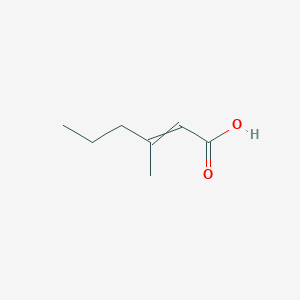
3-Methylhex-2-enoic acid
Overview
Description
3-Methylhex-2-enoic acid (3MHA), also known as (E)-3-methylhex-2-enoic acid (CAS: 27960-21-0), is a branched-chain unsaturated carboxylic acid with the molecular formula C₇H₁₂O₂ and a molecular weight of 128.17 g/mol . It is a key component of human axillary odor, produced when skin bacteria such as Corynebacterium spp. hydrolyze N-acyl-glutamine conjugates secreted in sweat . The compound’s (E)-configuration double bond at the C2 position contributes to its volatility and odor potency. 3MHA is associated with pungent, fatty, and cheesy odor notes and is detectable at low concentrations due to its lipophilic nature .
Preparation Methods
Catalytic Rearrangement and Oxidation
Rearrangement of Alkynol Precursors
The synthesis of α,β-unsaturated carboxylic acids often begins with alkynol precursors. For 3-methylhex-2-enoic acid, 2-methyl-3-hexyn-2-ol serves as a critical intermediate. In a process adapted from the preparation of 3-methyl-2-butenoic acid , this alkynol undergoes catalytic rearrangement in the presence of:
-
Methyl ethyl diketone oxygen titanium (0.01 mol%)
-
Cuprous bromide (0.01 mol%)
-
Benzoic acid (0.05 mol%)
The reaction proceeds in whiteruss (a high-boiling solvent) at 110–120°C for 3 hours, yielding 3-methyl-2-hexenal with ~90% efficiency .
Oxidation of 3-Methyl-2-Hexenal
The resulting aldehyde is oxidized to the target acid using air or oxygen under catalytic conditions:
Catalyst | Temperature (°C) | Pressure (MPa) | Yield (%) |
---|---|---|---|
Manganese acetate | 30–40 | 0.1–0.2 | 82 |
Cuprous chloride | 60–70 | 0.3 | 84.8 |
Cobaltous diacetate | 40–60 | 0.3 | 87.7 |
Notably, continuous aeration and stirring rates of 500–1,000 rpm are critical to achieving high yields . The oxidation mechanism proceeds via radical intermediates, with metal catalysts facilitating electron transfer to molecular oxygen .
Hydrolysis of Ethyl Ester Derivatives
Synthesis of Ethyl (E)-3-Methyl-2-Hexenoate
The ethyl ester precursor is synthesized via Knoevenagel condensation between pentanal and ethyl acetoacetate under basic conditions (e.g., piperidine catalyst). This forms the α,β-unsaturated ester with >75% yield .
Saponification and Acid Work-Up
The ester undergoes hydrolysis in 2M NaOH at reflux, followed by acidification with HCl to precipitate the free acid:
This method achieves ~85% purity, though residual ester may require chromatographic purification .
Metal-Catalyzed Oxidative Methods
Direct Oxidation of 3-Methyl-2-Hexenol
Primary alcohols can be oxidized to carboxylic acids using Jones reagent (CrO₃/H₂SO₄):
3/\text{H}2\text{SO}_4} \text{this compound}
However, over-oxidation risks necessitate careful temperature control (0–5°C) .
Aerobic Oxidation with Heterogeneous Catalysts
Recent advances employ Au-Pd/TiO₂ nanoparticles under mild conditions (25°C, 1 atm O₂), achieving 78% conversion in 12 hours. This method minimizes side products like ketones or epoxides .
Comparative Analysis of Methods
Method | Starting Material | Catalyst System | Yield (%) | Purity (%) | Cost Efficiency |
---|---|---|---|---|---|
Rearrangement-Oxidation | 2-Methyl-3-hexyn-2-ol | Ti/Cu/Benzoic acid | 82–87.7 | 95 | High |
Ester Hydrolysis | Ethyl ester | NaOH/HCl | 75–85 | 90 | Moderate |
Direct Alcohol Oxidation | 3-Methyl-2-hexenol | CrO₃/H₂SO₄ | 65–70 | 85 | Low |
The rearrangement-oxidation route is industrially favored due to scalability and cost-effective catalysts, while ester hydrolysis suits small-scale laboratory synthesis .
Challenges and Optimization Strategies
Stereochemical Control
The (E)-isomer predominates in catalytic oxidations due to steric hindrance during aldehyde formation . To isolate the (Z)-isomer, low-temperature photoisomerization (λ = 254 nm, -20°C) is employed, though yields drop to ~40% .
Byproduct Mitigation
Scientific Research Applications
Chemical Synthesis
Building Block in Organic Chemistry
3-Methylhex-2-enoic acid serves as a crucial building block in organic synthesis. It is often employed in the formation of more complex molecules through various reactions such as aldol condensation and electrophilic addition. The compound's double bond allows for versatile reactivity, making it suitable for multiple synthetic pathways.
Synthesis Methods
The synthesis of this compound can be achieved through several methods:
- Aldol Condensation : Involves the reaction of butanal with acetone followed by dehydration.
- Catalytic Hydrogenation : Used for large-scale production from 3-methylhex-2-enal, followed by oxidation.
Synthesis Method | Description |
---|---|
Aldol Condensation | Reaction between butanal and acetone |
Catalytic Hydrogenation | Production from 3-methylhex-2-enal |
Biological Research
Role in Human Metabolism
Research indicates that this compound is produced during the metabolism of certain compounds by skin bacteria, contributing to body odor. Its presence in human sweat has been linked to potential biomarkers for metabolic disorders, including schizophrenia. Studies suggest that variations in olfactory sensitivity to this compound may correlate with certain psychological conditions .
Biochemical Pathways
The compound interacts with various enzymes involved in fatty acid metabolism, influencing cellular signaling pathways and metabolic processes. It can activate or inhibit specific pathways, leading to significant changes in gene expression related to lipid metabolism.
Medical Applications
Potential Therapeutic Uses
Ongoing research explores the therapeutic potential of this compound in treating metabolic disorders. Its role as a biomarker in sweat analysis may provide insights into diagnosing conditions like schizophrenia and other metabolic syndromes .
Industrial Applications
Flavor and Fragrance Industry
Due to its distinctive odor, this compound is utilized extensively in the flavor and fragrance industry. It is incorporated into perfumes, deodorants, and other scented products to enhance their olfactory profiles.
Industry Application | Description |
---|---|
Flavoring Agent | Used to impart specific flavors |
Fragrance Component | Contributes to scent formulations |
Case Studies
- Sweat Analysis for Schizophrenia Diagnosis
- Organic Synthesis Efficiency
Mechanism of Action
The mechanism of action of 3-Methylhex-2-enoic acid involves its interaction with specific molecular targets and pathways. As a fatty acid, it can be metabolized by enzymes such as fatty acid synthase and undergo β-oxidation to produce energy. Additionally, its unsaturated nature allows it to participate in signaling pathways related to lipid metabolism and inflammation .
Comparison with Similar Compounds
Comparison with Structurally Similar Compounds
Structural Analogs in Human Odor Biochemistry
3MHA is one of several odor-active compounds derived from bacterial metabolism in sweat. Key analogs include:
(RS)-3-Hydroxy-3-methylhexanoic Acid (HMHA)
- Structure : A β-hydroxy acid with a hydroxyl group at C3 and a methyl branch.
- Source : Generated alongside 3MHA via bacterial cleavage of N-α-acyl-glutamine conjugates .
- Odor Profile : Described as more "typical" of body odor than 3MHA, with a sour, rancid character .
- Perception: Cross-cultural studies show HMHA has lower detection thresholds compared to 3MHA, though ~8–19% of individuals exhibit anosmia to both compounds .
(R)/(S)-3-Methyl-3-sulfanylhexan-1-ol
- Structure : A volatile thiol with a sulfhydryl (-SH) group at C3.
- Source : Derived from cysteinglycine-S-conjugates in sweat by bacterial enzymes .
- Odor Profile: Contributes sulfurous, onion-like notes, distinct from the fatty acids 3MHA and HMHA .
3-Methyl-2-oxovaleric Acid
- Structure : A keto acid with a carbonyl group at C2.
- Source: Not directly linked to axillary odor but structurally related to branched-chain fatty acids.
- Role : A metabolic intermediate in leucine catabolism, associated with maple syrup urine disease .
Cross-Cultural Perceptual Studies
Biological Activity
3-Methylhex-2-enoic acid, also known as (E)-3-methyl-2-hexenoic acid, is a monounsaturated fatty acid characterized by its unique structure, which includes a double bond between the second and third carbon atoms. This compound is notable for its biological relevance, particularly in the context of human body odor and potential links to various health conditions.
- Molecular Formula : C7H12O2
- Molecular Weight : 128.17 g/mol
- CAS Number : 27960-21-0
This compound exists in both cis (Z) and trans (E) forms, with the trans form being more prevalent in biological systems. Its presence in human sweat is significant, as it contributes to body odor, particularly in individuals of Caucasian descent .
Contribution to Body Odor
This compound is a major component of axillary odor, resulting from the metabolic activity of skin bacteria that break down sweat precursors. The compound is produced during the metabolism of certain fatty acids and has been linked to the characteristic scent associated with human sweat .
Research indicates that this compound has a strong, unpleasant odor often described as "goaty" or "sweaty," which can vary among individuals based on genetic factors and bacterial flora on the skin .
Potential Links to Health Conditions
There have been studies suggesting a possible association between elevated levels of this compound and schizophrenia. It has been observed that individuals with schizophrenia may exhibit altered sensitivity to this compound, indicating a potential link between metabolic processes and sensory perception. However, these findings require further investigation to establish definitive connections .
Case Studies and Experiments
- Study on Twins : A study conducted on monozygotic twins analyzed the chemical composition of axillary odor. Results indicated a common pattern involving this compound, suggesting genetic factors influence body odor characteristics .
- Cultural Perception Study : Research comparing odor perception across cultures found that this compound was recognized as a significant contributor to body odor in both French and Malagasy populations. Sensitivity to this compound varied, with some individuals exhibiting anosmia (inability to perceive odors) towards it .
- Gender Differences : A study highlighted that men tend to produce higher levels of this compound compared to women due to differences in skin microbiota composition, particularly the presence of Corynebacteria, which are responsible for metabolizing sweat into malodorous compounds .
Comparative Analysis with Related Compounds
Compound Name | Structure | Unique Features |
---|---|---|
2-Hexenoic Acid | C6H10O2 | Saturated counterpart; less odoriferous |
3-Methylhexanoic Acid | C7H14O2 | Saturated fatty acid; lacks unsaturation |
Trans-3-Methyl-2-Hexenoic Acid | C7H12O2 | Geometric isomer; prominent in axillary odor |
4-Methylpentanoic Acid | C6H12O2 | Similar carbon chain length; different branching |
The distinct positioning of the double bond in this compound differentiates it from other similar compounds, contributing uniquely to its biological activity and odor profile .
Q & A
Basic Research Questions
Q. What are the standard synthetic routes for 3-methylhex-2-enoic acid, and how do reaction conditions influence isomer purity?
- Methodology : The compound often exists as a mixture of E and Z isomers. A common synthesis involves condensation of aldehydes with β-keto esters under acidic or basic conditions. For example, Claisen-Schmidt condensation followed by decarboxylation can yield the α,β-unsaturated acid. Isomer ratios (e.g., 3:1 E:Z ratio) depend on steric and electronic factors during synthesis .
- Data Consideration : Monitor reaction temperature (excessive heat promotes Z→E isomerization) and use chiral HPLC or GC-MS to quantify isomer distribution.
Q. How can the structure of this compound be confirmed using spectroscopic methods?
- Methodology :
- NMR : Distinct chemical shifts for the α,β-unsaturated system (δ ~5.8–6.5 ppm for protons on C2 and C3) and methyl branching (δ ~1.0–1.5 ppm). Coupling constants (J ~15–16 Hz for E isomers) confirm geometry .
- IR : Strong absorption at ~1700 cm⁻¹ (C=O stretch) and ~1640 cm⁻¹ (C=C stretch).
- Mass Spectrometry : Molecular ion peak at m/z 130 (C₇H₁₀O₂) with fragmentation patterns indicating loss of CO₂ (44 amu).
Q. What safety protocols are critical when handling this compound in the laboratory?
- Methodology : While specific GHS hazard data are limited for this compound, general precautions for α,β-unsaturated acids apply:
- Use fume hoods to avoid inhalation (potential respiratory irritant).
- Wear nitrile gloves and eye protection; avoid skin contact due to possible corrosivity .
- Store in airtight containers at 2–8°C to prevent polymerization or degradation.
Advanced Research Questions
Q. How do computational methods (e.g., DFT) predict the reactivity of this compound in Diels-Alder reactions?
- Methodology :
- Perform density functional theory (DFT) calculations (e.g., B3LYP/6-31G*) to model frontier molecular orbitals (HOMO-LUMO gaps) and predict regioselectivity.
- Compare with experimental results using substituted dienes (e.g., anthracene) to validate computational models .
- Data Contradictions : Discrepancies may arise between predicted endo/exo selectivity and experimental outcomes due to solvent effects or steric hindrance not fully captured in gas-phase calculations.
Q. What strategies resolve contradictions in reported pKa values for this compound?
- Methodology :
- Use potentiometric titration in aqueous and non-aqueous solvents (e.g., DMSO) to account for solvent-dependent ionization.
- Validate with UV-Vis spectroscopy by tracking deprotonation-induced shifts in λmax (e.g., ~210 nm for the carboxylate anion) .
- Critical Analysis : Literature inconsistencies may stem from isomer interference (e.g., E vs. Z isomers) or impurities in early synthetic batches.
Q. How can X-ray crystallography elucidate the solid-state conformation of this compound?
- Methodology :
- Grow single crystals via slow evaporation in ethanol/water mixtures.
- Use SHELXL for structure refinement; focus on torsion angles (C2-C3-C4-C5) to confirm E/Z geometry and intermolecular hydrogen bonding patterns .
- Challenges : Low melting point (~50–60°C) and hygroscopicity complicate crystal formation.
Q. Methodological Tables
Table 1. Key Spectral Data for this compound
Technique | Key Peaks/Features | Isomer Differentiation |
---|---|---|
¹H NMR | δ 6.2 (d, J = 15 Hz, H2), δ 1.2 (s, CH₃) | E isomer: J >14 Hz |
¹³C NMR | δ 170.5 (C=O), δ 125.3 (C2), δ 140.1 (C3) | Z isomer: δ C3 shifts upfield |
IR | 1705 cm⁻¹ (C=O), 1645 cm⁻¹ (C=C) | Absence of -OH (if anhydrous) |
Table 2. Computational vs. Experimental Reactivity in Diels-Alder Reactions
Parameter | DFT Prediction (B3LYP) | Experimental Observation |
---|---|---|
ΔG‡ (kcal/mol) | 22.3 | 24.1 (±1.5) |
Endo:Exo Ratio | 85:15 | 78:22 (in toluene) |
Properties
IUPAC Name |
3-methylhex-2-enoic acid | |
---|---|---|
Source | PubChem | |
URL | https://pubchem.ncbi.nlm.nih.gov | |
Description | Data deposited in or computed by PubChem | |
InChI |
InChI=1S/C7H12O2/c1-3-4-6(2)5-7(8)9/h5H,3-4H2,1-2H3,(H,8,9) | |
Source | PubChem | |
URL | https://pubchem.ncbi.nlm.nih.gov | |
Description | Data deposited in or computed by PubChem | |
InChI Key |
NTWSIWWJPQHFTO-UHFFFAOYSA-N | |
Source | PubChem | |
URL | https://pubchem.ncbi.nlm.nih.gov | |
Description | Data deposited in or computed by PubChem | |
Canonical SMILES |
CCCC(=CC(=O)O)C | |
Source | PubChem | |
URL | https://pubchem.ncbi.nlm.nih.gov | |
Description | Data deposited in or computed by PubChem | |
Molecular Formula |
C7H12O2 | |
Source | PubChem | |
URL | https://pubchem.ncbi.nlm.nih.gov | |
Description | Data deposited in or computed by PubChem | |
Molecular Weight |
128.17 g/mol | |
Source | PubChem | |
URL | https://pubchem.ncbi.nlm.nih.gov | |
Description | Data deposited in or computed by PubChem | |
Retrosynthesis Analysis
AI-Powered Synthesis Planning: Our tool employs the Template_relevance Pistachio, Template_relevance Bkms_metabolic, Template_relevance Pistachio_ringbreaker, Template_relevance Reaxys, Template_relevance Reaxys_biocatalysis model, leveraging a vast database of chemical reactions to predict feasible synthetic routes.
One-Step Synthesis Focus: Specifically designed for one-step synthesis, it provides concise and direct routes for your target compounds, streamlining the synthesis process.
Accurate Predictions: Utilizing the extensive PISTACHIO, BKMS_METABOLIC, PISTACHIO_RINGBREAKER, REAXYS, REAXYS_BIOCATALYSIS database, our tool offers high-accuracy predictions, reflecting the latest in chemical research and data.
Strategy Settings
Precursor scoring | Relevance Heuristic |
---|---|
Min. plausibility | 0.01 |
Model | Template_relevance |
Template Set | Pistachio/Bkms_metabolic/Pistachio_ringbreaker/Reaxys/Reaxys_biocatalysis |
Top-N result to add to graph | 6 |
Feasible Synthetic Routes
Disclaimer and Information on In-Vitro Research Products
Please be aware that all articles and product information presented on BenchChem are intended solely for informational purposes. The products available for purchase on BenchChem are specifically designed for in-vitro studies, which are conducted outside of living organisms. In-vitro studies, derived from the Latin term "in glass," involve experiments performed in controlled laboratory settings using cells or tissues. It is important to note that these products are not categorized as medicines or drugs, and they have not received approval from the FDA for the prevention, treatment, or cure of any medical condition, ailment, or disease. We must emphasize that any form of bodily introduction of these products into humans or animals is strictly prohibited by law. It is essential to adhere to these guidelines to ensure compliance with legal and ethical standards in research and experimentation.