirm optimal conditions with triplicate runs.
- Tools like JMP or Minitab can automate analysis and identify significant factors .
Q. How should systematic reviews address conflicting evidence on the biological activity of this compound?
- Answer : Follow PRISMA guidelines for systematic reviews:
- Conduct meta-analyses to quantify effect sizes across studies, stratified by experimental models (e.g., in vitro vs. in vivo).
- Assess heterogeneity using I² statistics and explore sources of bias (e.g., publication bias via funnel plots).
- Highlight methodological limitations (e.g., inconsistent dosing regimens) in the discussion .
Q. What strategies are effective for analyzing structure-activity relationships (SAR) of this compound analogs?
- Answer :
- Perform comparative molecular field analysis (CoMFA) or QSAR modeling using descriptors like logP, steric bulk, and electronic parameters.
- Validate models with leave-one-out cross-validation and external test sets.
- Correlate in silico predictions with experimental IC₅₀ values from enzyme inhibition assays .
Methodological and Data Management Questions
Q. How can researchers ensure robust data management for long-term studies involving this compound?
- Answer :
- Use FAIR principles (Findable, Accessible, Interoperable, Reusable): store raw data in repositories like Zenodo or Figshare with persistent DOIs.
- For large datasets (e.g., GC-MS chromatograms), include processed data in appendices and hyperlink to supplementary files .
Q. What ethical considerations are critical when designing toxicity studies for this compound?
- Answer :
- Adhere to OECD test guidelines (e.g., OECD 423 for acute toxicity) and obtain institutional animal care committee (IACUC) approval.
- For human cell line studies, ensure informed consent and compliance with Declaration of Helsinki principles.
- Disclose conflicts of interest and funding sources in the ethics statement .
Q. How can researchers address limitations in detecting low-concentration degradation products of this compound?
- Answer :
- Employ high-sensitivity techniques like LC-QTOF-MS for trace analysis.
- Use isotope-labeled internal standards to improve quantification accuracy.
- Validate limits of detection (LOD) and quantification (LOQ) via calibration curves .
Contradiction and Validation Questions
Q. What steps should be taken if in vitro and in vivo toxicity data for this compound conflict?
- Answer :
- Re-evaluate bioavailability factors (e.g., metabolic activation in vivo vs. static in vitro conditions).
- Conduct pharmacokinetic studies (e.g., AUC, Cmax) to correlate exposure levels with observed effects.
- Use human-relevant models (e.g., 3D organoids) to bridge the gap between assay systems .
Q. How can researchers validate computational predictions of this compound’s physicochemical properties?
Properties
IUPAC Name |
ethyl 9-hydroxynonanoate | |
---|---|---|
Source | PubChem | |
URL | https://pubchem.ncbi.nlm.nih.gov | |
Description | Data deposited in or computed by PubChem | |
InChI |
InChI=1S/C11H22O3/c1-2-14-11(13)9-7-5-3-4-6-8-10-12/h12H,2-10H2,1H3 | |
Source | PubChem | |
URL | https://pubchem.ncbi.nlm.nih.gov | |
Description | Data deposited in or computed by PubChem | |
InChI Key |
MNWRYCGYJOYZEI-UHFFFAOYSA-N | |
Source | PubChem | |
URL | https://pubchem.ncbi.nlm.nih.gov | |
Description | Data deposited in or computed by PubChem | |
Canonical SMILES |
CCOC(=O)CCCCCCCCO | |
Source | PubChem | |
URL | https://pubchem.ncbi.nlm.nih.gov | |
Description | Data deposited in or computed by PubChem | |
Molecular Formula |
C11H22O3 | |
Source | PubChem | |
URL | https://pubchem.ncbi.nlm.nih.gov | |
Description | Data deposited in or computed by PubChem | |
DSSTOX Substance ID |
DTXSID70394221 | |
Record name | Ethyl 9-hydroxynonanoate | |
Source | EPA DSSTox | |
URL | https://comptox.epa.gov/dashboard/DTXSID70394221 | |
Description | DSSTox provides a high quality public chemistry resource for supporting improved predictive toxicology. | |
Molecular Weight |
202.29 g/mol | |
Source | PubChem | |
URL | https://pubchem.ncbi.nlm.nih.gov | |
Description | Data deposited in or computed by PubChem | |
CAS No. |
3639-34-7 | |
Record name | Ethyl 9-hydroxynonanoate | |
Source | EPA DSSTox | |
URL | https://comptox.epa.gov/dashboard/DTXSID70394221 | |
Description | DSSTox provides a high quality public chemistry resource for supporting improved predictive toxicology. | |
Retrosynthesis Analysis
AI-Powered Synthesis Planning: Our tool employs the Template_relevance Pistachio, Template_relevance Bkms_metabolic, Template_relevance Pistachio_ringbreaker, Template_relevance Reaxys, Template_relevance Reaxys_biocatalysis model, leveraging a vast database of chemical reactions to predict feasible synthetic routes.
One-Step Synthesis Focus: Specifically designed for one-step synthesis, it provides concise and direct routes for your target compounds, streamlining the synthesis process.
Accurate Predictions: Utilizing the extensive PISTACHIO, BKMS_METABOLIC, PISTACHIO_RINGBREAKER, REAXYS, REAXYS_BIOCATALYSIS database, our tool offers high-accuracy predictions, reflecting the latest in chemical research and data.
Strategy Settings
Precursor scoring | Relevance Heuristic |
---|---|
Min. plausibility | 0.01 |
Model | Template_relevance |
Template Set | Pistachio/Bkms_metabolic/Pistachio_ringbreaker/Reaxys/Reaxys_biocatalysis |
Top-N result to add to graph | 6 |
Feasible Synthetic Routes
Disclaimer and Information on In-Vitro Research Products
Please be aware that all articles and product information presented on BenchChem are intended solely for informational purposes. The products available for purchase on BenchChem are specifically designed for in-vitro studies, which are conducted outside of living organisms. In-vitro studies, derived from the Latin term "in glass," involve experiments performed in controlled laboratory settings using cells or tissues. It is important to note that these products are not categorized as medicines or drugs, and they have not received approval from the FDA for the prevention, treatment, or cure of any medical condition, ailment, or disease. We must emphasize that any form of bodily introduction of these products into humans or animals is strictly prohibited by law. It is essential to adhere to these guidelines to ensure compliance with legal and ethical standards in research and experimentation.