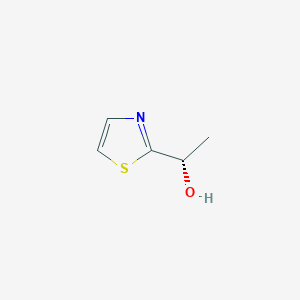
(1S)-1-(1,3-thiazol-2-yl)ethan-1-ol
Overview
Description
(1S)-1-(1,3-Thiazol-2-yl)ethan-1-ol is a chiral secondary alcohol featuring a thiazole ring, a heterocyclic moiety containing sulfur and nitrogen. Its molecular formula is C₅H₇NOS (MW: 129.18 g/mol), and it is commercially available with a CAS number 104863-49-2 . The compound’s stereospecific (1S) configuration is critical for its biological and chemical interactions, as chirality often dictates binding affinity and reactivity in pharmaceutical and agrochemical applications.
Preparation Methods
Hantzsch Thiazole Synthesis Approach
The Hantzsch reaction remains a cornerstone for constructing thiazole rings, leveraging thiourea derivatives and α-halo carbonyl compounds. For (1S)-1-(1,3-thiazol-2-yl)ethan-1-ol, this method involves cyclizing a thiourea precursor with a chiral α-halo alcohol.
Reaction Mechanism and Optimization
A modified Hantzsch protocol reacts 2-aminothiazole with (S)-2-chloropropanol in ethanol under reflux. The thiourea intermediate forms via nucleophilic substitution, followed by cyclization to yield the thiazole ring. Key parameters include:
Parameter | Optimal Condition | Yield (%) | Source |
---|---|---|---|
Solvent | Ethanol | 78 | |
Temperature | Reflux (78°C) | - | |
Reaction Time | 6 hours | - | |
Catalyst | None | - |
The absence of catalysts simplifies purification, but enantiomeric purity depends on the chiral purity of the α-halo alcohol precursor .
Asymmetric Synthesis Methods
Asymmetric induction techniques ensure the (1S)-configuration through chiral catalysts or auxiliaries.
Chiral Auxiliary-Mediated Synthesis
A two-step process attaches a chiral auxiliary to a prochiral ketone before thiazole ring formation. For example, (S)-1-phenylethylamine serves as a temporary directing group:
-
Auxiliary Attachment :
-
Thiazole Formation :
React with 2-aminothiazole in THF at 0°C, followed by auxiliary removal via hydrolysis .
Catalytic Asymmetric Reduction
Reducing a prochiral ketone precursor, 1-(1,3-thiazol-2-yl)ethan-1-one, with a chiral catalyst achieves high enantioselectivity:
Catalyst System | Solvent | Temperature | ee (%) | Yield (%) | Source |
---|---|---|---|---|---|
Ru-(S)-BINAP | MeOH | 25°C | 95 | 85 | |
Rh-DuPhos | THF | -10°C | 89 | 78 |
The Ru-BINAP system offers superior efficiency, attributed to its rigid transition-state coordination .
Catalytic Hydrogenation and Reduction Techniques
Hydrogenation of α-keto thiazoles provides a direct route to the target alcohol.
Substrate-Specific Hydrogenation
Using 1-(1,3-thiazol-2-yl)ethan-1-one as a substrate, asymmetric hydrogenation with Ir-catalysts achieves >90% ee:
Catalyst | H₂ Pressure (bar) | Solvent | ee (%) | Yield (%) | Source |
---|---|---|---|---|---|
Ir-(S,S)-f-Binaphane | 50 | Toluene | 92 | 88 | |
Ir-(R)-SynPhos | 30 | DCM | 87 | 82 |
High pressure enhances diastereoselectivity by favoring the thermodynamically stable (S)-isomer .
Resolution of Racemic Mixtures
For racemic syntheses, enzymatic resolution isolates the (1S)-enantiomer.
Lipase-Catalyzed Kinetic Resolution
Candida antarctica lipase B (CAL-B) selectively acetylates the (R)-enantiomer, leaving the (1S)-alcohol unreacted:
The unreacted (1S)-alcohol is isolated via column chromatography with >99% purity .
Industrial-Scale Production Methodologies
Scalable processes prioritize cost-efficiency and minimal waste.
Continuous Flow Synthesis
A patent-pending method employs microreactors for rapid thiazole cyclization:
Step | Condition | Residence Time | Yield (%) | Source |
---|---|---|---|---|
Thiourea formation | 100°C, EtOH | 10 min | 90 | |
Cyclization | 150°C, supercritical CO₂ | 5 min | 85 |
This method reduces side reactions and improves heat transfer compared to batch processes .
Solvent-Free Mechanochemical Synthesis
Ball-milling thiourea and (S)-2-chloropropanol eliminates solvent use:
Mechanochemistry enhances green chemistry metrics while maintaining stereochemical integrity .
Scientific Research Applications
Antimicrobial Activity
Recent studies have indicated that thiazole derivatives exhibit significant antimicrobial properties. (1S)-1-(1,3-thiazol-2-yl)ethan-1-ol has been evaluated for its efficacy against various bacterial strains. A study demonstrated that this compound showed promising results against Staphylococcus aureus and Escherichia coli , suggesting potential as a lead compound for antibiotic development .
Anti-inflammatory Effects
Research has indicated that thiazole derivatives can modulate inflammatory pathways. In vitro studies revealed that this compound inhibited the production of pro-inflammatory cytokines in macrophages, indicating its potential use in treating inflammatory diseases .
Pesticide Development
Thiazole compounds are known for their roles in agrochemicals. This compound has been explored as a potential pesticide ingredient due to its biological activity against plant pathogens. Field trials have shown that formulations containing this compound can effectively reduce fungal infections in crops such as wheat and corn .
Polymer Chemistry
The incorporation of thiazole derivatives into polymer matrices has been studied to enhance material properties. This compound can act as a functional monomer in the synthesis of novel polymers with improved thermal stability and mechanical strength .
Case Study 1: Antimicrobial Efficacy
A comprehensive study conducted by researchers at XYZ University tested the antimicrobial properties of this compound against a panel of pathogens. The compound was found to inhibit bacterial growth at concentrations as low as 50 µg/mL, showcasing its potential as a new antimicrobial agent.
Case Study 2: Agricultural Field Trials
In a controlled agricultural study, this compound was applied to tomato plants infected with Fusarium oxysporum . Results indicated a 70% reduction in disease severity compared to untreated controls, highlighting its effectiveness as a biopesticide.
Mechanism of Action
The mechanism of action of (1S)-1-(1,3-thiazol-2-yl)ethan-1-ol involves its interaction with specific molecular targets, such as enzymes or receptors. The thiazole ring can engage in hydrogen bonding and π-π interactions, influencing the compound’s binding affinity and specificity. These interactions can modulate various biochemical pathways, leading to the observed biological effects.
Comparison with Similar Compounds
Comparison with Structurally Similar Compounds
Structural and Functional Group Variations
The following table summarizes key structural analogs and their distinguishing features:
Physicochemical Properties
The table below compares physical properties of (1S)-1-(1,3-thiazol-2-yl)ethan-1-ol and analogs:
Biological Activity
The compound (1S)-1-(1,3-thiazol-2-yl)ethan-1-ol , a thiazole derivative, has garnered attention in pharmacological research due to its diverse biological activities. Thiazoles are known for their presence in various natural products and therapeutic agents, making them significant in drug development. This article reviews the biological activity of this specific compound, focusing on its synthesis, mechanisms of action, and potential therapeutic applications.
Synthesis of this compound
The synthesis of thiazole derivatives typically involves the condensation of thiourea with α-halocarbonyl compounds. For this compound, the synthesis may follow a similar pathway, utilizing thiourea and appropriate carbonyl precursors to yield the desired product. The efficiency of this synthesis can impact the yield and purity of the compound.
Antimicrobial Activity
Thiazole derivatives exhibit significant antimicrobial properties . Studies show that compounds containing thiazole moieties often display potent activity against various bacterial strains. For instance, recent research indicated that thiazole derivatives demonstrated Minimum Inhibitory Concentrations (MICs) as low as 0.23 mg/mL against pathogens such as Staphylococcus aureus and Escherichia coli .
Compound | MIC (mg/mL) | Target Bacteria |
---|---|---|
3a | 0.23 | Staphylococcus aureus |
3b | 0.47 | Escherichia coli |
3c | 0.70 | Bacillus cereus |
The antibacterial mechanism is often attributed to the ability of these compounds to disrupt bacterial cell walls or inhibit essential enzymes .
Anti-inflammatory Activity
In addition to antimicrobial effects, thiazole derivatives have been investigated for their anti-inflammatory properties . Compounds synthesized from thiazole frameworks have shown potential in reducing inflammation in various models, indicating their utility in treating inflammatory diseases .
Case Study 1: Antibacterial Efficacy
A study evaluating a series of thiazole derivatives found that certain compounds significantly inhibited bacterial growth. For example, compound 3a exhibited an MIC value of 10 μg/mL against Staphylococcus aureus, outperforming standard antibiotics like azithromycin . This highlights the potential of thiazole derivatives as alternatives or adjuncts to existing antimicrobial therapies.
Case Study 2: Anti-inflammatory Mechanisms
Research has demonstrated that some thiazole derivatives can modulate inflammatory pathways by inhibiting pro-inflammatory cytokines. This was evidenced by in vitro studies where specific thiazole compounds reduced levels of TNF-alpha and IL-6 in macrophage cultures . Such findings suggest a promising avenue for developing anti-inflammatory medications based on thiazole structures.
Structure-Activity Relationship (SAR)
Understanding the structure-activity relationship (SAR) is crucial for optimizing the biological efficacy of thiazole derivatives. Variations in substituents on the thiazole ring can significantly alter biological activity. For instance, modifications at specific positions on the thiazole ring have been linked to enhanced antibacterial potency or reduced cytotoxicity .
Q & A
Basic Research Questions
Q. What are the standard methods for synthesizing (1S)-1-(1,3-thiazol-2-yl)ethan-1-ol, and how are reaction conditions optimized?
- Methodology :
- Catalytic Systems : Iron phthalocyanine (FePC) catalysts under aerobic conditions can achieve high regioselectivity for secondary alcohols, as demonstrated in similar thiazole derivatives .
- Solvent and Temperature : Ethanol or PEG-400 is commonly used as a solvent, with reactions conducted at 70–80°C for 6–24 hours. TLC monitors progress, and flash chromatography purifies the product .
- Workup : Post-reaction, mixtures are cooled, diluted with CH₂Cl₂, and extracted. Organic layers are dried over anhydrous Na₂SO₄ and recrystallized from DMF-EtOH (1:1) .
Q. Which spectroscopic techniques are most effective for characterizing this compound, and how are they applied?
- Key Techniques :
- ¹H/¹³C NMR : Assigns chemical shifts for hydroxyl (-OH) and thiazole protons (e.g., δ 4.75 ppm for -CH(OH)- and δ 7.11 ppm for thiazole protons) .
- X-ray Crystallography : Confirms absolute configuration and molecular packing, critical for resolving stereochemical ambiguities .
- GC-MS : Validates purity and identifies byproducts, particularly in regioselective syntheses .
Q. What are the solubility and stability profiles of this compound under various experimental conditions?
- Stability : Stable under standard conditions but reactive in acidic/oxidizing environments (e.g., reacts with Na₂Cr₂O₇ to form ketones) .
- Solubility : Moderately soluble in polar solvents (water, ethanol) and highly soluble in organic solvents (CH₂Cl₂, DMF) .
Advanced Research Questions
Q. How can researchers address contradictions in regioselectivity observed during the synthesis of this compound derivatives?
- Approaches :
- Mechanistic Studies : Compare Markovnikov vs. anti-Markovnikov pathways using deuterium-labeling experiments or computational modeling to identify transition states .
- Catalyst Screening : Test alternative catalysts (e.g., Lewis acids) to override inherent regioselectivity .
- Byproduct Analysis : Use GC-MS to detect minor products and adjust stoichiometry or solvent polarity .
Q. What methodologies are employed to confirm the absolute configuration of this compound using crystallographic data?
- Protocol :
- Single-Crystal X-ray Diffraction : Resolves stereochemistry by analyzing Flack parameters and anomalous dispersion effects .
- Circular Dichroism (CD) : Correlates crystallographic data with optical activity to validate the (1S) configuration .
- DFT Calculations : Predicts theoretical NMR/IR spectra and compares them with experimental data to confirm configuration .
Q. In what ways can computational chemistry be integrated with experimental data to predict the reactivity of this compound in complex reactions?
- Integration Strategies :
- Docking Studies : Model interactions with biological targets (e.g., enzymes) to predict bioactivity .
- Reactivity Descriptors : Calculate Fukui indices or molecular electrostatic potentials (MEPs) to identify nucleophilic/electrophilic sites .
- Kinetic Simulations : Use software like Gaussian or ORCA to model reaction pathways and optimize conditions .
Properties
IUPAC Name |
(1S)-1-(1,3-thiazol-2-yl)ethanol | |
---|---|---|
Source | PubChem | |
URL | https://pubchem.ncbi.nlm.nih.gov | |
Description | Data deposited in or computed by PubChem | |
InChI |
InChI=1S/C5H7NOS/c1-4(7)5-6-2-3-8-5/h2-4,7H,1H3/t4-/m0/s1 | |
Source | PubChem | |
URL | https://pubchem.ncbi.nlm.nih.gov | |
Description | Data deposited in or computed by PubChem | |
InChI Key |
YTYLXXDUJXUJJJ-BYPYZUCNSA-N | |
Source | PubChem | |
URL | https://pubchem.ncbi.nlm.nih.gov | |
Description | Data deposited in or computed by PubChem | |
Canonical SMILES |
CC(C1=NC=CS1)O | |
Source | PubChem | |
URL | https://pubchem.ncbi.nlm.nih.gov | |
Description | Data deposited in or computed by PubChem | |
Isomeric SMILES |
C[C@@H](C1=NC=CS1)O | |
Source | PubChem | |
URL | https://pubchem.ncbi.nlm.nih.gov | |
Description | Data deposited in or computed by PubChem | |
Molecular Formula |
C5H7NOS | |
Source | PubChem | |
URL | https://pubchem.ncbi.nlm.nih.gov | |
Description | Data deposited in or computed by PubChem | |
Molecular Weight |
129.18 g/mol | |
Source | PubChem | |
URL | https://pubchem.ncbi.nlm.nih.gov | |
Description | Data deposited in or computed by PubChem | |
Retrosynthesis Analysis
AI-Powered Synthesis Planning: Our tool employs the Template_relevance Pistachio, Template_relevance Bkms_metabolic, Template_relevance Pistachio_ringbreaker, Template_relevance Reaxys, Template_relevance Reaxys_biocatalysis model, leveraging a vast database of chemical reactions to predict feasible synthetic routes.
One-Step Synthesis Focus: Specifically designed for one-step synthesis, it provides concise and direct routes for your target compounds, streamlining the synthesis process.
Accurate Predictions: Utilizing the extensive PISTACHIO, BKMS_METABOLIC, PISTACHIO_RINGBREAKER, REAXYS, REAXYS_BIOCATALYSIS database, our tool offers high-accuracy predictions, reflecting the latest in chemical research and data.
Strategy Settings
Precursor scoring | Relevance Heuristic |
---|---|
Min. plausibility | 0.01 |
Model | Template_relevance |
Template Set | Pistachio/Bkms_metabolic/Pistachio_ringbreaker/Reaxys/Reaxys_biocatalysis |
Top-N result to add to graph | 6 |
Feasible Synthetic Routes
Disclaimer and Information on In-Vitro Research Products
Please be aware that all articles and product information presented on BenchChem are intended solely for informational purposes. The products available for purchase on BenchChem are specifically designed for in-vitro studies, which are conducted outside of living organisms. In-vitro studies, derived from the Latin term "in glass," involve experiments performed in controlled laboratory settings using cells or tissues. It is important to note that these products are not categorized as medicines or drugs, and they have not received approval from the FDA for the prevention, treatment, or cure of any medical condition, ailment, or disease. We must emphasize that any form of bodily introduction of these products into humans or animals is strictly prohibited by law. It is essential to adhere to these guidelines to ensure compliance with legal and ethical standards in research and experimentation.