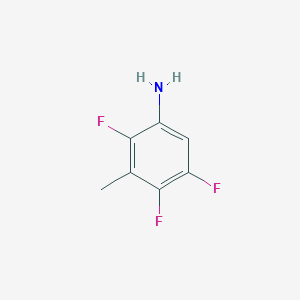
2,4,5-Trifluoro-3-methylaniline
- Click on QUICK INQUIRY to receive a quote from our team of experts.
- With the quality product at a COMPETITIVE price, you can focus more on your research.
Overview
Description
2,4,5-Trifluoro-3-methylaniline, also known as TFMA, is a chemical compound that belongs to the class of fluoroanilines. It is widely used in scientific research due to its unique properties, including its ability to act as a versatile building block in organic synthesis.
Mechanism Of Action
The mechanism of action of 2,4,5-Trifluoro-3-methylaniline is not fully understood, but it is believed to act as an inhibitor of various enzymes. It has been shown to inhibit the activity of acetylcholinesterase, which is an enzyme that plays a key role in the breakdown of the neurotransmitter acetylcholine. 2,4,5-Trifluoro-3-methylaniline has also been shown to inhibit the activity of monoamine oxidase, which is an enzyme that plays a key role in the metabolism of neurotransmitters such as dopamine, norepinephrine, and serotonin.
Biochemical And Physiological Effects
2,4,5-Trifluoro-3-methylaniline has been shown to exhibit various biochemical and physiological effects. It has been shown to exhibit potent antifungal activity against various fungi, including Candida albicans, Aspergillus niger, and Trichophyton mentagrophytes. 2,4,5-Trifluoro-3-methylaniline has also been shown to exhibit potent antibacterial activity against various bacteria, including Staphylococcus aureus, Escherichia coli, and Pseudomonas aeruginosa.
Advantages And Limitations For Lab Experiments
2,4,5-Trifluoro-3-methylaniline has several advantages for lab experiments. It is a stable compound that can be easily synthesized in high yields with high purity. It is also a versatile building block in organic synthesis, which makes it an important intermediate in the development of new pharmaceuticals and agrochemicals. However, 2,4,5-Trifluoro-3-methylaniline has some limitations for lab experiments. It is toxic and can be hazardous if not handled properly. It also has limited solubility in water, which can make it difficult to use in certain experiments.
Future Directions
There are several future directions for the use of 2,4,5-Trifluoro-3-methylaniline in scientific research. One direction is the development of new pharmaceuticals and agrochemicals based on 2,4,5-Trifluoro-3-methylaniline and its derivatives. Another direction is the investigation of the mechanism of action of 2,4,5-Trifluoro-3-methylaniline and its derivatives to better understand their biological activities. Additionally, the development of new synthesis methods for 2,4,5-Trifluoro-3-methylaniline and its derivatives could lead to improved yields and purity. Finally, the investigation of the toxicological effects of 2,4,5-Trifluoro-3-methylaniline and its derivatives could lead to the development of safer handling procedures and the identification of potential therapeutic uses.
Scientific Research Applications
2,4,5-Trifluoro-3-methylaniline has been extensively used in scientific research due to its unique properties. It can act as a versatile building block in organic synthesis, which makes it an important intermediate in the development of new pharmaceuticals and agrochemicals. 2,4,5-Trifluoro-3-methylaniline has also been used as a starting material for the synthesis of various heterocyclic compounds, which have been shown to exhibit potent biological activities.
properties
CAS RN |
119916-26-6 |
---|---|
Product Name |
2,4,5-Trifluoro-3-methylaniline |
Molecular Formula |
C7H6F3N |
Molecular Weight |
161.12 g/mol |
IUPAC Name |
2,4,5-trifluoro-3-methylaniline |
InChI |
InChI=1S/C7H6F3N/c1-3-6(9)4(8)2-5(11)7(3)10/h2H,11H2,1H3 |
InChI Key |
IQAXTTBBFAQERT-UHFFFAOYSA-N |
SMILES |
CC1=C(C(=CC(=C1F)F)N)F |
Canonical SMILES |
CC1=C(C(=CC(=C1F)F)N)F |
synonyms |
Benzenamine, 2,4,5-trifluoro-3-methyl- (9CI) |
Origin of Product |
United States |
Synthesis routes and methods
Procedure details
Disclaimer and Information on In-Vitro Research Products
Please be aware that all articles and product information presented on BenchChem are intended solely for informational purposes. The products available for purchase on BenchChem are specifically designed for in-vitro studies, which are conducted outside of living organisms. In-vitro studies, derived from the Latin term "in glass," involve experiments performed in controlled laboratory settings using cells or tissues. It is important to note that these products are not categorized as medicines or drugs, and they have not received approval from the FDA for the prevention, treatment, or cure of any medical condition, ailment, or disease. We must emphasize that any form of bodily introduction of these products into humans or animals is strictly prohibited by law. It is essential to adhere to these guidelines to ensure compliance with legal and ethical standards in research and experimentation.