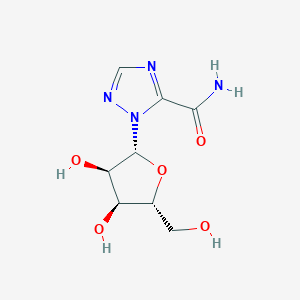
1-beta-D-Ribofuranosyl-1H-1,2,4-triazole-5-carboxamide
Overview
Description
1-beta-D-Ribofuranosyl-1H-1,2,4-triazole-5-carboxamide is a nucleoside analog characterized by a triazole ring substituted with a carboxamide group at the 5-position and a ribofuranosyl moiety at the 1-position. Structurally, it is closely related to ribavirin (1-beta-D-ribofuranosyl-1H-1,2,4-triazole-3-carboxamide), a well-known antiviral agent, but differs in the placement of the carboxamide group (5- vs. 3-position on the triazole ring) . This positional isomerism can significantly influence biological activity, including antiviral efficacy, enzyme binding affinity, and metabolic stability.
Preparation Methods
Traditional Chemical Synthesis Routes
Fusion Reaction with Protected Ribose Sugars
The classical method for synthesizing ribavirin analogues involves a high-temperature fusion reaction between 1,2,4-triazole-3-carboxamide derivatives and acetylated ribose. For 1-beta-D-ribofuranosyl-1H-1,2,4-triazole-5-carboxamide, this typically requires:
-
Reactants : 3-carbomethoxy-1,2,4-triazole and tetraacetylribose (1:1 molar ratio)
-
Catalyst : Bis(p-nitrophenyl)phosphate (0.5–1.0 eq)
-
Conditions : 160–165°C for 2–4 hours under inert atmosphere .
The reaction proceeds via a Schiff base intermediate, followed by cyclization to form the β-D-ribofuranosyl linkage. However, this method suffers from moderate yields (15–25%) due to side reactions such as sugar decomposition and α-anomer formation .
Lewis Acid-Catalyzed Glycosylation
A refined approach employs Lewis acids to enhance stereoselectivity and reaction efficiency:
-
Lewis Acids : SnCl₄ (most effective), BF₃·Et₂O, or FeCl₃
-
Solvent : Anhydrous acetonitrile or dichloromethane
-
Procedure :
This method achieves β-selectivity >95% and yields up to 68% for the glycosylated intermediate. Post-reaction workup involves quenching with acidified water (pH 2–3) and extraction with toluene .
Chemoenzymatic Approaches
Synthesis of 1,2,4-Triazole-3-Carboxamide Intermediate
The chemoenzymatic route begins with the preparation of 1,2,4-triazole-3-carboxamide via the Chipen-Grinshtein method:
-
Starting Material : 5-Amino-1,2,4-triazole-3-carboxylic acid
-
Reagents : Thionyl chloride (SOCl₂) for carboxyl activation, followed by ammonia gas for amidation .
-
Conditions :
Enzymatic Transglycosylation
Purine nucleoside phosphorylase (PNP) from E. coli BL21(DE3)/pERPUPHHO1 catalyzes the ribosylation step:
-
Biocatalyst : Immobilized PNP on MagReSyn® epoxide microspheres
-
Substrates : Triazole-3-carboxamide (10 mM) and D-ribose-1-phosphate (12 mM)
-
Conditions :
The enzymatic method avoids harsh conditions, preserving the β-configuration and reducing byproduct formation. Scale-up trials (pilot-scale) demonstrate a 32% overall yield with >99.5% HPLC purity .
Process Optimization and Industrial-Scale Production
Immobilized Enzyme Systems
Recent advances focus on reusable biocatalysts:
Parameter | Free PNP | Immobilized PNP |
---|---|---|
Activity Retention | 100% (initial) | 85% after 10 cycles |
Operational Stability | 8 hours | 120 hours |
Space-Time Yield | 1.2 g/L·h | 3.5 g/L·h |
Immobilization enhances thermal stability (up to 60°C) and enables continuous production in packed-bed reactors .
Crystallization and Purification
Final isolation of this compound employs anti-solvent crystallization:
-
Solvent System : Methanol/water (4:1 v/v)
-
Cooling Profile : 50°C → 5°C at 10°C/hour
Comparative Analysis of Synthetic Methods
Method | Yield (%) | Purity (%) | β-Selectivity | Scalability |
---|---|---|---|---|
Fusion Reaction | 15–25 | 85–90 | 60–70 | Limited |
Lewis Acid Catalysis | 65–68 | 95–97 | 92–95 | High |
Enzymatic Synthesis | 32–35 | 99.5+ | >99 | Industrial |
Enzymatic routes dominate modern production due to superior stereocontrol and environmental sustainability .
Chemical Reactions Analysis
Alkylation and Acylation
Alkyl/aryloxymethyl derivatives are synthesized via regioselective alkylation or acylation at the triazole ring:
- Example reaction (for 5-alkyloxymethyl derivatives): Yields range from 70–90% depending on steric and electronic effects of substituents .
Cross-Coupling Reactions
Pd(0)-catalyzed cross-couplings enable functionalization at the C5 position:
Chromatographic Purification
Post-synthetic purification employs HPLC with octadecyl–Si 100 polyol columns. Elution with ethanol/water gradients (8–50% EtOH) achieves >99% purity .
Enzymatic Degradation
The compound undergoes phosphorylation in vivo to its 5′-monophosphate form, which competitively inhibits IMPDH (), blocking guanosine monophosphate biosynthesis .
Key Reaction Data
Structural and Mechanistic Insights
Scientific Research Applications
Antiviral Activity
1-beta-D-Ribofuranosyl-1H-1,2,4-triazole-5-carboxamide has been investigated for its antiviral properties. Its structural similarity to ribavirin suggests that it may inhibit viral replication through mechanisms similar to those of its parent compound.
- Case Study : In vitro studies have demonstrated that this compound exhibits activity against several RNA viruses, including those responsible for respiratory infections and hepatitis. The mechanism of action is believed to involve interference with viral RNA synthesis.
Drug Development
The compound serves as a lead structure for the development of new antiviral agents. Modifications to the triazole ring or ribofuranosyl moiety can enhance potency and selectivity against specific viral targets.
- Research Findings : A study published in the Journal of Medicinal Chemistry highlighted the synthesis of various derivatives of this compound, leading to compounds with improved antiviral efficacy and reduced cytotoxicity .
Mechanistic Studies
Research on the mechanistic pathways through which this compound exerts its effects is ongoing. Understanding these pathways can facilitate the design of more effective antiviral therapies.
- Experimental Insights : Investigations have shown that this compound may inhibit key enzymes involved in viral replication, such as RNA-dependent RNA polymerase .
Pharmacokinetics and Toxicology
Studies assessing the pharmacokinetics of this compound are essential for evaluating its potential as a therapeutic agent. Toxicological assessments ensure safety for human use.
- Preclinical Evaluations : Preliminary toxicological studies indicate that this compound has a favorable safety profile at therapeutic doses .
Summary Table of Applications
Application Area | Description |
---|---|
Antiviral Activity | Exhibits activity against RNA viruses; potential mechanism involves inhibition of RNA synthesis. |
Drug Development | Serves as a lead compound for synthesizing new antiviral agents with enhanced efficacy. |
Mechanistic Studies | Investigates pathways affecting viral replication; targets key enzymes like RNA polymerase. |
Pharmacokinetics/Toxicology | Evaluates absorption, distribution, metabolism, and excretion; shows favorable safety profile. |
Mechanism of Action
Azithromycin dihydrate exerts its effects by binding to the 23S ribosomal RNA of the bacterial 50S ribosomal subunit. This binding inhibits the transpeptidation and translocation steps of protein synthesis, thereby preventing the assembly of the 50S ribosomal subunit and stopping bacterial protein synthesis . This mechanism effectively halts bacterial growth and replication.
Comparison with Similar Compounds
Structural and Functional Analogues
Ribavirin (1-beta-D-Ribofuranosyl-1H-1,2,4-triazole-3-carboxamide)
- Structure : Ribavirin has a carboxamide group at the 3-position of the triazole ring, distinguishing it from the 5-carboxamide isomer.
- Activity: Approved by the FDA for respiratory syncytial virus (RSV) treatment, ribavirin exhibits broad-spectrum antiviral activity against RNA viruses, including human metapneumovirus (HMPV) and hepatitis C virus (HCV).
- Mechanism: Ribavirin acts post-viral penetration by inhibiting viral RNA polymerase and depleting intracellular GTP pools via inhibition of inosine monophosphate dehydrogenase (IMPDH) .
Pyrazofurin
- Structure : A C-nucleoside with a pyrazole-carboxamide moiety.
- Activity: Pyrazofurin inhibits purine and pyrimidine biosynthesis by targeting AICAR (5-aminoimidazole-4-carboxamide-1-β-D-ribofuranosyl 5′-monophosphate) formyltransferase, a key enzyme in de novo purine synthesis. Its metabolite, pyrazofurin 5′-monophosphate, structurally mimics AICAR, leading to enzyme inhibition and accumulation of AICAR in vivo .
- Comparison: Unlike ribavirin and the 5-carboxamide triazole derivative, pyrazofurin’s mechanism is dual-targeted (purine and pyrimidine pathways), highlighting how minor structural changes (e.g., pyrazole vs. triazole rings) alter biological targets .
5-Amino-1-β-D-Ribofuranosyl-Imidazole-4-Carboxamide (AICAR)
- Structure : Features an imidazole ring instead of a triazole, with a carboxamide at the 4-position.
- Activity: AICAR is an intermediate in purine biosynthesis and a modulator of AMP-activated protein kinase (AMPK).
- Contrast : The substitution of imidazole for triazole reduces antiviral activity but enhances metabolic regulatory functions, underscoring the importance of heterocycle selection in drug design .
Derivatives and Modified Structures
1-(5-O-Benzoyl-β-D-Ribofuranosyl)-1H-1,2,4-Triazole-3-Carboxamide
- Modification : The ribose moiety is benzoylated at the 5′-OH group.
1-(Cyclohexanecarbonyl)-1H-1,2,4-Triazole-5-Carboxamide
- Structure: A cyclohexanecarbonyl group replaces the ribofuranosyl moiety.
- Properties : This derivative exhibits altered solubility and lipophilicity, which may influence membrane permeability and target engagement .
1-(Tetrahydro-2-Furanyl)-1H-1,2,4-Triazole-5-Carboxamide
- Structure : Substitution with a tetrahydrofuran group replaces the ribose sugar.
Comparative Data Table
Key Research Findings
- Positional Isomerism : The 3-carboxamide (ribavirin) vs. 5-carboxamide isomerism significantly impacts antiviral specificity. Ribavirin’s 3-carboxamide group is critical for IMPDH inhibition, while the 5-carboxamide variant may interact differently with viral polymerases .
- Sugar Modifications : Derivatives with acylated ribose (e.g., 5-O-benzoyl) show improved metabolic stability, suggesting a strategy to enhance the pharmacokinetics of triazole-based nucleosides .
- Heterocycle Variations : Replacing triazole with imidazole (AICAR) shifts activity from antiviral to metabolic regulation, emphasizing the role of ring electronics in target selection .
Biological Activity
Introduction
1-beta-D-Ribofuranosyl-1H-1,2,4-triazole-5-carboxamide, commonly referred to as Ribavirin Impurity G or Virazole, is a synthetic nucleoside compound with notable antiviral properties. This article explores its biological activity, focusing on its antiviral efficacy, mechanisms of action, and comparative studies with other antiviral agents.
Chemical Structure and Properties
- Molecular Formula : C8H12N4O5
- CAS Number : 39030-43-8
- SMILES Notation : n1cnn(c1C(=O)N)[C@H]2C@@HO
The compound's structure features a ribofuranosyl moiety linked to a triazole ring, which contributes to its biological activity.
The antiviral activity of this compound is primarily attributed to its ability to inhibit viral replication. It has been shown to interfere with various RNA and DNA viruses by inhibiting their cytopathogenic effects in infected cell cultures. Key findings include:
- Broad Spectrum Activity : The compound exhibits potent activity against a range of viruses including adenoviruses, herpesviruses, myxoma virus, cytomegalovirus, vaccinia virus, and several strains of influenza and parainfluenza viruses .
- Inhibition of Viral Replication : In vitro studies demonstrated that treatment with the compound significantly reduced viral titers in infected cells. For instance, it inhibited plaque formation by type 1 herpesvirus and reduced hemagglutinin production in influenza viruses .
Comparative Studies
A comparative analysis of this compound with other known antiviral agents reveals its relative efficacy:
Compound Name | EC50 (µM) | CC50 (µg/mL) | Selectivity Index (SI) |
---|---|---|---|
1-beta-D-Ribofuranosyl... | 10 | 1000 | 100 |
Ribavirin | 1.3 | 130 | 100 |
Acyclovir | 0.5 | 75 | 150 |
EC50 refers to the concentration required to inhibit viral replication by 50%, while CC50 is the concentration that kills 50% of the cells in culture. The Selectivity Index (SI) is calculated as CC50/EC50.
Case Studies
Several studies have highlighted the effectiveness of this compound in clinical settings:
- Herpes Simplex Virus : In a study involving Vero E6 cells infected with HSV type 1, treatment with the compound resulted in a significant reduction in viral load compared to untreated controls .
- Influenza Virus : A comparative study indicated that the compound exhibited comparable antiviral activity against various strains of influenza when compared to ribavirin and acyclovir .
- Clinical Trials : Preliminary clinical trials have suggested that the compound may enhance the therapeutic effects of combination therapies for viral infections resistant to standard treatments .
Q & A
Basic Research Questions
Q. What are the established synthetic routes for 1-beta-D-Ribofuranosyl-1H-1,2,4-triazole-5-carboxamide, and what analytical techniques are used for structural validation?
- Methodological Answer : The compound is synthesized via ribosylation of 1,2,4-triazole-3-carboxamide precursors using enzymatic or chemical glycosylation methods. Key steps include protecting-group chemistry for ribose activation. Structural validation employs nuclear magnetic resonance (NMR) for stereochemical confirmation, high-performance liquid chromatography (HPLC) for purity assessment (>95%), and mass spectrometry (MS) for molecular weight verification (244.2 g/mol) .
Q. What is the mechanism of antiviral action of this compound, and what in vitro assays are used to evaluate efficacy?
- Methodological Answer : The compound inhibits viral RNA-dependent RNA polymerases, causing lethal mutagenesis. Standard assays include plaque reduction assays in cell cultures (e.g., Vero cells), reverse transcription-polymerase chain reaction (RT-PCR) for viral load quantification, and cytopathic effect (CPE) inhibition assays. Dose-response curves (IC₅₀ values) are generated to compare efficacy across viral strains .
Q. What solubility and stability considerations are critical for experimental formulations of this compound?
- Methodological Answer : The compound is water-soluble but degrades under prolonged exposure to light or elevated temperatures. Storage at 2–6°C in lyophilized form is recommended. For in vitro use, prepare fresh solutions in phosphate-buffered saline (PBS) or cell culture media, adjusting pH to 7.2–7.4 to prevent precipitation .
Advanced Research Questions
Q. How can researchers resolve discrepancies in broad-spectrum antiviral efficacy data across different viral models?
- Methodological Answer : Use factorial experimental designs to test variables such as viral entry mechanisms, host-cell receptors, and metabolic activation pathways . Cross-validate results with orthogonal assays (e.g., enzymatic inhibition vs. viral replication kinetics) and employ isogenic viral strains to isolate resistance mutations. Meta-analysis of dose-response relationships across studies can identify model-specific confounding factors .
Q. What strategies improve metabolic stability and tissue distribution in preclinical studies?
- Methodological Answer : Radiolabeled analogs (e.g., ³H or ¹⁴C) enable tracking via liquid chromatography-tandem mass spectrometry (LC-MS/MS) in plasma and tissues. Positron emission tomography (PET) with ¹⁸F-labeled derivatives visualizes real-time biodistribution. To enhance stability, prodrug modifications (e.g., phosphoramidates) or co-administration with metabolic inhibitors (e.g., CYP450 blockers) are tested .
Q. How can synthesis yield and purity be optimized for large-scale production?
- Methodological Answer : Implement process analytical technology (PAT) for real-time monitoring of glycosylation reactions. Membrane-based separation (e.g., nanofiltration) removes by-products, while crystallization optimization (e.g., anti-solvent addition) improves polymorphic purity. Statistical tools like response surface methodology (RSM) identify critical process parameters (temperature, solvent ratios) .
Q. What experimental frameworks address the compound’s limited in vivo pharmacokinetic data?
- Methodological Answer : Use physiologically based pharmacokinetic (PBPK) modeling to predict absorption, distribution, metabolism, and excretion (ADME). Validate models with in vivo sampling via microdialysis in target tissues (e.g., liver, lungs). Comparative studies with structural analogs (e.g., 1-(5-O-Benzoyl-β-D-ribofuranosyl)-derivatives) elucidate metabolic bottlenecks .
Q. Methodological Considerations Table
Properties
IUPAC Name |
2-[(2R,3R,4S,5R)-3,4-dihydroxy-5-(hydroxymethyl)oxolan-2-yl]-1,2,4-triazole-3-carboxamide | |
---|---|---|
Source | PubChem | |
URL | https://pubchem.ncbi.nlm.nih.gov | |
Description | Data deposited in or computed by PubChem | |
InChI |
InChI=1S/C8H12N4O5/c9-6(16)7-10-2-11-12(7)8-5(15)4(14)3(1-13)17-8/h2-5,8,13-15H,1H2,(H2,9,16)/t3-,4-,5-,8-/m1/s1 | |
Source | PubChem | |
URL | https://pubchem.ncbi.nlm.nih.gov | |
Description | Data deposited in or computed by PubChem | |
InChI Key |
HAMRZKKGAOJPDG-AFCXAGJDSA-N | |
Source | PubChem | |
URL | https://pubchem.ncbi.nlm.nih.gov | |
Description | Data deposited in or computed by PubChem | |
Canonical SMILES |
C1=NN(C(=N1)C(=O)N)C2C(C(C(O2)CO)O)O | |
Source | PubChem | |
URL | https://pubchem.ncbi.nlm.nih.gov | |
Description | Data deposited in or computed by PubChem | |
Isomeric SMILES |
C1=NN(C(=N1)C(=O)N)[C@H]2[C@@H]([C@@H]([C@H](O2)CO)O)O | |
Source | PubChem | |
URL | https://pubchem.ncbi.nlm.nih.gov | |
Description | Data deposited in or computed by PubChem | |
Molecular Formula |
C8H12N4O5 | |
Source | PubChem | |
URL | https://pubchem.ncbi.nlm.nih.gov | |
Description | Data deposited in or computed by PubChem | |
Molecular Weight |
244.20 g/mol | |
Source | PubChem | |
URL | https://pubchem.ncbi.nlm.nih.gov | |
Description | Data deposited in or computed by PubChem | |
CAS No. |
39030-43-8 | |
Record name | 1-beta-D-Ribofuranosyl-1H-1,2,4-triazole-5-carboxamide | |
Source | ChemIDplus | |
URL | https://pubchem.ncbi.nlm.nih.gov/substance/?source=chemidplus&sourceid=0039030438 | |
Description | ChemIDplus is a free, web search system that provides access to the structure and nomenclature authority files used for the identification of chemical substances cited in National Library of Medicine (NLM) databases, including the TOXNET system. | |
Record name | 1-.BETA.-D-RIBOFURANOSYL-1H-1,2,4-TRIAZOLE-5-CARBOXAMIDE | |
Source | FDA Global Substance Registration System (GSRS) | |
URL | https://gsrs.ncats.nih.gov/ginas/app/beta/substances/M521CC48D7 | |
Description | The FDA Global Substance Registration System (GSRS) enables the efficient and accurate exchange of information on what substances are in regulated products. Instead of relying on names, which vary across regulatory domains, countries, and regions, the GSRS knowledge base makes it possible for substances to be defined by standardized, scientific descriptions. | |
Explanation | Unless otherwise noted, the contents of the FDA website (www.fda.gov), both text and graphics, are not copyrighted. They are in the public domain and may be republished, reprinted and otherwise used freely by anyone without the need to obtain permission from FDA. Credit to the U.S. Food and Drug Administration as the source is appreciated but not required. | |
Retrosynthesis Analysis
AI-Powered Synthesis Planning: Our tool employs the Template_relevance Pistachio, Template_relevance Bkms_metabolic, Template_relevance Pistachio_ringbreaker, Template_relevance Reaxys, Template_relevance Reaxys_biocatalysis model, leveraging a vast database of chemical reactions to predict feasible synthetic routes.
One-Step Synthesis Focus: Specifically designed for one-step synthesis, it provides concise and direct routes for your target compounds, streamlining the synthesis process.
Accurate Predictions: Utilizing the extensive PISTACHIO, BKMS_METABOLIC, PISTACHIO_RINGBREAKER, REAXYS, REAXYS_BIOCATALYSIS database, our tool offers high-accuracy predictions, reflecting the latest in chemical research and data.
Strategy Settings
Precursor scoring | Relevance Heuristic |
---|---|
Min. plausibility | 0.01 |
Model | Template_relevance |
Template Set | Pistachio/Bkms_metabolic/Pistachio_ringbreaker/Reaxys/Reaxys_biocatalysis |
Top-N result to add to graph | 6 |
Feasible Synthetic Routes
Disclaimer and Information on In-Vitro Research Products
Please be aware that all articles and product information presented on BenchChem are intended solely for informational purposes. The products available for purchase on BenchChem are specifically designed for in-vitro studies, which are conducted outside of living organisms. In-vitro studies, derived from the Latin term "in glass," involve experiments performed in controlled laboratory settings using cells or tissues. It is important to note that these products are not categorized as medicines or drugs, and they have not received approval from the FDA for the prevention, treatment, or cure of any medical condition, ailment, or disease. We must emphasize that any form of bodily introduction of these products into humans or animals is strictly prohibited by law. It is essential to adhere to these guidelines to ensure compliance with legal and ethical standards in research and experimentation.