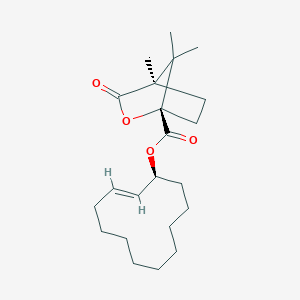
2-Cyclododecenyl camphanate
- Click on QUICK INQUIRY to receive a quote from our team of experts.
- With the quality product at a COMPETITIVE price, you can focus more on your research.
Overview
Description
2-Cyclododecenyl camphanate is a chiral organic ester characterized by a 12-membered cycloalkenyl ring fused to a camphanate moiety. The camphanate group, derived from camphor, is widely utilized in stereochemical studies due to its rigid bicyclic structure, which aids in resolving enantiomers via diastereomeric crystallization . The compound exists in both E (trans) and Z (cis) isomeric forms, with the E isomer being more prevalent in crystallographic studies. Its structure has been elucidated via X-ray diffraction, revealing a loosely packed monoclinic crystal lattice (space group P2₁) and significant conformational flexibility due to the trans double bond in the cyclododecenyl ring .
Preparation Methods
Synthetic Routes and Reaction Conditions: : Beta-Ergocryptine is typically isolated from ergot or fermentation broth. The biosynthetic pathway begins with the prenylation of L-tryptophan using dimethylallyl pyrophosphate (DMAPP), derived from mevalonic acid. This reaction is catalyzed by a prenyltransferase enzyme named FgaPT2 in Aspergillus fumigatus .
Industrial Production Methods: : Industrial production of beta-Ergocryptine involves the cultivation of ergot fungi, particularly Claviceps purpurea, on suitable substrates. The ergot sclerotia are harvested, and the alkaloids are extracted and purified using various chromatographic techniques .
Chemical Reactions Analysis
Hydrolysis of the Camphanate Ester
The ester linkage in 2-cyclododecenyl camphanate is susceptible to hydrolysis under acidic or basic conditions. This reaction would yield cyclododecenol and camphanic acid.
Proposed Reaction:
2 Cyclododecenyl camphanate+H2OH+ or OH−Cyclododecenol+Camphanic Acid
Key Factors:
-
Acid-Catalyzed Hydrolysis : Protonation of the ester carbonyl enhances electrophilicity, facilitating nucleophilic attack by water.
-
Base-Catalyzed Hydrolysis (Saponification) : Hydroxide ions deprotonate water, generating a stronger nucleophile for ester cleavage.
Comparison to Related Esters :
Ester Type | Hydrolysis Conditions | Products |
---|---|---|
Camphanate esters | Acidic (HCl/H₂O) | Alcohol + Camphanic acid |
Acetate esters | Basic (NaOH/H₂O) | Alcohol + Acetate salt |
Hydrogenation of the Cyclododecene Ring
The cyclododecene moiety contains a double bond that could undergo hydrogenation to form a saturated cyclododecane derivative.
Proposed Reaction:
2 Cyclododecenyl camphanate+H2Pt PdCyclododecanyl camphanate
Catalyst Sensitivity 6:
-
Raney Nickel : Effective for hydrogenating cyclic alkenes but may require elevated temperatures.
-
Platinum/Palladium : Operates under milder conditions but is cost-prohibitive for large-scale reactions.
Oxidation of the Cyclododecene Ring
The double bond in the cyclododecene group may undergo epoxidation or dihydroxylation.
Epoxidation with Peracids :
2 Cyclododecenyl camphanate+RCO3H→Epoxide derivative+RCO2H
Experimental Insights from Cyclododecatriene :
-
Selective epoxidation of cyclododecatriene (CDT) with H₂O₂/HAHPT catalyst achieves 96.7% selectivity for diepoxides at 35°C.
-
Reaction conditions for this compound would likely require optimization to avoid over-oxidation.
Thermal Decomposition
Camphanate esters are thermally stable but may decompose under extreme conditions.
Potential Pathways6 :
-
Decarboxylation : Loss of CO₂ from the camphanate group.
-
Retro-Diels-Alder : Fragmentation of the bicyclic camphanate structure.
Thermogravimetric Analysis (Hypothetical):
Temperature Range (°C) | Mass Loss (%) | Proposed Decomposition Product |
---|---|---|
200–250 | 15–20 | Cyclododecene + CO₂ |
300–350 | 30–40 | Isomeric hydrocarbons |
Enzymatic Hydrolysis
Camphanate esters are chiral, making them substrates for esterases or lipases.
Stereochemical Considerations :
-
Enzymes like Candida antarctica lipase B (CAL-B) could selectively hydrolyve one enantiomer, enabling kinetic resolution.
Reaction with Grignard Reagents
The ester carbonyl could react with Grignard reagents to form tertiary alcohols.
Proposed Mechanism:
-
Nucleophilic attack by RMgX on the carbonyl carbon.
-
Protonation of the intermediate to yield alcohol.
2 Cyclododecenyl camphanate+RMgX→Tertiary alcohol+MgX OR
Limitations and Research Gaps
Scientific Research Applications
Beta-Ergocryptine has a wide range of scientific research applications:
Chemistry: It serves as a starting material for the synthesis of other ergot alkaloids, such as bromocriptine.
Biology: It is used to study the biosynthesis and metabolism of ergot alkaloids in fungi.
Medicine: Beta-Ergocryptine and its derivatives have been investigated for their potential therapeutic effects, particularly in the treatment of neurological disorders.
Industry: It is used in the production of pharmaceuticals and as a research tool in various biochemical studies
Mechanism of Action
Beta-Ergocryptine exerts its effects primarily through interactions with neurotransmitter receptors in the central nervous system. It acts as an agonist or antagonist at various serotonin, dopamine, and adrenergic receptors. This interaction modulates the release of neurotransmitters, thereby influencing various physiological processes .
Comparison with Similar Compounds
Structural and Crystallographic Differences
The structural and crystallographic properties of 2-cyclododecenyl camphanate are distinct from related camphanate esters (Table 1):
- Ring Size and Flexibility : The 12-membered ring in this compound exhibits greater conformational mobility compared to the 11-membered ring in 2-bromo-2-cycloundecenyl camphanate. This is attributed to reduced steric hindrance and the trans double bond, which allows for torsional adjustments .
- Substituent Effects : The bromine substituent in the cycloundecenyl derivative introduces steric and electronic effects, stabilizing the cis (Z) configuration and reducing ring flexibility. In contrast, this compound lacks bulky substituents, enabling looser molecular packing .
- Crystallographic Parameters: While both compounds crystallize in monoclinic systems, the unit cell volume of the cycloundecenyl derivative (1,063.80 ų) is smaller than that of the cyclododecenyl analog, reflecting differences in ring size and substituent bulk .
Research Findings and Key Insights
- Crystal Packing : The loosely packed structure of (E)-2-cyclododecenyl camphanate results in a lower density (1.334 g/cm³) compared to gem-dichlorodiarylcyclopropane derivatives, which exhibit tighter packing due to aryl group interactions .
- Thermal Stability : The trans double bond in this compound reduces ring strain, enhancing thermal stability relative to cyclopropane-based analogs .
- Synthetic Utility: Camphanate esters are synthesized via esterification of camphanoyl chloride with cycloalkenols. The reaction’s yield and stereochemical outcome depend on the cycloalkenyl substrate’s ring size and substituents .
Q & A
Basic Research Questions
Q. What established methods are used to synthesize 2-Cyclododecenyl camphanate, and how do reaction conditions influence diastereoselectivity?
- Methodological Answer : Synthesis typically involves esterification of cyclododecenol with camphanic acid derivatives under controlled conditions. Diastereoselectivity is enhanced using sterically hindered camphanoyl chlorides and chiral catalysts. Chromatographic resolution (e.g., silica gel chromatography) is critical for isolating enantiomers, as demonstrated in racemic diol resolution via camphanate derivatization . Reaction temperature and solvent polarity significantly impact stereochemical outcomes, with lower temperatures favoring kinetic control .
Q. Which spectroscopic techniques are essential for characterizing the stereochemical configuration of this compound?
- Methodological Answer : Nuclear Magnetic Resonance (NMR) spectroscopy, particularly NOESY/ROESY for spatial proximity analysis, and X-ray crystallography for absolute configuration determination. Coupling constants in 1H-NMR help infer double-bond geometry (e.g., trans configuration), while 13C-NMR identifies carbonyl and ester functionalities. X-ray diffraction provides definitive proof of molecular conformation and crystal packing effects .
Q. How does the crystal packing of this compound influence its molecular mobility, and what experimental approaches quantify this?
- Methodological Answer : Crystal packing density, influenced by trans double bonds or bulky substituents, affects conformational mobility. Thermal ellipsoid analysis in X-ray crystallography reveals atomic displacement parameters, while molecular mechanics simulations model torsional flexibility. Comparative studies with structurally similar compounds (e.g., meta-cyclophanes) highlight packing efficiency differences .
Advanced Research Questions
Q. How can researchers resolve contradictions between computational models and experimental crystallographic data regarding conformational flexibility?
- Methodological Answer : Integrate molecular dynamics (MD) simulations with experimental X-ray data. MD trajectories identify low-energy conformers, while crystallographic B-factors (thermal ellipsoids) validate dynamic behavior. Discrepancies in torsion angles (e.g., due to trans double bonds) require iterative refinement of force field parameters to align simulations with observed data .
Q. What strategies mitigate racemization during the synthesis of enantiomerically pure this compound derivatives?
- Methodological Answer : Use chiral auxiliaries (e.g., enantiopure camphanic acid) to enforce stereochemical control. Low-temperature reaction conditions minimize epimerization, while kinetic resolution via enzymatic hydrolysis or chiral stationary-phase chromatography ensures enantiopurity. Post-synthetic analysis with polarimetry or chiral HPLC confirms retention of configuration .
Q. How do steric and electronic effects in camphanate derivatives influence regioselectivity during ring-opening or functionalization reactions?
- Methodological Answer : Steric hindrance from the camphanate’s bicyclic structure directs nucleophilic attacks to less hindered sites. For example, HBr-mediated ring-opening of oxa-derivatives favors regioselectivity at sterically accessible positions. Electronic effects are probed via Hammett plots or DFT calculations to predict reaction pathways .
Q. What analytical frameworks address uncertainties in crystallographic data interpretation for flexible molecules like this compound?
- Methodological Answer : Apply multi-conformer crystallographic models and disorder modeling in refinement software (e.g., SHELXL). Pair with solid-state NMR to validate dynamic disorder. Statistical tools like R-factors and electron density maps assess model accuracy, while comparative analysis with related structures identifies outliers .
Q. Data Analysis and Contradiction Management
Q. How should researchers handle discrepancies between observed and simulated molecular geometries?
- Methodological Answer : Perform sensitivity analysis on computational parameters (e.g., van der Waals radii, torsional barriers). Cross-validate with spectroscopic data (e.g., IR/Raman for bond vibrations) and alternative simulation methods (e.g., ab initio vs. semi-empirical). Publish negative results to refine community-wide force fields .
Q. What protocols ensure reproducibility in stereochemical assignments for camphanate derivatives?
- Methodological Answer : Adopt IUPAC guidelines for stereodescriptors and deposit crystallographic data in public repositories (e.g., CCDC). Use enantiomeric excess (ee) calculations via HPLC peak integration and report solvent/temperature conditions comprehensively. Peer validation through independent synthesis and characterization is critical .
Properties
CAS No. |
120450-70-6 |
---|---|
Molecular Formula |
C23H38O2 |
Molecular Weight |
362.5 g/mol |
IUPAC Name |
[(1S,2E)-cyclododec-2-en-1-yl] (1S,4R)-4,7,7-trimethyl-3-oxo-2-oxabicyclo[2.2.1]heptane-1-carboxylate |
InChI |
InChI=1S/C22H34O4/c1-20(2)21(3)15-16-22(20,26-18(21)23)19(24)25-17-13-11-9-7-5-4-6-8-10-12-14-17/h11,13,17H,4-10,12,14-16H2,1-3H3/b13-11+/t17-,21+,22-/m1/s1 |
InChI Key |
XRTQJRZAEFORQH-UHFFFAOYSA-N |
SMILES |
CC1(C2(CCC1(OC2=O)C(=O)OC3CCCCCCCCCC=C3)C)C |
Isomeric SMILES |
C[C@@]12CC[C@@](C1(C)C)(OC2=O)C(=O)O[C@H]\3CCCCCCCCC/C=C3 |
Canonical SMILES |
CC1(C2(CCC1(OC2=O)C(=O)OC3CCCCCCCCCC=C3)C)C |
Synonyms |
2-cyclododecenyl camphanate |
Origin of Product |
United States |
Disclaimer and Information on In-Vitro Research Products
Please be aware that all articles and product information presented on BenchChem are intended solely for informational purposes. The products available for purchase on BenchChem are specifically designed for in-vitro studies, which are conducted outside of living organisms. In-vitro studies, derived from the Latin term "in glass," involve experiments performed in controlled laboratory settings using cells or tissues. It is important to note that these products are not categorized as medicines or drugs, and they have not received approval from the FDA for the prevention, treatment, or cure of any medical condition, ailment, or disease. We must emphasize that any form of bodily introduction of these products into humans or animals is strictly prohibited by law. It is essential to adhere to these guidelines to ensure compliance with legal and ethical standards in research and experimentation.