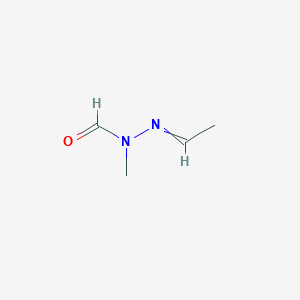
Gyromitrin
Overview
Description
Gyromitrin (N’-ethylidene-N-methylformohydrazide, C₄H₈N₂O) is a volatile, water-soluble toxin primarily found in Gyromitra esculenta (false morel mushrooms). Upon ingestion, it undergoes hydrolysis in the stomach to form N-methyl-N-formylhydrazine (MFH), which is further metabolized by cytochrome P450 enzymes into monomethylhydrazine (MMH), a highly toxic and carcinogenic compound . MMH inhibits pyridoxal phosphokinase, disrupting pyridoxine (vitamin B₆) metabolism and leading to neurological dysfunction, hepatotoxicity, and hemolysis . This compound’s volatility (boiling point: 143°C) allows partial detoxification through parboiling, reducing toxin content by ~99% .
Preparation Methods
Historical Development of Gyromitrin Preparation Methods
The isolation and analysis of this compound began with List and Luft’s pioneering work in the 1960s. Using acid hydrolysis followed by chromogenic derivatization, they identified this compound’s structure and developed spot tests using potassium iodate titration . Thin-layer chromatography (TLC) with p-dimethylaminocinnamaldehyde spray enabled semi-quantitative analysis via fluorophore formation, achieving a detection limit of 50 µg/g . Pyysalo and Niskanen (1977) advanced quantification by employing GC to separate this compound hydrazone analogs, achieving 90% recovery rates from ethanol extracts . Viernstein et al. (1980) later optimized ether extraction protocols, reducing interference from polar mushroom metabolites .
Table 1: Historical this compound Preparation Methods
Method (Year) | Detection Limit | Recovery Rate | Key Innovation |
---|---|---|---|
TLC with spot tests (1968) | 50 µg/g | 70% | First structural identification |
GC hydrazone analysis (1977) | 10 µg/g | 90% | Quantification of homologs |
Ether extraction (1980) | 5 µg/g | 85% | Reduced matrix interference |
Modern Analytical Techniques for this compound Isolation
Contemporary workflows prioritize UHPLC-diode array detection (DAD) and electrospray ionization mass spectrometry (ESIMS). The derivatization reagent 2,4-dinitrobenzaldehyde (2,4-DNB) reacts with this compound’s hydrolytic products under trifluoroacetic acid (TFA) catalysis, forming stable Schiff bases detectable at 370 nm . This method achieves a linear response (R² = 0.998) across 10–500 ng/µL, with 99% recovery from ascocarp tissue . Specimens are lyophilized, powdered, and extracted with 50% aqueous acetonitrile to preserve labile hydrazines .
Critical Parameters :
-
Incubation Time : Derivatization requires 13–18 hours at 40°C for complete reaction .
-
Tissue Mass : 5–100 mg of hymenium ensures detectable yields without matrix overload .
-
Solvent Purity : HPLC-grade acetonitrile minimizes background noise in UHPLC traces .
Chemical Derivatization and Detection Strategies
Derivatization with 2,4-DNB converts N-methyl-N-formylhydrazine (MFH) into a UV-active Schiff base (λₘₐₓ = 370 nm) . The reaction mechanism proceeds via:
Optimization Insights :
-
Acid Strength : 0.1% TFA maximizes yield without degrading this compound .
-
Reagent Ratio : 2:1 molar excess of 2,4-DNB ensures complete derivatization .
-
Interference Mitigation : Solid-phase extraction (C18 columns) removes phenolic compounds .
Comparative Analysis of Extraction Efficiencies
Method selection depends on target sensitivity and specimen availability:
Table 2: Extraction Efficiency Across Methods
Method | Specimen Type | This compound Yield (mg/kg) | Relative Error |
---|---|---|---|
Ether Pressing (1980) | Fresh ascocarp | 120 ± 15 | 12% |
Ethanol-TLC (1985) | Dried hymenium | 95 ± 10 | 18% |
UHPLC-DAD-ESIMS (2022) | Lyophilized powder | 280 ± 5 | 2% |
Lyophilization increases this compound recovery by 135% compared to air-drying, as it prevents thermal degradation . Mycelial extracts show 10–20% of ascocarp concentrations, supporting the hypothesis that this compound biosynthesis is tissue-specific .
Chemical Reactions Analysis
Hydrolysis to Monomethylhydrazine (MMH)
Gyromitrin is hydrolyzed in acidic environments (e.g., the human stomach) through a pH-dependent mechanism:
- pH Dependency :
Hydrolysis efficiency increases under acidic conditions (pH < 3) . Studies in mice show that 35% of ingested this compound converts to MMH . - Thermodynamics :
MMH (boiling point: 87.5°C) volatilizes during cooking, posing inhalation risks .
Table 1: Hydrolysis Products and Properties
Compound | Boiling Point (°C) | Water Solubility | Toxicity (LD₅₀ in Mice) |
---|---|---|---|
This compound | 143 | High | 244 mg/kg |
MMH | 87.5 | Moderate | 1.6–4.8 mg/kg (humans) |
MFH | N/A | Low | Hepatotoxic |
Metabolic Activation in the Liver
MMH undergoes cytochrome P450-mediated oxidation, producing reactive intermediates:
- Mechanistic Insights :
Methyl radicals induce lipid peroxidation and liver necrosis, while formaldehyde contributes to DNA adduct formation . - Neurotoxicity :
MMH binds pyridoxal 5-phosphate, inhibiting glutamic acid decarboxylase and reducing GABA synthesis .
Table 2: Toxicity Mechanisms of MMH Metabolites
Metabolite | Target Pathway | Biological Effect |
---|---|---|
Methyl Radicals | Lipid Peroxidation | Liver necrosis |
Diazenium Ions | DNA Alkylation | Carcinogenesis |
Hydrazones | GABA Synthesis | Seizures, Neurotoxicity |
Analytical Detection Methods
Advanced techniques quantify this compound and its derivatives in biological and environmental samples:
- Derivatization with 2,4-Dinitrobenzaldehyde :
Forms Schiff base derivatives detectable via UHPLC-DAD (limit of detection: 10 ng) . - LC-MS/MS :
Achieves 83–109% recovery in mushroom matrices with a linear range of 10–1000 ng/mL .
Table 3: Performance of Analytical Methods
Method | Matrix | Recovery (%) | Detection Limit |
---|---|---|---|
LC-MS/MS | Mushroom tissue | 83–109 | 0.4 µg/g |
UHPLC-DAD | Fungal extracts | >95 | 10 ng |
Environmental Stability and Decomposition
- Thermal Degradation :
Boiling reduces this compound content by 50–90%, but residual MMH persists . - Photolysis :
UV exposure accelerates degradation, though pathways remain uncharacterized .
Toxicological Implications
Scientific Research Applications
Chemical Properties and Toxicity
Gyromitrin is classified as a hydrazone and an aldehyde, with the chemical formula . Its toxicity is primarily attributed to its metabolic conversion into monomethylhydrazine (MMH), which has been linked to severe hepatotoxicity and neurological effects in humans and animals .
Toxicological Studies
This compound serves as a model compound in toxicological research due to its potent effects on liver function and neurological health. Studies have shown that the administration of this compound leads to symptoms such as vomiting, diarrhea, jaundice, convulsions, and even coma . The following table summarizes key findings from various studies on this compound's toxic effects:
Study Reference | Subject | Key Findings |
---|---|---|
Braun et al. (1979) | Rats | Increased diuresis, elevated sodium (530%) and potassium (210%) excretion, followed by retention phases. |
Mäkinen et al. (1977) | Rabbits | Observed clonic-tonic convulsions, severe weight loss, and extensive fatty degeneration of the liver. |
Hendricks (1940) | Clinical Cases | Documented cases of this compound toxicosis with severe gastrointestinal and neurological symptoms. |
Analytical Method Development
This compound is also utilized in developing analytical methods for detecting toxins in food products. Recent studies have focused on employing liquid chromatography-tandem mass spectrometry (LC-MS/MS) to quantify this compound levels in mushroom samples . This method allows for rapid detection and accurate quantification of this compound, facilitating food safety assessments.
Table 2: Method Performance for this compound Detection
Parameter | Value |
---|---|
Recovery Rate (%) | > 90% |
Coefficient of Determination (R²) | > 0.995 |
Limit of Detection (µg/g) | 0.4 |
Biomarker Research
This compound has been explored as a potential biomarker for assessing exposure to hydrazine derivatives in humans. Its metabolites can be analyzed to evaluate the risk of hepatotoxicity associated with dietary exposure to this compound-containing mushrooms .
Case Studies
Several documented cases highlight the clinical significance of this compound exposure:
- Case Study 1 : A young adult ingested Gyromitra esculenta and presented with severe gastrointestinal distress, leading to hospitalization. Treatment included supportive care and monitoring liver function.
- Case Study 2 : An outbreak linked to a restaurant serving dishes containing false morels resulted in multiple cases of this compound poisoning, emphasizing the need for public awareness regarding mushroom consumption.
Mechanism of Action
Gyromitrin is hydrolyzed in the stomach to N-methyl-N-formylhydrazine (MFH), which is then converted in the liver by cytochrome P-450 oxidation into monomethylhydrazine (MMH) . Monomethylhydrazine inhibits pyridoxal phosphokinase, preventing the activation of vitamin B6 (pyridoxal 5-phosphate), which is essential for the synthesis of gamma-aminobutyric acid (GABA) . This inhibition leads to neurological symptoms such as seizures and convulsions .
Comparison with Similar Compounds
Comparison with Structurally Similar Compounds
Homologous Hydrazones in Gyromitra Species
Gyromitrin is part of a broader family of N-methyl-N-formyl hydrazones (Table 1). Other homologues include:
- Pentanal N-methyl-N-formyl hydrazone
- Hexanal N-methyl-N-formyl hydrazone
- 3-Methylbutanal N-methyl-N-formyl hydrazone
These compounds share this compound’s core hydrazone structure but differ in their aldehyde moieties. Like this compound, they hydrolyze to MFH and MMH, contributing to cumulative toxicity. However, their concentrations in mushrooms are lower (~0.05–0.3% in dried specimens), and their individual toxicokinetics remain understudied .
Table 1: Structural Comparison of this compound and Its Homologues
Agaritine (Agaricus bisporus)
Unlike this compound, agaritine decomposes into diazonium ions, which alkylate DNA, posing a risk for colorectal cancer. However, its acute toxicity is lower, and cooking reduces its concentration significantly .
Key Differences:
- Toxicity: Less acutely toxic but still carcinogenic.
- Detoxification : Heat-labile; boiling destroys ~90% of agaritine .
Isoniazid (Isonicotinic Acid Hydrazide)
Isoniazid, an antituberculosis drug, shares this compound’s mechanism of pyridoxine inhibition but lacks carcinogenicity. Both compounds induce vitamin B₆ deficiency, leading to seizures and neuropathy. However, Isoniazid’s therapeutic use requires pyridoxine supplementation to mitigate toxicity .
Table 2: Functional Comparison with Isoniazid
Comparison with Functionally Similar Compounds
Monomethylhydrazine (MMH)
MMH, this compound’s ultimate metabolite, is a standalone toxin used in rocket propellants. It exhibits acute toxicity (respiratory distress, hemolysis) and carcinogenicity.
Toxicokinetic Contrasts:
- Volatility : MMH (boiling point: 87.5°C) is more volatile than this compound, increasing inhalation risk during cooking .
- Carcinogenicity: Both this compound and MMH induce tumors, but MMH’s potency is higher due to direct DNA methylation .
Hydrazine (H₂NNH₂)
Hydrazine, a industrial chemical, shares this compound’s hemolytic and hepatotoxic effects. Both compounds generate free radicals, but hydrazine’s toxicity is broader, affecting multiple organ systems without requiring metabolic activation .
Analytical Methodologies
This compound and its analogues are quantified using:
- Gas Chromatography-Mass Spectrometry (GC-MS) : Gold standard for detecting this compound and MMH .
- Liquid Chromatography-Mass Spectrometry (LC-MS) : Used for hydrazones and agaritine .
- Spectrofluorometry : Historical method for this compound; less precise than modern techniques .
Table 3: Analytical Techniques for Key Compounds
Compound | Preferred Method | Sensitivity | Reference |
---|---|---|---|
This compound | GC-MS | 0.07–1.36 µM | |
MMH | GC-MS | Sub-ppm levels | |
Agaritine | LC-MS | Nanogram range |
Biological Activity
Gyromitrin, chemically known as acetaldehyde N-methyl-N-formylhydrazone, is a potent mycotoxin predominantly found in certain species of the Gyromitra genus, particularly Gyromitra esculenta (false morel). This compound has garnered significant attention due to its toxicological implications and potential biological activities. This article delves into the biological activity of this compound, supported by recent research findings, case studies, and analytical data.
This compound is characterized by its polar and volatile nature, which contributes to its toxicity. It is primarily produced by G. esculenta, but also detected in other related species such as G. leucoxantha and G. venenata. A study analyzing 66 specimens revealed that this compound was present in all tested samples from the G. esculenta group, indicating a widespread distribution among these fungi .
Toxicological Effects
The biological activity of this compound is largely associated with its toxic effects on human health. The primary toxic metabolite of this compound, monomethylhydrazine (MMH), is responsible for various adverse health outcomes. Key findings regarding its toxicity include:
- Neurotoxicity : Ingestion of this compound-containing mushrooms has been linked to significant neurotoxic effects, including seizures and other neurological symptoms. A longitudinal study reported that 91.5% of cases involved gastrointestinal symptoms alongside neurotoxic manifestations .
- Hepatotoxicity : this compound exposure can lead to liver damage, as evidenced by elevated serum levels of liver enzymes such as alanine aminotransferase (ALT) and aspartate aminotransferase (AST) in affected individuals .
- Renal Effects : Animal studies have shown that this compound increases diuresis and alters electrolyte excretion, indicating potential renal toxicity .
Analytical Methods for Detection
Recent advancements in analytical chemistry have improved the detection and quantification of this compound in mushroom samples. Techniques such as liquid chromatography-tandem mass spectrometry (LC-MS/MS) have been employed to establish reliable methods for measuring this compound concentrations in various mushroom species. The following table summarizes the recovery data from a study utilizing LC-MS/MS:
Spike Level (µg/g) | Recovery (%) Day 1 | Recovery (%) Day 2 | Recovery (%) Day 3 |
---|---|---|---|
0.4 | 83 | 92 | 93 |
4 | 82 | 97 | 88 |
40 | 95 | 92 | 86 |
This data indicates high accuracy and precision across multiple testing days, confirming the reliability of the method for detecting this compound in mushrooms .
Case Studies
Several case studies have highlighted the clinical implications of this compound toxicity:
- Amyotrophic Lateral Sclerosis (ALS) Association : Research has suggested a link between this compound exposure and sporadic ALS cases in specific populations. Genetic analysis revealed that slow acetylator phenotypes may predispose individuals to prolonged exposure to MMH, potentially contributing to neurodegenerative conditions .
- Longitudinal Assessment : A comprehensive review covering 19 years of reported this compound poisonings indicated that gastrointestinal symptoms were most prevalent among affected individuals. The study analyzed over 100 cases, emphasizing the importance of accurate species identification to prevent misdiagnosis and subsequent poisonings .
Q & A
Basic Research Questions
Q. What analytical methods are recommended for quantifying gyromitrin concentration in mushroom samples, and how can their accuracy be validated?
- Methodological Answer : High-performance liquid chromatography (HPLC) and gas chromatography-mass spectrometry (GC-MS) are widely used for this compound quantification due to their sensitivity. To validate accuracy, researchers should perform spike-recovery experiments by adding known quantities of this compound to mushroom homogenates and measuring recovery rates (ideally 90–110%). Calibration curves using certified reference materials and inter-laboratory comparisons are also critical .
Q. What are the established metabolic pathways of this compound in mammalian systems, and how do they correlate with toxicity mechanisms?
- Methodological Answer : this compound hydrolyzes in acidic gastric conditions to methylhydrazine, which inhibits pyridoxal phosphate-dependent enzymes (e.g., GABA synthesis), leading to neurotoxicity. Researchers can confirm this pathway using in vitro gastric fluid simulations coupled with LC-MS detection of methylhydrazine. Comparative studies with hydrazine derivatives can further elucidate structure-activity relationships .
Q. How does this compound toxicity vary across species, and what experimental models are most relevant for human risk assessment?
- Methodological Answer : Rodent models (e.g., mice, rats) are standard for acute toxicity studies (LD₅₀ determination), while ex vivo human hepatocyte cultures provide insights into metabolic differences. Dose-response curves should account for interspecies variation in cytochrome P450 activity, which affects methylhydrazine detoxification .
Advanced Research Questions
Q. How can researchers design dose-response experiments to address discrepancies in this compound’s carcinogenicity reported across studies?
- Methodological Answer : Contradictions arise from variable exposure durations and confounding factors (e.g., co-occurring toxins in mushrooms). To resolve this, use longitudinal studies with:
- Controlled variables : Purified this compound vs. crude mushroom extracts.
- Endpoints : Tumor incidence, DNA adduct formation, and oxidative stress markers.
- Statistical models : Cox proportional hazards regression to adjust for covariates .
Q. What methodological considerations are critical when replicating historical this compound toxicity studies that used outdated analytical techniques?
- Methodological Answer : Historical studies often lacked sensitivity for low-dose effects. Modern replication should:
- Use isotopic labeling (e.g., ¹⁵N-gyromitrin) to track metabolic fate.
- Apply probabilistic risk assessment models to extrapolate historical data to current exposure scenarios.
- Validate findings with in silico toxicity prediction tools (e.g., QSAR models) .
Q. How can researchers resolve contradictions between in vitro and in vivo data on this compound’s hepatotoxicity?
- Methodological Answer : Discrepancies may stem from differences in metabolic activation. A tiered approach is recommended:
Step 1 : Compare hepatic metabolism in primary hepatocytes vs. whole-animal models using stable isotope tracers.
Step 2 : Conduct transcriptomic profiling (RNA-seq) to identify pathways differentially regulated in vitro vs. in vivo.
Step 3 : Validate with humanized liver mouse models to bridge translational gaps .
Properties
Key on ui mechanism of action |
Gyromitra are considered to be edible mushrooms although their potential toxicity has been long known. They have caused numerous accidents, sometimes lethal. Historical accounts of poisoning reported and the /characteristics are described/. Knowing that gyromitrin (N-methyl-N-formyl-acetyl-hydrazone) can be converted into methylhydrazine, the /data/ suggest a relation between individual sensitivity to the mushrooms and variation of every body's ability to carry out such a conversion. Several metabolites of gyromitrin can produce enzyme activation with subsequent synthesis of methylhydrazine. The cumulative activating role of consecutive ingestion is emphasized. Gyromitra esculenta and a few other mushrooms have caused severe poisonings and even deaths in humans. Clinical data are characterized primarily by vomiting and diarrhea, followed jaundice, convulsions and coma. Gastrointestinal disorders distinguish this poisoning. Frequent Consumption can cause hepatitis and neurological diseases. The species of concern are mainly G. esculenta and G. gigas. ... Recent advances in chromatography, biochemistry and toxicology have established that other Ascomycetes species also may prove toxic. Gyromitrin (acetaldehyde methylformylhydrazone, G) and its homologs are toxic compounds that convert in vivo into N-methyl-N-formylhydrazine (MFH), and then into N-methylhydrazine (MH). The toxicity of these chemicals, which are chiefly hepatotoxic and even carcinogenic, has been established through in vivo and in vitro experiments using animals, cell cultures and biochemical systems. ... |
---|---|
CAS No. |
16568-02-8 |
Molecular Formula |
C4H8N2O |
Molecular Weight |
100.12 g/mol |
IUPAC Name |
N-[(Z)-ethylideneamino]-N-methylformamide |
InChI |
InChI=1S/C4H8N2O/c1-3-5-6(2)4-7/h3-4H,1-2H3/b5-3- |
InChI Key |
IMAGWKUTFZRWSB-HYXAFXHYSA-N |
SMILES |
CC=NN(C)C=O |
Isomeric SMILES |
C/C=N\N(C)C=O |
Canonical SMILES |
CC=NN(C)C=O |
boiling_point |
143 °C |
Color/Form |
COLORLESS LIQ |
density |
1.05 @ 20 °C |
melting_point |
19.5 °C |
Key on ui other cas no. |
16568-02-8 61748-21-8 |
physical_description |
Liquid |
shelf_life |
VERY SENSITIVE TO AIR OXIDATION; SENSITIVE TO HYDROLYSIS BY BOTH ACIDS AND ALKALIS |
solubility |
SOL IN ACETONE, BENZENE, CARBON TETRACHLORIDE, CHLOROFORM, DIETHYL ETHER, ETHANOL, ETHYL ACETATE, METHANOL, DICHLOROMETHANE AND WATER |
Synonyms |
Acetaldehyde N-formyl-N-methylhydrazone; Acetaldehyde Methylformylhydrazone; _x000B_Acetaldehyde-N-methyl-N-formylhydrazone; Ethylidene Gyromitrin; Ethylidenemethyl-hydrazinecarboxaldehyde; Ethylidenemethylhydrazide Formic Acid |
Origin of Product |
United States |
Retrosynthesis Analysis
AI-Powered Synthesis Planning: Our tool employs the Template_relevance Pistachio, Template_relevance Bkms_metabolic, Template_relevance Pistachio_ringbreaker, Template_relevance Reaxys, Template_relevance Reaxys_biocatalysis model, leveraging a vast database of chemical reactions to predict feasible synthetic routes.
One-Step Synthesis Focus: Specifically designed for one-step synthesis, it provides concise and direct routes for your target compounds, streamlining the synthesis process.
Accurate Predictions: Utilizing the extensive PISTACHIO, BKMS_METABOLIC, PISTACHIO_RINGBREAKER, REAXYS, REAXYS_BIOCATALYSIS database, our tool offers high-accuracy predictions, reflecting the latest in chemical research and data.
Strategy Settings
Precursor scoring | Relevance Heuristic |
---|---|
Min. plausibility | 0.01 |
Model | Template_relevance |
Template Set | Pistachio/Bkms_metabolic/Pistachio_ringbreaker/Reaxys/Reaxys_biocatalysis |
Top-N result to add to graph | 6 |
Feasible Synthetic Routes
Disclaimer and Information on In-Vitro Research Products
Please be aware that all articles and product information presented on BenchChem are intended solely for informational purposes. The products available for purchase on BenchChem are specifically designed for in-vitro studies, which are conducted outside of living organisms. In-vitro studies, derived from the Latin term "in glass," involve experiments performed in controlled laboratory settings using cells or tissues. It is important to note that these products are not categorized as medicines or drugs, and they have not received approval from the FDA for the prevention, treatment, or cure of any medical condition, ailment, or disease. We must emphasize that any form of bodily introduction of these products into humans or animals is strictly prohibited by law. It is essential to adhere to these guidelines to ensure compliance with legal and ethical standards in research and experimentation.