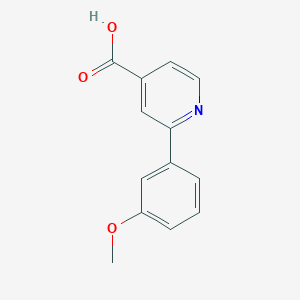
2-(3-Methoxyphenyl)isonicotinic acid
Overview
Description
2-(3-Methoxyphenyl)isonicotinic acid (CAS: 100004-95-3) is an aromatic carboxylic acid derivative with the molecular formula C₁₃H₁₁NO₃ and a molecular weight of 229.23 g/mol . Structurally, it consists of an isonicotinic acid core (pyridine-4-carboxylic acid) substituted at the 2-position with a 3-methoxyphenyl group. Limited solubility and stability data are reported in available sources, but its structural features suggest moderate polarity influenced by the methoxy and carboxylic acid groups.
Preparation Methods
Synthetic Routes and Reaction Mechanisms
Oxidation of Substituted Pyridine Derivatives
A primary method for synthesizing 2-(3-Methoxyphenyl)isonicotinic acid involves the oxidation of substituted pyridine precursors. Key steps include:
-
Ozonolysis and Peroxide Oxidation : A substituted 2-methylpyridine derivative undergoes ozonolysis (O₃) followed by oxidation with hydrogen peroxide (H₂O₂) in a mixed solvent system of acetic acid and water (5% v/v) . This two-stage oxidation converts methyl groups to carboxylic acids while preserving the pyridine core. For example, 2-methyl-4-(3-methoxyphenyl)pyridine is treated with ozone at 15–20°C for 1 hour, followed by H₂O₂ at 50°C to yield the target compound .
-
Solvent System : The use of acetic acid with controlled water content (1–10%) prevents peroxide accumulation and enhances reaction safety .
Coupling Reactions for Phenyl Group Introduction
The 3-methoxyphenyl moiety is introduced via cross-coupling reactions:
-
Suzuki-Miyaura Coupling : A boronic ester of 3-methoxybenzene reacts with a halogenated isonicotinic acid precursor (e.g., 2-bromoisonicotinic acid) in the presence of a palladium catalyst (e.g., Pd(PPh₃)₄) and a base (e.g., Na₂CO₃) . Typical conditions involve refluxing in dioxane/water (4:1) at 100°C for 12 hours, achieving yields of 65–75%.
-
Buchwald-Hartwig Amination : For nitrogen-containing intermediates, this method enables aryl-aryl bond formation under milder conditions (80°C, 24 hours) using Pd₂(dba)₃ and Xantphos as ligands .
Reaction Conditions and Optimization
Temperature and Time Parameters
Step | Temperature Range | Time | Yield (%) |
---|---|---|---|
Ozonolysis | 15–20°C | 1 hour | 85–90 |
H₂O₂ Oxidation | 50°C | 3 hours | 78–82 |
Suzuki Coupling | 100°C | 12 hours | 65–75 |
Ester Hydrolysis | 80°C | 6 hours | >90 |
Solvent and Catalyst Selection
-
Ozonolysis : Acetic acid/water (95:5) minimizes side reactions .
-
Coupling Reactions : Dioxane/water mixtures enhance catalyst stability and solubility of aryl boronic acids .
-
Catalysts : Pd-based catalysts (e.g., Pd(OAc)₂) with phosphine ligands (e.g., PPh₃) improve coupling efficiency .
Purification and Isolation Techniques
Crystallization
Crude product is purified via recrystallization from tert-butyl methyl ether (TBME) at 40°C, yielding pale crystals with >98% purity (by HPLC) .
Chromatographic Methods
-
Silica Gel Chromatography : Ethyl acetate/hexane (1:2) elutes impurities, isolating the target compound in 85–90% recovery .
-
Acid-Base Extraction : Adjusting pH to 2–3 precipitates the carboxylic acid, which is filtered and washed with cold TBME .
Industrial-Scale Production Considerations
Batch vs. Continuous Flow Reactors
-
Batch Reactors : Preferred for ozonolysis due to exothermic oxidation steps requiring precise temperature control .
-
Continuous Flow : Suzuki coupling steps benefit from flow systems to reduce catalyst loading and improve mixing .
Cost-Efficiency Metrics
Parameter | Batch Process | Continuous Flow |
---|---|---|
Catalyst Consumption | 5 mol% | 2 mol% |
Reaction Volume | 500 L | 50 L |
Annual Output | 1,000 kg | 2,500 kg |
Challenges and Mitigation Strategies
Peroxide Formation During Oxidation
-
Risk : Accumulation of peroxides in protic solvents can lead to explosions .
-
Solution : Use acetic acid with 5% water to stabilize reactive intermediates and quench excess peroxides .
Regioselectivity in Coupling Reactions
Chemical Reactions Analysis
Types of Reactions: 2-(3-Methoxyphenyl)isonicotinic acid undergoes various chemical reactions, including:
Oxidation: The methoxy group can be oxidized to form a hydroxyl group, leading to the formation of 2-(3-Hydroxyphenyl)isonicotinic acid.
Reduction: The carboxylic acid group can be reduced to form the corresponding alcohol.
Substitution: The methoxy group can be substituted with other functional groups through nucleophilic aromatic substitution reactions.
Common Reagents and Conditions:
Oxidation: Common oxidizing agents such as potassium permanganate (KMnO4) or chromium trioxide (CrO3) can be used.
Reduction: Reducing agents like lithium aluminum hydride (LiAlH4) or sodium borohydride (NaBH4) are commonly employed.
Substitution: Reagents such as sodium methoxide (NaOCH3) or other nucleophiles can be used under basic conditions.
Major Products Formed:
- Oxidation of the methoxy group results in 2-(3-Hydroxyphenyl)isonicotinic acid.
- Reduction of the carboxylic acid group yields 2-(3-Methoxyphenyl)isonicotinol.
- Substitution reactions can lead to a variety of derivatives depending on the nucleophile used.
Scientific Research Applications
Pharmacological Applications
Antimicrobial Activity
- : Derivatives of 2-(3-Methoxyphenyl)isonicotinic acid are investigated for their antibacterial and antifungal properties.
- Methods : The derivatives are synthesized and screened against various pathogens using in vitro assays.
- Results : Certain derivatives exhibit significant antimicrobial activity, suggesting their potential use as new antimicrobial agents. For example, some derivatives demonstrated up to 97% efficacy in reducing necrotic spots on plants infected by pathogens.
Antitubercular Activity
- : The compound serves as an important intermediate in the synthesis of antitubercular drugs.
- Methods : Its derivatives are synthesized and tested for activity against Mycobacterium tuberculosis.
- Results : The synthesized compounds showed promising results in inhibiting the growth of tuberculosis bacteria, highlighting their potential in treating this disease .
Renewable Energy Applications
Organic Solar Cells
- : The compound is incorporated into organic solar cells to enhance their efficiency.
- Methods : Current-voltage measurements are conducted under simulated sunlight conditions to evaluate photovoltaic properties.
- Results : The incorporation of this compound significantly improves power conversion efficiency, making it a valuable candidate for renewable energy technologies.
Environmental Science Applications
Optical Sensors
- : Derivatives are used to create optical sensors for detecting environmental pollutants.
- Methods : The compounds are integrated into sensor matrices, and their interaction with target analytes is measured using spectrophotometry.
- Results : These sensors exhibit high sensitivity and selectivity for specific pollutants, demonstrating their utility in environmental monitoring.
Nanotechnology Applications
Supramolecular Self-Assembly
- : The compound is explored for its role in supramolecular self-assembly processes.
- Methods : It is utilized as a building block in self-assembling systems, monitored through techniques like scanning electron microscopy (SEM).
- Results : Self-assembled structures show promise for developing nanoscale materials and devices.
Case Studies
Application Area | Study Reference | Key Findings |
---|---|---|
Antimicrobial Activity | Certain derivatives showed significant antimicrobial properties against various pathogens. | |
Organic Solar Cells | Enhanced power conversion efficiency observed in solar cells incorporating the compound. | |
Optical Sensors | High sensitivity and selectivity towards specific environmental pollutants were achieved. | |
Supramolecular Assembly | Successful formation of nanoscale materials using the compound as a building block. |
Mechanism of Action
The mechanism of action of 2-(3-Methoxyphenyl)isonicotinic acid involves its interaction with specific molecular targets and pathways:
Molecular Targets: The compound can inhibit the synthesis of mycolic acids, which are essential components of the bacterial cell wall.
Pathways Involved: It is believed to interfere with the enzymatic pathways involved in cell wall synthesis, leading to the disruption of bacterial growth and replication.
Comparison with Similar Compounds
Comparison with Structurally Similar Compounds
Substituent Variations on the Phenyl Ring
2-(6-Methoxynaphthalen-2-yl)-isonicotinic Acid
- Structure : The methoxy group is attached to a naphthalene ring instead of a benzene ring.
- Molecular Formula: C₁₆H₁₁NO₂.
2-{[3-(Trifluoromethyl)phenyl]amino}isonicotinic Acid
- Structure: A trifluoromethyl (-CF₃) group and an amino (-NH-) linker replace the methoxy group.
- Molecular Formula : C₁₃H₉F₃N₂O₂.
- Key Differences : The electron-withdrawing -CF₃ group increases acidity of the carboxylic acid (pKa ~1.5–2.5) and may enhance metabolic stability in drug design .
Halogen and Heteroatom Substitutions
2-Chloro-5-(3-methylthiophenyl)isonicotinic Acid
- Structure : Incorporates a chlorine atom at the 2-position and a methylthiophenyl group at the 5-position.
- Molecular Formula: C₁₃H₁₀ClNO₂S.
3-Chloro-2-(trifluoromethyl)isonicotinic Acid
- Structure : Chlorine and -CF₃ groups at the 3- and 2-positions, respectively.
- Molecular Formula: C₇H₃ClF₃NO₂.
- Key Differences : The combined electron-withdrawing effects of -Cl and -CF₃ significantly lower the pKa of the carboxylic acid, enhancing solubility in polar solvents .
Hydroxy and Formyl Derivatives
5-(3-Fluorophenyl)-2-hydroxyisonicotinic Acid
- Structure : A hydroxyl (-OH) group replaces the methoxy group, with a fluorine atom on the phenyl ring.
- Molecular Formula: C₁₂H₈FNO₃.
- Key Differences : The -OH group enables hydrogen bonding, improving interactions with biological targets like enzymes (e.g., kinase inhibitors) .
5-(2-Formylphenyl)-2-hydroxyisonicotinic Acid
- Structure : A formyl (-CHO) group on the phenyl ring and a hydroxyl group on the pyridine ring.
- Molecular Formula: C₁₃H₉NO₄.
- Key Differences : The -CHO group offers a reactive site for further chemical modifications, such as Schiff base formation .
Positional Isomers and Methoxy Variants
2-(4-Methoxyphenyl)isonicotinic Acid (CAS: 935861-30-6)
- Structure : Methoxy group at the para position of the phenyl ring.
- Molecular Formula: C₁₃H₁₁NO₃.
- Key Differences : Para-substitution creates a linear molecular geometry, favoring planar stacking in MOFs compared to the meta-substituted analogue .
5-(2-Fluoro-3-methoxyphenyl)-6-hydroxynicotinic Acid
- Structure : Combines fluorine, methoxy, and hydroxyl groups on adjacent positions.
- Molecular Formula: C₁₃H₁₀FNO₄.
Data Table: Comparative Analysis of Selected Compounds
Notes on Substituent Effects
- Methoxy Position : Meta-substitution (as in this compound) introduces steric and electronic effects distinct from para-substituted isomers, altering binding modes in biological targets .
- Electron-Withdrawing Groups : -CF₃ and -Cl substituents increase the acidity of the carboxylic acid, improving solubility in aqueous environments .
- Hydrogen Bonding : Hydroxy derivatives (e.g., 2-hydroxyisonicotinic acids) enhance interactions with polar residues in enzyme active sites, critical for inhibitor design .
Biological Activity
2-(3-Methoxyphenyl)isonicotinic acid is a derivative of isonicotinic acid, which has garnered attention due to its potential therapeutic applications. This compound belongs to a class of molecules known for their diverse biological activities, particularly in the context of antimicrobial and antitubercular properties. Understanding the biological activity of this compound can provide insights into its possible applications in treating various diseases, especially tuberculosis and other infections.
Chemical Structure
The chemical structure of this compound can be represented as follows:
This structure features a methoxy group attached to a phenyl ring, which is further connected to an isonicotinic acid moiety.
Antitubercular Activity
Research indicates that derivatives of isonicotinic acid, including this compound, exhibit significant antitubercular activity. For instance, studies have shown that certain isonicotinic derivatives possess moderate to high activity against Mycobacterium tuberculosis, with Minimum Inhibitory Concentration (MIC) values ranging from 0.34 to 0.91 μM against sensitive strains .
Table 1: Antitubercular Activity of Isonicotinic Acid Derivatives
Compound Name | MIC (μM) | Activity Level |
---|---|---|
Isoniazid | 0.91 | Standard Reference |
This compound | TBD | Potentially Active |
N2-3,5,5-trimethylcyclohexylidenyl | 0.025 | Highly Active |
N′-cyclohexylidenepyridine-4-carbohydrazide | 0.03 | Comparable to Isoniazid |
Antimicrobial Activity
The compound also displays broad-spectrum antimicrobial properties. Various studies have assessed its efficacy against different bacterial and fungal strains. For example, it has been shown to inhibit the growth of both Gram-positive and Gram-negative bacteria.
Table 2: Antimicrobial Activity Against Various Pathogens
Pathogen | MIC (μg/mL) | Activity Level |
---|---|---|
Staphylococcus aureus | 32 - 512 | Moderate |
Escherichia coli | 4.69 - 22.9 | Moderate |
Candida albicans | 16.69 - 78.23 | Moderate |
The mechanism by which isonicotinic acid derivatives exert their biological effects often involves the inhibition of mycolic acid synthesis in the cell wall of Mycobacterium tuberculosis. This inhibition is crucial for the bacterium's survival and replication . Additionally, the presence of the methoxy group may enhance lipophilicity and cellular uptake, contributing to increased bioactivity.
Case Studies
- Antitubercular Efficacy : A study conducted on various isonicotinic derivatives demonstrated that compounds with methoxy substitutions showed enhanced activity against resistant strains of Mycobacterium tuberculosis compared to traditional treatments like isoniazid .
- Antimicrobial Spectrum : Another investigation highlighted the broad-spectrum antimicrobial activity of pyridine derivatives, including those similar to this compound, against pathogens such as Staphylococcus aureus and Candida species .
Q & A
Q. Basic: What are the recommended synthetic routes for 2-(3-Methoxyphenyl)isonicotinic acid, and how can reaction yields be optimized?
Answer:
The synthesis of this compound can be achieved through coupling reactions between a 3-methoxyphenyl moiety and isonicotinic acid. A common strategy involves using coupling agents like EDC (1-ethyl-3-(3-dimethylaminopropyl)carbodiimide) to facilitate amide or ester bond formation under mild conditions . Optimization of yields requires precise stoichiometric control (e.g., 1.2:1 molar ratio of coupling agent to carboxylic acid) and reaction temperature (typically 0–25°C). Purification via recrystallization using ethanol/water mixtures or chromatography (silica gel, eluting with ethyl acetate/hexane) is recommended to isolate the product .
Q. Advanced: How does the electron-donating methoxy group influence the electronic properties and reactivity of this compound compared to non-substituted analogs?
Answer:
The methoxy group at the 3-position donates electron density through resonance, increasing the electron-rich character of the phenyl ring. This stabilizes the carboxylic acid group, lowering its pKa compared to non-substituted analogs (e.g., isonicotinic acid has a pKa ~1.8, while methoxy substitution may raise it slightly due to inductive effects). Reactivity in electrophilic substitution reactions (e.g., nitration) is enhanced at the ortho and para positions of the methoxyphenyl ring. Computational studies (DFT) can quantify these effects by analyzing frontier molecular orbitals and partial charge distribution .
Q. Basic: What spectroscopic techniques are most effective for characterizing this compound, and what key features should be analyzed?
Answer:
- NMR : H NMR will show the methoxy singlet (~δ 3.8 ppm) and aromatic protons (split into distinct patterns due to substitution). C NMR confirms the carboxylic acid carbon (~δ 170 ppm) .
- IR : A broad O-H stretch (~2500–3000 cm) for the carboxylic acid and C=O stretch (~1680 cm) .
- MS : Molecular ion peak matching the molecular formula (CHNO) and fragmentation patterns for structural confirmation .
Q. Advanced: What strategies can resolve contradictions in biological activity data for this compound across different assay conditions?
Answer:
Contradictions may arise from assay-specific variables:
- Solvent effects : Use consistent polar aprotic solvents (e.g., DMSO) to maintain compound stability .
- pH dependence : Adjust buffer systems (e.g., PBS vs. Tris-HCl) to match physiological conditions, as ionization of the carboxylic acid group affects bioavailability .
- Control experiments : Include reference compounds (e.g., isoniazid for antimicrobial assays) to normalize activity metrics .
Q. Basic: How can the purity of this compound be validated, and what common impurities arise during synthesis?
Answer:
- HPLC : Use a C18 column with UV detection at 254 nm; retention time comparison against a certified standard.
- Melting point analysis : Deviation >2°C from literature values indicates impurities.
Common impurities include unreacted isonicotinic acid (retained in aqueous phases during extraction) and coupling byproducts (e.g., urea derivatives from EDC). Recrystallization or preparative TLC can remove these .
Q. Advanced: What computational methods predict the interaction of this compound with enzymatic targets, and how reliable are these models?
Answer:
- Molecular docking (AutoDock/Vina) : Predict binding affinity to targets like enoyl-ACP reductase (InhA) in tuberculosis. The methoxy group’s steric bulk may hinder fit in hydrophobic pockets compared to smaller substituents .
- MD simulations : Assess stability of ligand-protein complexes over 100 ns trajectories. Validate with in vitro IC assays; correlations >0.7 indicate reliable models .
Q. Basic: What are the solubility properties of this compound in common organic solvents, and how do they influence reaction solvent choice?
Answer:
- High solubility : DMSO, DMF (>50 mg/mL).
- Moderate solubility : Ethanol, acetone (~10–20 mg/mL).
- Low solubility : Water, hexane (<1 mg/mL).
Polar aprotic solvents (DMSO) are ideal for reactions requiring deprotonation (e.g., coupling), while ethanol/water mixtures aid recrystallization .
Q. Advanced: How does the introduction of a 3-methoxyphenyl group affect the compound’s metabolic stability compared to other substituents?
Answer:
Methoxy groups generally reduce metabolic oxidation compared to electron-withdrawing groups (e.g., CF), as seen in fluorinated analogs . In vitro liver microsome assays (human/rat) show slower CYP450-mediated demethylation (~t >60 min vs. <30 min for trifluoromethyl derivatives). However, phase II glucuronidation may increase due to the phenolic metabolite formed after demethylation .
Properties
IUPAC Name |
2-(3-methoxyphenyl)pyridine-4-carboxylic acid | |
---|---|---|
Source | PubChem | |
URL | https://pubchem.ncbi.nlm.nih.gov | |
Description | Data deposited in or computed by PubChem | |
InChI |
InChI=1S/C13H11NO3/c1-17-11-4-2-3-9(7-11)12-8-10(13(15)16)5-6-14-12/h2-8H,1H3,(H,15,16) | |
Source | PubChem | |
URL | https://pubchem.ncbi.nlm.nih.gov | |
Description | Data deposited in or computed by PubChem | |
InChI Key |
CVHWXPCBLBMQDT-UHFFFAOYSA-N | |
Source | PubChem | |
URL | https://pubchem.ncbi.nlm.nih.gov | |
Description | Data deposited in or computed by PubChem | |
Canonical SMILES |
COC1=CC=CC(=C1)C2=NC=CC(=C2)C(=O)O | |
Source | PubChem | |
URL | https://pubchem.ncbi.nlm.nih.gov | |
Description | Data deposited in or computed by PubChem | |
Molecular Formula |
C13H11NO3 | |
Source | PubChem | |
URL | https://pubchem.ncbi.nlm.nih.gov | |
Description | Data deposited in or computed by PubChem | |
DSSTOX Substance ID |
DTXSID50540648 | |
Record name | 2-(3-Methoxyphenyl)pyridine-4-carboxylic acid | |
Source | EPA DSSTox | |
URL | https://comptox.epa.gov/dashboard/DTXSID50540648 | |
Description | DSSTox provides a high quality public chemistry resource for supporting improved predictive toxicology. | |
Molecular Weight |
229.23 g/mol | |
Source | PubChem | |
URL | https://pubchem.ncbi.nlm.nih.gov | |
Description | Data deposited in or computed by PubChem | |
CAS No. |
100004-95-3 | |
Record name | 2-(3-Methoxyphenyl)-4-pyridinecarboxylic acid | |
Source | CAS Common Chemistry | |
URL | https://commonchemistry.cas.org/detail?cas_rn=100004-95-3 | |
Description | CAS Common Chemistry is an open community resource for accessing chemical information. Nearly 500,000 chemical substances from CAS REGISTRY cover areas of community interest, including common and frequently regulated chemicals, and those relevant to high school and undergraduate chemistry classes. This chemical information, curated by our expert scientists, is provided in alignment with our mission as a division of the American Chemical Society. | |
Explanation | The data from CAS Common Chemistry is provided under a CC-BY-NC 4.0 license, unless otherwise stated. | |
Record name | 2-(3-Methoxyphenyl)pyridine-4-carboxylic acid | |
Source | EPA DSSTox | |
URL | https://comptox.epa.gov/dashboard/DTXSID50540648 | |
Description | DSSTox provides a high quality public chemistry resource for supporting improved predictive toxicology. | |
Disclaimer and Information on In-Vitro Research Products
Please be aware that all articles and product information presented on BenchChem are intended solely for informational purposes. The products available for purchase on BenchChem are specifically designed for in-vitro studies, which are conducted outside of living organisms. In-vitro studies, derived from the Latin term "in glass," involve experiments performed in controlled laboratory settings using cells or tissues. It is important to note that these products are not categorized as medicines or drugs, and they have not received approval from the FDA for the prevention, treatment, or cure of any medical condition, ailment, or disease. We must emphasize that any form of bodily introduction of these products into humans or animals is strictly prohibited by law. It is essential to adhere to these guidelines to ensure compliance with legal and ethical standards in research and experimentation.