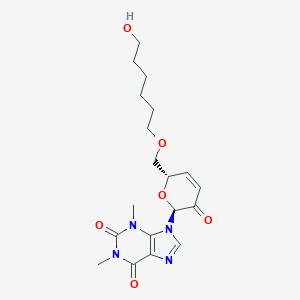
Dhp-TH
- Click on QUICK INQUIRY to receive a quote from our team of experts.
- With the quality product at a COMPETITIVE price, you can focus more on your research.
Overview
Description
"Dhp-TH" (Trihexadecylphosphane) is a phosphane-based compound characterized by three hexadecyl alkyl chains attached to a central phosphorus atom. This structural configuration imparts unique physicochemical properties, including high lipophilicity and thermal stability, which contribute to its biological activity .
Preparation Methods
Traditional Alkoxide-Mediated Condensation of Malonic Esters
The foundational method for synthesizing 4,6-dihydroxypyrimidine (DHP) involves the reaction of malonic esters with formamide in the presence of alkali metal alkoxides. This one-step condensation, first patented by Lonza AG , operates under autogenous pressure and elevated temperatures (102–120°C) to achieve yields exceeding 85%. Key improvements to this process include the use of concentrated sodium methoxide solutions (e.g., NM35, 35% in methanol) and precise temperature control during the holding phase. For instance, maintaining the reaction mixture at 105°C for 30 minutes post-addition of dimethyl malonate and formamide increases the yield to 86.5%, compared to 81.5% at 90°C for 60 minutes .
A critical limitation of earlier methods was inefficient nitrogen utilization due to multistep amidation processes. The integration of alkali metal alkoxides directly into the reaction mixture resolved this by enabling simultaneous deprotonation and cyclization . The resulting DHP is precipitated by acidification, filtered, and dried under vacuum (20–30 mbar at 70–80°C), yielding a product with ≥96% purity and <5 ppm cyanide residues .
Hantzsch Dihydropyridine Synthesis Using Nanocatalysts
The Hantzsch reaction remains a cornerstone for synthesizing 1,4-dihydropyridine (1,4-DHP) derivatives, which are structurally related to DHP. Recent innovations employ nanocatalysts to enhance reaction efficiency and sustainability. Magnetic nanoparticles (e.g., Fe₃O₄), metal oxides (e.g., ZnO), and nanocomposites (e.g., SiO₂-TiO₂) have demonstrated exceptional catalytic activity in multicomponent reactions involving aldehydes, β-ketoesters, and ammonium acetate .
For example, a solvent-free protocol using 10 wt% ZnO nanoparticles at 80°C achieves 92% yield of ethyl 2,6-dimethyl-4-phenyl-1,4-dihydropyridine-3,5-dicarboxylate within 20 minutes . This method eliminates volatile organic solvents and simplifies purification, as the catalyst is recoverable via magnetic separation or filtration. Comparative studies show nanocatalysts reduce reaction times by 40–60% compared to conventional acid-catalyzed methods .
Mechanistic Insights into Nanocatalyzed Hantzsch Reactions
The catalytic activity of nanomaterials in Hantzsch reactions arises from their high surface area and Lewis acid sites, which facilitate imine formation and cyclocondensation. Infrared spectroscopy (IR) and X-ray diffraction (XRD) analyses confirm that ZnO nanoparticles stabilize the enolic form of β-ketoesters, accelerating keto-enol tautomerism and subsequent nucleophilic attack by ammonium ions . This mechanistic pathway minimizes side reactions, such as the formation of 2,4,6-trihydroxynicotinamide, which is a common impurity in traditional DHP syntheses .
Continuous-Flow Synthesis in Microwave Reactors
Continuous-flow systems represent a paradigm shift in DHP production, offering scalability and precise control over reaction parameters. The Bohlmann–Rahtz pyridine synthesis and Hantzsch dihydropyridine synthesis have been successfully adapted to microwave flow reactors, enabling rapid heating (120–150°C) and reduced residence times (≤10 minutes) .
In one configuration, a mixture of ethyl acetoacetate, benzaldehyde, and ammonium acetate is pumped through a silica-packed column at 2 mL/min under microwave irradiation (300 W). This setup achieves 89% conversion to 1,4-DHP derivatives with >95% regioselectivity, compared to 72% yield in batch reactors . The elimination of manual intervention and improved heat transfer in flow systems reduce energy consumption by 30–40% .
Solvent-Free and Green Chemistry Approaches
Environmental concerns have driven the adoption of solvent-free methodologies for DHP synthesis. A notable example involves grinding stoichiometric amounts of malonic ester, formamide, and sodium methoxide in a ball mill at ambient temperature. This mechanochemical approach achieves 78% yield within 2 hours, albeit with slightly lower purity (93%) than thermal methods .
Alternatively, ionic liquids such as 1-butyl-3-methylimidazolium tetrafluoroborate ([BMIM][BF₄]) serve as dual solvents and catalysts, enabling DHP synthesis at 100°C with 88% yield and 99% atom economy . These methods align with green chemistry principles by minimizing waste and avoiding toxic solvents.
Structural and Purity Considerations in DHP Synthesis
The quality of DHP derivatives is critically dependent on reaction conditions. High-performance liquid chromatography (HPLC) analyses reveal that temperatures above 100°C suppress the formation of 2,4,6-trihydroxynicotinamide, a byproduct that compromises pharmacological activity . Furthermore, nuclear magnetic resonance (NMR) studies demonstrate that acidic conditions (pH 4.0) during workup enhance β-O-4 linkage formation in dehydrogenation polymers (DHPs), which are used as lignin models .
Comparative Analysis of DHP Synthesis Methods
Chemical Reactions Analysis
Types of Reactions
BW723C86 undergoes various chemical reactions, including:
Oxidation: The compound can be oxidized under specific conditions to form oxidized derivatives.
Reduction: Reduction reactions can be performed to modify the functional groups present in the compound.
Substitution: Substitution reactions, such as nucleophilic substitution, can be used to introduce different substituents into the molecule.
Common Reagents and Conditions
Common reagents used in these reactions include oxidizing agents like potassium permanganate, reducing agents like lithium aluminum hydride, and nucleophiles for substitution reactions. The reactions are typically carried out under controlled conditions to ensure the desired product is obtained .
Major Products Formed
The major products formed from these reactions depend on the specific reaction conditions and reagents used. For example, oxidation of BW723C86 can lead to the formation of oxidized derivatives with altered pharmacological properties .
Scientific Research Applications
BW723C86 has a wide range of scientific research applications:
Mechanism of Action
BW723C86 exerts its effects by selectively activating the 5-HT2B receptor. This activation leads to a cascade of intracellular signaling events, including the activation of the aryl hydrocarbon receptor (AhR) and the inhibition of protein kinase A (PKA) and cAMP response element-binding protein (CREB) pathways . These molecular targets and pathways are involved in various physiological processes, including inflammation, cell proliferation, and melanin synthesis .
Comparison with Similar Compounds
Dhp-TH belongs to the organophosphane family, which includes structurally analogous compounds such as triphenylphosphane (PPh₃) and tris(2-ethylhexyl)phosphane (TEHP). Below is a systematic comparison based on biological activity, structural features, and regulatory considerations:
Structural and Functional Differences
Compound | Substituent Groups | Lipophilicity (LogP) | Key Applications |
---|---|---|---|
This compound | Three C₁₆ alkyl chains | ~12.5 (estimated) | Anticancer, antimicrobial |
Triphenylphosphane | Three phenyl rings | ~4.2 | Catalysis, material science |
Tris(2-ethylhexyl)phosphane | Three branched C₈ alkyl chains | ~9.8 | Solvent stabilization |
The long alkyl chains in this compound enhance membrane permeability, enabling potent interactions with cellular targets, whereas the aromatic rings in triphenylphosphane favor π-π stacking in catalytic systems . TEHP’s branched alkyl chains reduce crystallinity, making it suitable for industrial solvents but less bioactive .
Toxicological and Regulatory Considerations
For example, bridging studies may leverage existing data on genotoxicity (Ames test negative) and acute toxicity (LD₅₀ >2000 mg/kg in rodents) to support newer iterations . In contrast, triphenylphosphane and TEHP lack such frameworks, requiring full toxicological assessments for novel applications .
Q & A
Basic Research Questions
Q. How can I formulate a focused research question on Dhp-TH's biochemical mechanisms?
- Methodology : Use the P-E/I-C-O framework (Population, Exposure/Intervention, Comparison, Outcome) to structure your question. For example:
- Population: Specific cell lines or in vivo models used in this compound studies.
- Intervention: this compound dosage, administration route, or molecular interactions.
- Comparison: Control groups (e.g., untreated cells or alternative compounds).
- Outcome: Measurable endpoints (e.g., enzyme inhibition, gene expression changes).
Q. What experimental design principles apply to this compound studies?
- Key Steps :
Define independent/dependent variables (e.g., this compound concentration vs. metabolic activity).
Use randomization and blinding to reduce bias in in vivo/in vitro assays .
Include positive/negative controls (e.g., known inhibitors or vehicle solutions).
Adhere to ethical guidelines for lab protocols (e.g., animal welfare, biosafety levels) .
Q. How should I design a data management plan (DMP) for this compound research?
- Elements to Include :
- Data Types : Raw spectroscopy data, chromatograms, or genomic datasets.
- Storage Formats : Non-proprietary formats (e.g., .csv, .fasta) for interoperability .
- Metadata Standards : Use FAIR principles (Findable, Accessible, Interoperable, Reusable) .
- Long-Term Archiving : Specify repositories (e.g., Zenodo, institutional databases) .
Advanced Research Questions
Q. How can I resolve contradictions in this compound's reported pharmacological effects?
- Methodology :
Conduct systematic literature reviews to identify conflicting results (e.g., this compound’s dual role as agonist/antagonist).
Perform dose-response replications under standardized conditions to isolate variables .
Apply triangulation (e.g., cross-validate findings via ELISA, qPCR, and molecular docking simulations) .
Q. What statistical approaches are optimal for analyzing this compound's multi-modal datasets?
- Advanced Methods :
- Mixed-effects models for longitudinal in vivo data with repeated measures.
- Principal Component Analysis (PCA) to reduce dimensionality in omics datasets.
- Bayesian inference for probabilistic interpretation of uncertain mechanistic pathways .
Q. How can I optimize this compound's experimental protocols for reproducibility?
- Strategies :
Pre-register protocols on platforms like Open Science Framework.
Use automated liquid handlers to minimize human error in high-throughput screens .
Document batch effects (e.g., reagent lot variations) and include them as covariates in analysis .
Q. What methodologies validate this compound's target specificity in complex biological systems?
- Approaches :
- CRISPR-Cas9 knockouts to confirm target gene necessity.
- Isothermal titration calorimetry (ITC) for binding affinity quantification.
- Network pharmacology to map this compound’s interactions within signaling pathways .
Q. Methodological Validation & Ethics
Q. How do I ensure this compound's cytotoxicity assays align with regulatory standards?
- Steps :
Follow OECD guidelines (e.g., Test No. 423 for acute oral toxicity).
Use ISO-certified cell lines and calibrate equipment (e.g., flow cytometers) routinely .
Report IC50/EC50 values with 95% confidence intervals .
Q. What strategies mitigate bias in this compound's clinical trial design?
- Recommendations :
- Stratified randomization to balance demographic covariates.
- Double-blind protocols for treatment allocation and outcome assessment.
- Pre-specified endpoints to avoid data dredging .
Q. Cross-Disciplinary Integration
Q. How can this compound research integrate computational and wet-lab findings?
- Framework :
Use molecular dynamics simulations to predict this compound’s binding conformations.
Validate in silico results with surface plasmon resonance (SPR) or X-ray crystallography .
Apply machine learning to identify structure-activity relationships (SAR) from historical data .
Properties
CAS No. |
107838-98-2 |
---|---|
Molecular Formula |
C19H26N4O6 |
Molecular Weight |
406.4 g/mol |
IUPAC Name |
9-[(2S,6S)-2-(6-hydroxyhexoxymethyl)-5-oxo-2H-pyran-6-yl]-1,3-dimethylpurine-2,6-dione |
InChI |
InChI=1S/C19H26N4O6/c1-21-16-15(17(26)22(2)19(21)27)20-12-23(16)18-14(25)8-7-13(29-18)11-28-10-6-4-3-5-9-24/h7-8,12-13,18,24H,3-6,9-11H2,1-2H3/t13-,18-/m0/s1 |
InChI Key |
FHOFJRKDXZHSEO-UGSOOPFHSA-N |
SMILES |
CN1C2=C(C(=O)N(C1=O)C)N=CN2C3C(=O)C=CC(O3)COCCCCCCO |
Isomeric SMILES |
CN1C2=C(C(=O)N(C1=O)C)N=CN2[C@@H]3C(=O)C=C[C@H](O3)COCCCCCCO |
Canonical SMILES |
CN1C2=C(C(=O)N(C1=O)C)N=CN2C3C(=O)C=CC(O3)COCCCCCCO |
Synonyms |
7-(3,4-dideoxy-6-O-(6-hydroxyhexyl)hex-3-enopyranosyl-2-ulose)theophylline DHP-TH |
Origin of Product |
United States |
Disclaimer and Information on In-Vitro Research Products
Please be aware that all articles and product information presented on BenchChem are intended solely for informational purposes. The products available for purchase on BenchChem are specifically designed for in-vitro studies, which are conducted outside of living organisms. In-vitro studies, derived from the Latin term "in glass," involve experiments performed in controlled laboratory settings using cells or tissues. It is important to note that these products are not categorized as medicines or drugs, and they have not received approval from the FDA for the prevention, treatment, or cure of any medical condition, ailment, or disease. We must emphasize that any form of bodily introduction of these products into humans or animals is strictly prohibited by law. It is essential to adhere to these guidelines to ensure compliance with legal and ethical standards in research and experimentation.