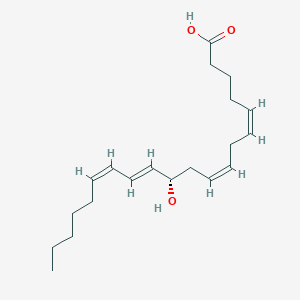
(5Z,8Z,11S,12E,14Z)-11-hydroxyicosa-5,8,12,14-tetraenoic acid
Overview
Description
Synthesis Analysis
The synthesis of hydroxyeicosa-tetraenoic acids involves the oxidation of arachidonic acid. For example, 11-hydroperoxy-eicosa-5,8,12,14-tetraenoic acid (11-HPETE) is prepared by singlet oxygen oxidation of arachidonic acid, which upon reduction forms 11-hydroxy-eicosa-5,8,12,14-tetraenoic acid (11-HETE) (Porter et al., 1980). This process highlights the enzymatic and chemical pathways leading to the formation of hydroxyeicosa-tetraenoic acids.
Molecular Structure Analysis
The molecular structure of hydroxyeicosa-tetraenoic acids is characterized by their polyunsaturated nature with specific geometric configurations (cis and trans) of the double bonds. These structures influence their biological functions and interactions with enzymes. For instance, the presence of hydroxyl groups and their positions significantly affect the molecule's activity and its role in biological pathways (Kitamura et al., 1988).
Chemical Reactions and Properties
Hydroxyeicosa-tetraenoic acids undergo various chemical reactions, including oxidation and reduction processes, that alter their structure and biological activity. The transformation of 11-HPETE to 11-HETE by prostaglandin endoperoxide synthetase demonstrates the complexity of these reactions (Porter et al., 1980). These reactions are crucial for producing different metabolites with varied biological functions.
Physical Properties Analysis
The physical properties of hydroxyeicosa-tetraenoic acids, such as solubility, melting point, and boiling point, are determined by their molecular structure. The presence of hydroxyl groups and the polyunsaturated nature of the fatty acid chain influence these properties, affecting their biological availability and function.
Chemical Properties Analysis
Hydroxyeicosa-tetraenoic acids exhibit specific chemical properties, including reactivity with various enzymes and participation in lipid signaling pathways. Their interactions with enzymes like lipoxygenases and cytochrome P450s play essential roles in their conversion to other bioactive metabolites, highlighting their importance in physiological and pathophysiological processes (Miki et al., 1989).
Scientific Research Applications
The synthesis of this acid and its racemic isomers may aid in studying oxidative stress mechanisms induced by heptynyl-lithium (Russell & Pabon, 1982).
HETE analog 1, a derivative, has vasoconstrictive and antiproliferative effects, which are crucial for future eicosanoid research (Pfister, Klimko, & Conrow, 2016).
It can be used to synthesize 11,12-leukotriene A4, a spasmogenic compound, and its diastereomers (Kitamura et al., 1988).
This acid helps in studying the metabolic fate of conjugated linoleic acid isomers (Loreau, Chardigny, Sebedio, & Nöel, 2003).
Radioactively labelled azido compounds of this acid can serve as photo-affinity probes in eicosanoid biosynthesis research (Romanov et al., 2004).
Starfish oocytes synthesize an allene oxide derivative, involved in their maturation (Brash et al., 1991).
12-HETE and 12-HPETE, derivatives of this acid, stimulate calcium release in human neutrophils (Reynaud & Pace-Asciak, 1997).
properties
IUPAC Name |
(5Z,8Z,11S,12E,14Z)-11-hydroxyicosa-5,8,12,14-tetraenoic acid | |
---|---|---|
Source | PubChem | |
URL | https://pubchem.ncbi.nlm.nih.gov | |
Description | Data deposited in or computed by PubChem | |
InChI |
InChI=1S/C20H32O3/c1-2-3-4-5-7-10-13-16-19(21)17-14-11-8-6-9-12-15-18-20(22)23/h6-7,9-11,13-14,16,19,21H,2-5,8,12,15,17-18H2,1H3,(H,22,23)/b9-6-,10-7-,14-11-,16-13+/t19-/m1/s1 | |
Source | PubChem | |
URL | https://pubchem.ncbi.nlm.nih.gov | |
Description | Data deposited in or computed by PubChem | |
InChI Key |
GCZRCCHPLVMMJE-YZGNWCGPSA-N | |
Source | PubChem | |
URL | https://pubchem.ncbi.nlm.nih.gov | |
Description | Data deposited in or computed by PubChem | |
Canonical SMILES |
CCCCCC=CC=CC(CC=CCC=CCCCC(=O)O)O | |
Source | PubChem | |
URL | https://pubchem.ncbi.nlm.nih.gov | |
Description | Data deposited in or computed by PubChem | |
Isomeric SMILES |
CCCCC/C=C\C=C\[C@H](C/C=C\C/C=C\CCCC(=O)O)O | |
Source | PubChem | |
URL | https://pubchem.ncbi.nlm.nih.gov | |
Description | Data deposited in or computed by PubChem | |
Molecular Formula |
C20H32O3 | |
Source | PubChem | |
URL | https://pubchem.ncbi.nlm.nih.gov | |
Description | Data deposited in or computed by PubChem | |
Molecular Weight |
320.5 g/mol | |
Source | PubChem | |
URL | https://pubchem.ncbi.nlm.nih.gov | |
Description | Data deposited in or computed by PubChem | |
Product Name |
(5Z,8Z,11S,12E,14Z)-11-hydroxyicosa-5,8,12,14-tetraenoic acid |
Retrosynthesis Analysis
AI-Powered Synthesis Planning: Our tool employs the Template_relevance Pistachio, Template_relevance Bkms_metabolic, Template_relevance Pistachio_ringbreaker, Template_relevance Reaxys, Template_relevance Reaxys_biocatalysis model, leveraging a vast database of chemical reactions to predict feasible synthetic routes.
One-Step Synthesis Focus: Specifically designed for one-step synthesis, it provides concise and direct routes for your target compounds, streamlining the synthesis process.
Accurate Predictions: Utilizing the extensive PISTACHIO, BKMS_METABOLIC, PISTACHIO_RINGBREAKER, REAXYS, REAXYS_BIOCATALYSIS database, our tool offers high-accuracy predictions, reflecting the latest in chemical research and data.
Strategy Settings
Precursor scoring | Relevance Heuristic |
---|---|
Min. plausibility | 0.01 |
Model | Template_relevance |
Template Set | Pistachio/Bkms_metabolic/Pistachio_ringbreaker/Reaxys/Reaxys_biocatalysis |
Top-N result to add to graph | 6 |
Feasible Synthetic Routes
Citations
Disclaimer and Information on In-Vitro Research Products
Please be aware that all articles and product information presented on BenchChem are intended solely for informational purposes. The products available for purchase on BenchChem are specifically designed for in-vitro studies, which are conducted outside of living organisms. In-vitro studies, derived from the Latin term "in glass," involve experiments performed in controlled laboratory settings using cells or tissues. It is important to note that these products are not categorized as medicines or drugs, and they have not received approval from the FDA for the prevention, treatment, or cure of any medical condition, ailment, or disease. We must emphasize that any form of bodily introduction of these products into humans or animals is strictly prohibited by law. It is essential to adhere to these guidelines to ensure compliance with legal and ethical standards in research and experimentation.