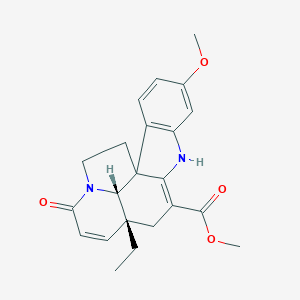
3-Oxo-11-methoxytabersonine
Overview
Description
3-Oxo-11-methoxytabersonine is a monoterpenoid indole alkaloid derived from plants in the Apocynaceae family, notably Alstonia yunnanensis and Melodinus suaveolens . Its molecular formula is C22H24N2O4, with a molecular weight of 380.45 g/mol . Structurally, it features a 3-ketone group and a methoxy substitution at position 11, distinguishing it from other tabersonine derivatives.
Preparation Methods
Biosynthetic Pathways and Enzymatic Oxidation
3-Oxo-11-methoxytabersonine is hypothesized to originate from tabersonine, a precursor in the vindoline biosynthesis pathway. Cytochrome P450 enzymes, particularly homologs of 16-methoxytabersonine 3-oxygenase (16T3O), catalyze regioselective oxidations and rearrangements. In the biosynthesis of related alkaloids like vincamine, 16T3O facilitates epoxidation at C15–C16, followed by epoxide opening and rearrangement to form the eburnamine-vincamine skeleton . By analogy, this compound may arise via enzymatic oxidation of tabersonine at C3, coupled with O-methylation at C11 (Scheme 1).
Mechanistic Insights :
-
Epoxidation : 16T3O introduces an epoxide at C15–C16 in tabersonine, which undergoes acid-catalyzed rearrangement to form iminium intermediates .
-
Oxo Group Formation : Subsequent oxidation of the C3 hydroxyl group by NADPH-dependent oxidases generates the 3-oxo moiety.
-
Methoxylation : S-Adenosyl methionine (SAM)-dependent methyltransferases mediate O-methylation at C11, though the specific enzyme remains uncharacterized .
Chemical Synthesis Strategies
Total Synthesis from Indole Precursors
The Wenkert enamine strategy, originally developed for vincamine, provides a template for constructing the pentacyclic framework of this compound. Key steps include:
-
Enamine Alkylation : Reaction of a substituted indole with methyl bromopyruvate forms the C3 keto group .
-
Bischler-Napieralski Cyclization : Builds the D-ring via intramolecular cyclization under acidic conditions .
-
Methoxylation : Late-stage O-methylation using methyl iodide and potassium carbonate in DMF introduces the 11-methoxy group .
Example Protocol :
-
Step 1 : Grignard addition to aldehyde 24 with phenylmagnesium bromide yields alcohol 25 .
-
Step 2 : TBDMS protection of 25 , followed by Me₂AlCl-catalyzed coupling with acid chloride 14 , affords intermediate 27 .
-
Step 3 : Oxidation with MnO₂ introduces the 3-oxo group, and SAM-mediated methylation finalizes the 11-methoxy substituent .
Semisynthesis from Tabersonine
Direct modification of tabersonine avoids de novo ring construction:
-
Protection : Boc protection of the indole nitrogen prevents unwanted side reactions.
-
Oxidation : Jones reagent (CrO₃/H₂SO₄) selectively oxidizes C3–OH to 3-oxo .
-
Methylation : Mitsunobu reaction with methanol and DIAD/PPh₃ installs the 11-methoxy group .
Challenges :
-
Regioselectivity : Competing oxidation at C14 or over-oxidation to carboxylic acids necessitates careful reagent selection .
-
Intermediate Stability : Hemiaminal intermediates, as seen in metabolite syntheses, are prone to decomposition, requiring low-temperature processing .
Comparative Analysis of Synthetic Routes
Analytical Characterization
-
NMR Spectroscopy :
-
High-Resolution Mass Spectrometry (HR-MS) :
Chemical Reactions Analysis
Types of Reactions: GSK Compound 34 can undergo various chemical reactions, including:
Oxidation: The hydroxyl groups on the phenyl ring can be oxidized to form quinones.
Reduction: The carbonyl groups in the pyrrole-2,5-dione core can be reduced to form alcohols.
Substitution: The fluorine atom on the indole ring can be substituted with other nucleophiles under appropriate conditions.
Common Reagents and Conditions:
Oxidation: Reagents such as potassium permanganate or chromium trioxide in acidic conditions.
Reduction: Reagents like sodium borohydride or lithium aluminum hydride.
Substitution: Nucleophiles such as amines or thiols in the presence of a base.
Major Products:
Oxidation: Quinone derivatives.
Reduction: Alcohol derivatives.
Substitution: Various substituted indole derivatives.
Scientific Research Applications
GSK Compound 34 has a wide range of applications in scientific research:
Chemistry: Used as a probe to study reaction mechanisms and enzyme kinetics.
Biology: Employed in cell-based assays to investigate cellular pathways and protein interactions.
Medicine: Explored as a potential therapeutic agent for diseases such as cancer and neurodegenerative disorders.
Industry: Utilized in the development of new drugs and as a standard in analytical chemistry
Mechanism of Action
GSK Compound 34 exerts its effects by binding to the active site of its target protein, thereby inhibiting its normal function. This inhibition disrupts key biochemical reactions within the cell, leading to a cascade of downstream effects. The compound’s molecular targets include enzymes involved in signal transduction and metabolic pathways .
Comparison with Similar Compounds
The bioactivity of 3-Oxo-11-methoxytabersonine is influenced by its structural modifications compared to related alkaloids. Below is a detailed comparison:
Table 1: Structural and Pharmacological Comparison of Tabersonine Derivatives
Key Findings from Comparative Studies
The 11-methoxy substitution increases lipophilicity, which may improve membrane permeability compared to 11-hydroxytabersonine .
Anticancer Efficacy :
- This compound demonstrates dose-dependent apoptosis in CRC cells, with mechanisms possibly involving AMPK and PI3K/AKT/mTOR pathways, similar to the related compound chaetocochin J .
- In contrast, unmodified tabersonine shows weaker activity, highlighting the importance of substitutions for potency .
Natural Sources and Biosynthesis :
- This compound is isolated from Melodinus suaveolens stems alongside analogs like melosuavine A and venalstonine, suggesting shared biosynthetic pathways involving oxidation and methylation .
- Tabersonine serves as a precursor for many derivatives, with oxidation at C-3 and substitution at C-11 being critical steps .
Biological Activity
Introduction
3-Oxo-11-methoxytabersonine is a monoterpenoid indole alkaloid derived from the Melodinus genus, particularly noted for its potential therapeutic applications in oncology. This compound has garnered attention due to its biological activities, particularly its anticancer properties against various cell lines. This article aims to provide a comprehensive overview of the biological activity of this compound, including its mechanisms of action, relevant case studies, and detailed research findings.
Chemical Structure and Properties
This compound is characterized by its complex structure typical of indole alkaloids. Its chemical formula includes a methoxy group and a keto group, contributing to its pharmacological properties. The compound's structural features are critical for its interaction with biological targets.
Anticancer Properties
Research indicates that this compound exhibits significant cytotoxic effects against various cancer cell lines. Notably, studies have shown its effectiveness against colorectal cancer (CRC) and lung cancer cell lines.
1. Colorectal Cancer (CRC)
In vitro studies on HCT116 colorectal cancer cells revealed an IC50 value of approximately 25.3 μM , indicating moderate cytotoxicity. The compound appears to induce apoptosis and cell cycle arrest through various molecular pathways, including the downregulation of cyclin B1 and cdc2, leading to G2/M phase arrest .
2. Lung Cancer
The compound also demonstrated potent activity against human lung cancer cell lines such as H157 and A549. It was found to induce necroptosis in an apoptosis-independent manner, with significant induction of autophagy observed through the activation of the AMP-activated protein kinase (AMPK)/mTOR and JNK signaling pathways . The IC50 values for these cell lines were reported at 22.6 μM for H157 and a lower value for A549, showcasing its potential as a therapeutic agent in lung cancer treatment .
The mechanisms underlying the anticancer effects of this compound involve several key pathways:
- Apoptosis Induction : The compound promotes apoptosis via modulation of pro-apoptotic and anti-apoptotic proteins.
- Cell Cycle Arrest : It causes cell cycle arrest at the G2/M phase by downregulating cyclins and cdc2.
- Autophagy Activation : The activation of autophagy is protective against necroptosis induced by the compound, suggesting a dual role in cell survival and death .
Summary of Research Findings
Cell Line | IC50 (μM) | Mechanism | References |
---|---|---|---|
HCT116 | 25.3 | G2/M phase arrest | |
H157 | 22.6 | Necroptosis, autophagy activation | |
A549 | Lower than H157 | Autophagy induction |
Study 1: Efficacy Against Colorectal Cancer
A study published in Frontiers in Pharmacology explored the effects of this compound on HCT116 cells. The results showed significant inhibition of cell proliferation and induction of apoptosis, confirming its potential as a CRC therapeutic agent .
Study 2: Mechanistic Insights in Lung Cancer
Another investigation focused on the H157 and A549 lung cancer models demonstrated that treatment with this compound led to increased reactive oxygen species (ROS) production and subsequent activation of autophagic pathways, highlighting its multifaceted role in cancer therapy .
Q & A
Basic Research Questions
Q. What methodologies are employed to isolate 3-Oxo-11-methoxytabersonine from natural sources?
Isolation typically involves solvent extraction (e.g., ethanol or methanol) followed by chromatographic techniques such as column chromatography or HPLC. The compound is sourced primarily from Alstonia yunnanensis, where plant material is dried, powdered, and subjected to sequential solvent partitioning. Final purification may require reverse-phase HPLC with UV detection at 254 nm. Structural confirmation is achieved via spectroscopic methods (NMR, MS) and comparison with literature data .
Q. How is the structural identity of this compound validated?
A combination of H-NMR, C-NMR, and high-resolution mass spectrometry (HRMS) is used to confirm the molecular structure. X-ray crystallography may be applied if single crystals are obtainable. For novel derivatives, elemental analysis and 2D NMR techniques (e.g., COSY, HSQC) are critical to resolve stereochemical ambiguities. Full experimental details, including solvent systems and instrument parameters, must be documented to ensure reproducibility .
Q. What are the primary natural sources of this compound, and how are they selected for study?
The compound is predominantly isolated from Alstonia yunnanensis (). Source selection relies on ethnobotanical data, phylogenetic proximity to known alkaloid-producing species, and preliminary phytochemical screening (e.g., TLC or LC-MS profiling). Researchers should prioritize voucher specimen deposition in herbariums for traceability .
Q. What in vitro assays are recommended for initial bioactivity screening?
Common assays include cytotoxicity testing (e.g., MTT assay on cancer cell lines), enzyme inhibition studies (e.g., acetylcholinesterase for neurological activity), and antimicrobial susceptibility tests. Positive controls (e.g., doxorubicin for cytotoxicity) and dose-response curves are essential. Data should be analyzed using IC or EC values with appropriate statistical validation (e.g., ANOVA) .
Advanced Research Questions
Q. What strategies address challenges in the total synthesis of this compound?
Retrosynthetic analysis often focuses on constructing the indole alkaloid core via Pictet-Spengler or Fischer indole synthesis. Key challenges include stereoselective introduction of the 11-methoxy group and stabilization of the 3-oxo moiety. Protecting groups (e.g., tert-butyldimethylsilyl for hydroxyls) and transition-metal catalysis (e.g., palladium-mediated cross-coupling) are frequently employed. Computational modeling (DFT) can optimize reaction pathways .
Q. How can contradictions in reported bioactivity data be resolved?
Contradictions may arise from variations in assay conditions (e.g., cell line specificity, serum concentration). To resolve these, researchers should:
- Replicate studies under standardized protocols (e.g., CLSI guidelines for antimicrobial assays).
- Validate compound purity (>95% by HPLC) and stability (e.g., LC-MS post-assay).
- Perform meta-analyses of published data to identify confounding variables .
Q. What experimental approaches elucidate the biosynthetic pathway of this compound?
Isotopic labeling (e.g., C-glucose feeding) combined with LC-MS/MS can track precursor incorporation. Gene knockout studies in host organisms (e.g., A. yunnanensis cell cultures) or heterologous expression in E. coli may identify key enzymes (e.g., cytochrome P450s for methoxylation). Transcriptomic and metabolomic datasets are integrated to map pathway nodes .
Q. How do structural modifications at the 3-oxo or 11-methoxy positions affect bioactivity?
Systematic SAR (Structure-Activity Relationship) studies involve synthesizing analogs (e.g., 3-hydroxy or 11-demethoxy derivatives) and testing them in parallel. Molecular docking (e.g., AutoDock Vina) predicts binding affinities to target proteins (e.g., tubulin for anticancer activity). Physicochemical properties (logP, polar surface area) are calculated to assess bioavailability .
Q. What methodologies validate the ecological role of this compound in its native plant species?
Field studies analyze compound distribution across plant tissues (e.g., leaves vs. roots) via LC-MS. Bioassays with herbivores or pathogens test its role in plant defense. Comparative metabolomics of stressed vs. unstressed plants can link compound production to environmental triggers .
Q. How can computational tools enhance the study of this compound?
Molecular dynamics simulations (e.g., GROMACS) model interactions with biological targets (e.g., DNA topoisomerases). QSAR (Quantitative Structure-Activity Relationship) models predict novel derivatives with enhanced activity. Machine learning (e.g., Random Forest classifiers) identifies structural features correlated with specific bioactivities .
Q. Methodological Notes
- Data Reproducibility : Document all experimental parameters (e.g., solvent ratios, temperature gradients) in Supplementary Information to enable replication .
- Ethical Compliance : Adhere to Nagoya Protocol guidelines when sourcing plant material .
- Open Science : Deposit raw spectral data in public repositories (e.g., Zenodo) with CC-BY licenses .
Properties
IUPAC Name |
methyl (12R,19S)-12-ethyl-5-methoxy-15-oxo-8,16-diazapentacyclo[10.6.1.01,9.02,7.016,19]nonadeca-2(7),3,5,9,13-pentaene-10-carboxylate | |
---|---|---|
Source | PubChem | |
URL | https://pubchem.ncbi.nlm.nih.gov | |
Description | Data deposited in or computed by PubChem | |
InChI |
InChI=1S/C22H24N2O4/c1-4-21-8-7-17(25)24-10-9-22(20(21)24)15-6-5-13(27-2)11-16(15)23-18(22)14(12-21)19(26)28-3/h5-8,11,20,23H,4,9-10,12H2,1-3H3/t20-,21-,22?/m0/s1 | |
Source | PubChem | |
URL | https://pubchem.ncbi.nlm.nih.gov | |
Description | Data deposited in or computed by PubChem | |
InChI Key |
ULVYAIAFWAUMLF-LGTSYYJHSA-N | |
Source | PubChem | |
URL | https://pubchem.ncbi.nlm.nih.gov | |
Description | Data deposited in or computed by PubChem | |
Canonical SMILES |
CCC12CC(=C3C4(C1N(CC4)C(=O)C=C2)C5=C(N3)C=C(C=C5)OC)C(=O)OC | |
Source | PubChem | |
URL | https://pubchem.ncbi.nlm.nih.gov | |
Description | Data deposited in or computed by PubChem | |
Isomeric SMILES |
CC[C@]12CC(=C3C4([C@H]1N(CC4)C(=O)C=C2)C5=C(N3)C=C(C=C5)OC)C(=O)OC | |
Source | PubChem | |
URL | https://pubchem.ncbi.nlm.nih.gov | |
Description | Data deposited in or computed by PubChem | |
Molecular Formula |
C22H24N2O4 | |
Source | PubChem | |
URL | https://pubchem.ncbi.nlm.nih.gov | |
Description | Data deposited in or computed by PubChem | |
DSSTOX Substance ID |
DTXSID00908017 | |
Record name | Methyl 16-methoxy-8-oxo-2,3,6,7-tetradehydroaspidospermidine-3-carboxylate | |
Source | EPA DSSTox | |
URL | https://comptox.epa.gov/dashboard/DTXSID00908017 | |
Description | DSSTox provides a high quality public chemistry resource for supporting improved predictive toxicology. | |
Molecular Weight |
380.4 g/mol | |
Source | PubChem | |
URL | https://pubchem.ncbi.nlm.nih.gov | |
Description | Data deposited in or computed by PubChem | |
CAS No. |
102719-84-6 | |
Record name | 3-Oxo-11-methoxytabersonine | |
Source | ChemIDplus | |
URL | https://pubchem.ncbi.nlm.nih.gov/substance/?source=chemidplus&sourceid=0102719846 | |
Description | ChemIDplus is a free, web search system that provides access to the structure and nomenclature authority files used for the identification of chemical substances cited in National Library of Medicine (NLM) databases, including the TOXNET system. | |
Record name | Methyl 16-methoxy-8-oxo-2,3,6,7-tetradehydroaspidospermidine-3-carboxylate | |
Source | EPA DSSTox | |
URL | https://comptox.epa.gov/dashboard/DTXSID00908017 | |
Description | DSSTox provides a high quality public chemistry resource for supporting improved predictive toxicology. | |
Retrosynthesis Analysis
AI-Powered Synthesis Planning: Our tool employs the Template_relevance Pistachio, Template_relevance Bkms_metabolic, Template_relevance Pistachio_ringbreaker, Template_relevance Reaxys, Template_relevance Reaxys_biocatalysis model, leveraging a vast database of chemical reactions to predict feasible synthetic routes.
One-Step Synthesis Focus: Specifically designed for one-step synthesis, it provides concise and direct routes for your target compounds, streamlining the synthesis process.
Accurate Predictions: Utilizing the extensive PISTACHIO, BKMS_METABOLIC, PISTACHIO_RINGBREAKER, REAXYS, REAXYS_BIOCATALYSIS database, our tool offers high-accuracy predictions, reflecting the latest in chemical research and data.
Strategy Settings
Precursor scoring | Relevance Heuristic |
---|---|
Min. plausibility | 0.01 |
Model | Template_relevance |
Template Set | Pistachio/Bkms_metabolic/Pistachio_ringbreaker/Reaxys/Reaxys_biocatalysis |
Top-N result to add to graph | 6 |
Feasible Synthetic Routes
Disclaimer and Information on In-Vitro Research Products
Please be aware that all articles and product information presented on BenchChem are intended solely for informational purposes. The products available for purchase on BenchChem are specifically designed for in-vitro studies, which are conducted outside of living organisms. In-vitro studies, derived from the Latin term "in glass," involve experiments performed in controlled laboratory settings using cells or tissues. It is important to note that these products are not categorized as medicines or drugs, and they have not received approval from the FDA for the prevention, treatment, or cure of any medical condition, ailment, or disease. We must emphasize that any form of bodily introduction of these products into humans or animals is strictly prohibited by law. It is essential to adhere to these guidelines to ensure compliance with legal and ethical standards in research and experimentation.