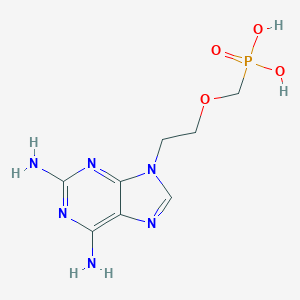
9-(2-Phosphonylmethoxyethyl)-2,6-diaminopurine
- Click on QUICK INQUIRY to receive a quote from our team of experts.
- With the quality product at a COMPETITIVE price, you can focus more on your research.
Overview
Description
9-(2-Phosphonylmethoxyethyl)-2,6-diaminopurine (PMEDAP) is an acyclic nucleoside phosphonate (ANP) belonging to the phosphonomethoxyethyl (PME) series. Its structure features a 2,6-diaminopurine base linked to a phosphonomethoxyethyl side chain, enabling direct interaction with viral DNA polymerases without requiring initial phosphorylation . PMEDAP demonstrates broad-spectrum antiviral activity against herpesviruses (e.g., HHV-6, HCMV) and retroviruses (e.g., HIV) in vitro . Additionally, it exhibits potent cytostatic effects, making it a candidate for antitumor therapy .
Chemical Reactions Analysis
GS 0573 undergoes various chemical reactions typical of purine derivatives. These reactions include:
Oxidation: The compound can be oxidized under specific conditions, although detailed reagents and conditions are not specified.
Reduction: Reduction reactions can also occur, but specific details are not provided.
Substitution: GS 0573 can undergo substitution reactions, particularly at the amino groups and the phosphonylmethoxyethyl group.
Common reagents and conditions for these reactions are not explicitly mentioned in the available literature. The major products formed from these reactions would depend on the specific reagents and conditions used.
Scientific Research Applications
Antiviral Applications
Mechanism of Action
PMEDAP acts as a prodrug that is metabolized into its active form, which inhibits viral replication. It has shown effectiveness against retroviruses and cytomegalovirus (CMV) infections in animal models. The compound's mechanism involves interference with nucleic acid synthesis, thereby inhibiting viral proliferation.
Case Study: Efficacy Against Retroviruses
A significant study published in the Journal of Medical Virology demonstrated that PMEDAP was effective in treating retrovirus infections in mice. The study reported a marked reduction in viral load following administration of the compound, highlighting its potential as a therapeutic agent for retroviral diseases such as HIV and HTLV .
Treatment of Cytomegalovirus Infections
PMEDAP has been specifically noted for its antiviral properties against CMV. In experimental settings, it has been shown to reduce the severity of CMV-induced pathology in infected mice. The compound's ability to inhibit CMV replication suggests it could be a valuable option for treating CMV infections, particularly in immunocompromised patients .
Research on Interleukin Modulation
Recent studies have suggested that PMEDAP may also play a role in modulating the effects of interleukins and other cytokines, which are critical in immune response regulation. This aspect opens avenues for further research into its potential use in inflammatory diseases and conditions where cytokine imbalance is a factor .
Pharmacokinetics and Bioavailability
The pharmacokinetic profile of PMEDAP indicates that it is designed to resist premature degradation, enhancing its bioavailability. Studies have shown that the compound can be effectively absorbed and utilized by the body, making it suitable for oral administration .
Comparative Efficacy with Other Antivirals
A comparative analysis of PMEDAP with other antiviral agents reveals that it exhibits comparable efficacy with certain established treatments while potentially offering a different mechanism of action that could be beneficial in cases of drug resistance .
Data Table: Summary of Applications
Mechanism of Action
The exact mechanism of action of GS 0573 is not fully elucidated. as a purine derivative, it is likely to interfere with viral replication by targeting viral DNA or RNA synthesis. The molecular targets and pathways involved are not explicitly detailed in the available literature .
Comparison with Similar Compounds
PME Series Derivatives
PMEA (Adefovir)
- Structure : Adenine base with the same PME side chain.
- Activity : Approved for HBV treatment (Hepsera®) at low doses due to dose-dependent toxicity in HIV therapy. PMEA is less potent than PMEDAP against HCMV and HHV-6 .
- Mechanism : Inhibits viral DNA polymerases but requires higher concentrations (EC₅₀: 25 μM) compared to PMEDAP (EC₅₀: 6 μM) to inhibit cellular DNA synthesis .
PMEG
- Structure : Guanine base with the PME side chain.
- Activity : Most potent PME derivative, inhibiting DNA synthesis at 1 μM (EC₅₀) due to efficient phosphorylation to its diphosphate form (PMEGpp). PMEGpp is incorporated into DNA 7-fold more efficiently than PMEApp (PMEA’s active form) .
- Limitations : High cytotoxicity limits clinical use despite superior antiviral activity .
N⁶-Substituted PMEDAP Derivatives
- Examples : N⁶-cyclopropyl-PMEDAP (cPr-PMEDAP).
- Activity: cPr-PMEDAP acts as a prodrug of PMEG, achieving complete tumor inhibition in rat choriocarcinoma models at 10 mg/kg (vs. 50 mg/kg for PMEDAP). It also exhibits prolonged antitumor effects post-treatment .
- Advantage : Enhanced therapeutic index due to reduced toxicity compared to PMEDAP and PMEG .
HPMP Series Derivatives
HPMPC (Cidofovir)
- Structure : Cytosine base with a hydroxypropoxymethylphosphonate (HPMP) side chain.
- Activity : Broad antiviral activity against dsDNA viruses (e.g., BK virus, EC₅₀: 2.5–10 μM) with higher potency than PMEDAP (EC₅₀: 13–64 μM) .
- Clinical Use : Approved for CMV retinitis, highlighting the HPMP series’ superior clinical translation .
HPMPDAP
- Structure: 2,6-Diaminopurine base with HPMP side chain.
- Activity : Inhibits DNA polymerase ε selectively, unlike PMEDAPpp, which targets polymerase δ . This specificity may reduce off-target effects in antiviral therapy .
Key Comparative Data
Table 1. Antiviral Activity and Selectivity
Compound | Target Virus (EC₅₀, μM) | Selectivity Index (SI) | Key Enzyme Inhibition |
---|---|---|---|
PMEDAP | HCMV (0.2–1.0), HIV (>10) | 5–10 | DNA polymerase δ (Ki: 0.3 μM) |
PMEA | HBV (0.1), HIV (25) | 20–50 | DNA polymerase α (Ki: 5 μM) |
PMEG | HIV (1.0), MCMV (0.5) | 2–5 | DNA polymerase δ (Ki: 0.1 μM) |
HPMPC | BK virus (2.5–10) | 10–46 | DNA polymerase ε (Ki: 0.05 μM) |
cPr-PMEDAP | Rat choriocarcinoma (10) | 15–20 | DNA polymerase δ (Ki: 0.2 μM) |
Table 2. Metabolic and Pharmacokinetic Properties
Compound | Phosphorylation Efficiency | Intracellular Half-life | Key Metabolite |
---|---|---|---|
PMEDAP | Moderate | 6–8 hours | PMEDAPpp |
PMEA | Low | 4–6 hours | PMEApp |
PMEG | High | 12–24 hours | PMEGpp |
HPMPC | High | >24 hours | HPMPCpp |
Data from
Biological Activity
9-(2-Phosphonylmethoxyethyl)-2,6-diaminopurine (PMEDAP) is an acyclic nucleoside phosphonate that has garnered attention for its potential therapeutic applications, particularly in antiviral treatments. This compound is structurally related to other nucleoside analogs and exhibits a range of biological activities, including antiviral and antiproliferative effects. This article delves into the biological activity of PMEDAP, summarizing key research findings, mechanisms of action, and relevant case studies.
Chemical Structure and Properties
PMEDAP belongs to the family of phosphonate compounds characterized by the presence of a phosphonylmethoxyethyl side chain attached to a 2,6-diaminopurine base. Its molecular formula is C₁₃H₁₈N₅O₃P, and it has a molecular weight of approximately 319.28 g/mol. The compound's unique structure contributes to its biological activity and pharmacokinetic properties.
PMEDAP exhibits its biological effects primarily through the following mechanisms:
- Inhibition of Viral Replication : PMEDAP acts as a competitive inhibitor of viral reverse transcriptase, thereby disrupting the replication cycle of retroviruses such as HIV and FIV (feline immunodeficiency virus) .
- Antiproliferative Effects : In vitro studies have demonstrated that PMEDAP can inhibit the growth of various tumor cell lines, showcasing its potential as an anticancer agent. The compound's IC50 values indicate significant antiproliferative activity comparable to related compounds like 9-(2-phosphonylmethoxyethyl)guanine (PMEG) .
Biological Activity Overview
The biological activities of PMEDAP can be summarized in the following table:
Antiviral Efficacy
A clinical study investigated the efficacy of PMEDAP in treating FIV-infected cats. The study involved 45 cats randomly assigned to receive either PMEDAP or a placebo over six weeks. Results indicated no significant improvements in clinical or virological parameters among treated groups compared to placebo, although some adverse effects such as anemia were noted .
Antiproliferative Activity
In another study, PMEDAP was tested against a broad range of tumor cell lines. The results showed that PMEDAP exhibited potent antiproliferative activity with IC50 values suggesting effectiveness similar to established antiviral agents. The study highlighted the compound's potential for further development as an anticancer therapeutic .
Q & A
Q. What are the key synthetic pathways for producing PMEDAP and its derivatives?
PMEDAP derivatives are synthesized via nucleophilic substitution and phosphonate coupling. A common method involves reacting 2,6-diaminopurine with alkyl glycidyl ethers under NaH catalysis in DMF at 100°C (Procedure A). Subsequent phosphonate introduction uses sodium t-butoxide and alkoxyalkyl p-toluenesulfonyloxymethylphosphonate at 80°C (Procedure C). For example, octadecyloxyethyl derivatives are synthesized with 49% yield, confirmed by NMR and mass spectrometry .
Q. How does PMEDAP selectively inhibit HIV reverse transcriptase (RT)?
PMEDAP's diphosphate form (PMEDAPpp) competitively inhibits HIV RT by binding to the active site, with higher affinity for RNA templates (Ki = 11–22 nM) compared to DNA. This selectivity arises from its structural mimicry of dATP, disrupting RNA-dependent DNA synthesis. Its potency exceeds AZTTP (Ki = 8 nM) but requires careful evaluation of template specificity in enzymatic assays .
Q. How do researchers address PMEDAP's cytotoxicity in antiviral studies?
PMEDAP's cytotoxicity stems from off-target inhibition of human DNA polymerases (e.g., Pol γ, Ki = 1.2 µM). Mitigation strategies include:
- Dose optimization : Balancing EC₅₀ (antiviral) vs. CC₅₀ (cytotoxic) values.
- Prodrug design : Alkyloxyalkyl esters (e.g., hexadecyloxypropyl) improve cellular uptake and reduce non-specific phosphorylation .
Q. What mechanisms drive resistance to PMEDAP in viral or cancer models?
Resistance arises via loss of adenine phosphoribosyltransferase (APRT) activity, preventing metabolic activation of PMEDAP to its diphosphate form. In E. coli and leukemia cells, APRT-deficient mutants show >10-fold reduced susceptibility. Combinatorial therapy with APRT-inducing agents may overcome resistance .
Q. How do in vivo efficacy studies for PMEDAP differ from in vitro models?
In vivo studies (e.g., Moloney murine sarcoma virus models) require:
- Pharmacokinetic profiling : Assessing prodrug hydrolysis to PMEDAP and PMEG.
- Tissue distribution analysis : PMEDAP accumulates in lymphoid tissues, necessitating LC-MS/MS quantification .
Q. What experimental approaches validate PMEDAP's selectivity for viral enzymes?
- Comparative kinetics : Measure Ki values for HIV RT vs. human polymerases (α, β, γ) using radiolabeled dNTPs.
- Template-switch assays : Use RNA/DNA hybrid templates to confirm RNA-selective inhibition .
Q. How do contradictory data on PMEDAP's efficacy vs. toxicity inform experimental design?
While PMEDAP shows superior anti-HBV activity vs. adefovir (EC₅₀ = 0.1 µM vs. 1 µM), its cytotoxicity (CC₅₀ = 10 µM) complicates clinical translation. Researchers must:
- Use isogenic cell lines : Compare APRT⁺/APRT⁻ cells to isolate toxicity mechanisms.
- Evaluate metabolite profiles : Quantify intracellular PMEDAPpp vs. unphosphorylated forms .
Q. How do structural modifications of PMEDAP enhance antiviral properties?
- Alkoxyalkyl side chains : Improve membrane permeability (e.g., octadecyloxyethyl increases logP by 3.5).
- N⁶ substitutions : Cyclopropyl or dimethylaminoethyl groups enhance RT binding affinity while reducing Pol γ inhibition .
Q. What role does 2,6-diaminopurine play in bioengineering applications?
Incorporating 2,6-diaminopurine (Z base) into oligonucleotides enhances RNA binding (ΔTm = +5°C vs. adenine). This property is leveraged in anti-miRNA SNAs (serinol nucleic acids) for gene silencing .
Q. What analytical methods quantify PMEDAP in biological matrices?
Preparation Methods
Synthetic Strategies for PMEDAP Core Structure
Base Nucleoside Preparation
The synthesis of PMEDAP begins with the preparation of the 2,6-diaminopurine scaffold. Holý et al. established a method starting with 2,6-diaminopurine, which is alkylated at the N9 position using 2-(hydroxymethyl)ethylene glycol followed by phosphonate incorporation . The ethylene glycol moiety is activated via tosylation to facilitate nucleophilic displacement, yielding the intermediate 9-(2-hydroxyethoxyethyl)-2,6-diaminopurine. This step typically achieves 60–75% yield, with purity confirmed by thin-layer chromatography (TLC) and nuclear magnetic resonance (NMR) .
Phosphonate Linkage Installation
Phosphonylation of the hydroxyl group is critical for conferring antiviral activity. Two primary approaches dominate:
-
Direct Phosphorylation : Reaction with phosphorus oxychloride (POCl₃) in trimethyl phosphate, followed by hydrolysis, produces the phosphonic acid derivative. This method, however, often results in low yields (30–40%) due to side reactions .
-
Diethyl Phosphonate Intermediate : A more efficient route involves coupling the hydroxylated intermediate with diethyl phosphite under Mitsunobu conditions (DIAD, triphenylphosphine). Subsequent acid hydrolysis (6M HCl, reflux) removes ethyl protecting groups, yielding PMEDAP with 55–65% overall yield .
Phosphorylation and Prodrug Derivatization
Prodrug Synthesis for Enhanced Bioavailability
PMEDAP’s poor membrane permeability necessitates prodrug formulations. The bis(pivaloyloxymethyl) (POM) and bis(butyl-l-alaninyl) esters are widely used:
-
Bis(POM)-PMEDAP : Treatment of PMEDAP with chloromethyl pivalate in the presence of NaH yields the bis(POM) prodrug. This method, adapted from PMEA prodrug synthesis, achieves 60–70% conversion .
-
Bis(Alaninyl) Derivatives : Coupling PMEDAP with N-protected l-alanine esters using carbodiimide chemistry, followed by deprotection, enhances cellular uptake. For example, bis(butyl-l-alaninyl)PMEDAP exhibits a 10-fold reduction in EC₅₀ compared to PMEDAP in antiviral assays .
Table 1. Prodrug Efficacy Against Vaccinia Virus (VV) and Cowpox Virus (CV)
Compound | CC₅₀ (μM) | VV EC₅₀ (μM) | CV EC₅₀ (μM) | Selectivity Index (VV) |
---|---|---|---|---|
PMEDAP | >339 | 204 | >347 | 1.7 |
Bis(POM)-PMEDAP | 117 | 5.1 | 13 | 23 |
Bis(Butyl-l-alaninyl)PMEDAP | 100 | 4.4 | 10 | 23 |
Data sourced from , Table 2. |
Purification and Analytical Characterization
Chromatographic Techniques
Crude PMEDAP is purified via reverse-phase high-performance liquid chromatography (RP-HPLC) using a C18 column and gradient elution (water/acetonitrile with 0.1% trifluoroacetic acid). This step resolves phosphonate diastereomers, achieving >98% purity . For prodrugs, silica gel chromatography with ethyl acetate/methanol gradients removes unreacted starting materials .
Spectroscopic Validation
-
NMR : ¹H NMR (D₂O, 400 MHz) of PMEDAP shows characteristic peaks at δ 8.12 (s, 1H, H8), 6.45 (br, 4H, NH₂), and 4.10–3.80 (m, 4H, CH₂OCH₂) .
-
Mass Spectrometry : ESI-MS confirms the molecular ion [M-H]⁻ at m/z 317.1 for PMEDAP and [M+H]⁺ at m/z 603.3 for bis(POM)-PMEDAP .
Scale-Up and Process Optimization
Industrial-scale synthesis requires addressing phosphonate hydrolysis and byproduct formation. Key advancements include:
-
Continuous Flow Reactors : Minimizing residence time during POCl₃ reactions reduces degradation, improving yields to 50% at the kilogram scale .
-
Crystallization Conditions : Recrystallization from ethanol/water (1:3) at 4°C yields PMEDAP as a white crystalline solid with 99.5% purity, suitable for pharmaceutical formulation .
Applications and Biological Relevance
PMEDAP’s diphosphate metabolite inhibits viral DNA polymerases (e.g., vaccinia virus) at nanomolar concentrations. Its N6-cyclopropyl derivative (cPrPMEDAP) demonstrates enhanced potency, with EC₅₀ values of 0.08 μM against VV, attributed to improved intracellular retention . In vivo studies using topical formulations (e.g., GS-9191) show complete regression of papillomas in rabbit models, underscoring its translational potential .
Properties
CAS No. |
113852-41-8 |
---|---|
Molecular Formula |
C8H13N6O4P |
Molecular Weight |
288.20 g/mol |
IUPAC Name |
2-(2,6-diaminopurin-9-yl)ethoxymethylphosphonic acid |
InChI |
InChI=1S/C8H13N6O4P/c9-6-5-7(13-8(10)12-6)14(3-11-5)1-2-18-4-19(15,16)17/h3H,1-2,4H2,(H2,15,16,17)(H4,9,10,12,13) |
InChI Key |
XHXFQGAZAVKMFF-UHFFFAOYSA-N |
SMILES |
C1=NC2=C(N=C(N=C2N1CCOCP(=O)(O)O)N)N |
Canonical SMILES |
C1=NC2=C(N=C(N=C2N1CCOCP(=O)(O)O)N)N |
Key on ui other cas no. |
113852-41-8 |
Synonyms |
9-(2-phosphonylmethoxyethyl)-2,6-diaminopurine PMEDAP |
Origin of Product |
United States |
Disclaimer and Information on In-Vitro Research Products
Please be aware that all articles and product information presented on BenchChem are intended solely for informational purposes. The products available for purchase on BenchChem are specifically designed for in-vitro studies, which are conducted outside of living organisms. In-vitro studies, derived from the Latin term "in glass," involve experiments performed in controlled laboratory settings using cells or tissues. It is important to note that these products are not categorized as medicines or drugs, and they have not received approval from the FDA for the prevention, treatment, or cure of any medical condition, ailment, or disease. We must emphasize that any form of bodily introduction of these products into humans or animals is strictly prohibited by law. It is essential to adhere to these guidelines to ensure compliance with legal and ethical standards in research and experimentation.