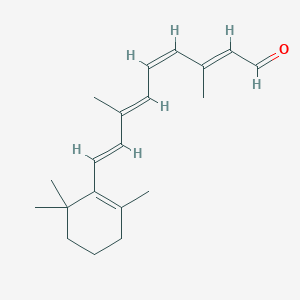
11-cis-Retinal
Overview
Description
11-cis-Retinal is the chromophore essential for vision in vertebrates, forming the light-sensitive component of rhodopsin by covalently binding to opsin proteins in photoreceptor cells . Upon photon absorption, it isomerizes to all-trans-retinal, initiating the phototransduction cascade. Vertebrates rely on the enzymatic retinoid cycle—distinct from invertebrates’ photochemical regeneration—to recycle all-trans-retinal back to this compound, ensuring continuous vision . Structurally, it features a conjugated polyene chain with a cyclohexene ring and a critical cis double bond at the 11th carbon (Fig. 1). This isomer is evolutionarily optimized for rapid photoisomerization and stable interaction with opsin .
Preparation Methods
Chemical Synthesis Approaches to 11-cis-Retinal
11-Yne Precursor Reduction Strategy
The most efficient chemical synthesis employs 11-yne retinoid precursors subjected to stereoselective reduction. Borhan et al. (1999) achieved 92% yield of 11-cis-retinal using Cu/Ag-activated zinc dust in THF at −78°C . This method exploits the trans-addition of hydrogen atoms across the triple bond, with metal activation ensuring precise stereochemical control.
Table 1: Comparative Analysis of Chemical Synthesis Methods
Method | Precursor | Catalyst | Temperature | Yield | Reference |
---|---|---|---|---|---|
Alkyne reduction | 11-yne retinoid | Cu/Ag-Zn dust | −78°C | 92% | |
Wittig olefination | C15-phosphonium | — | 25°C | 68% | |
Horner-Wadsworth-Emmons | β-ionylidene | NaHMDS | 0°C | 74% |
The 11-yne route’s superiority stems from:
-
Minimal isomerization during reduction due to low-temperature conditions
-
Compatibility with sensitive functional groups (e.g., aldehyde moieties)
Retinaldehyde Functionalization Techniques
Alternative approaches modify pre-formed retinaldehyde backbones. GlpBio’s protocol utilizes protected 11-cis-retinal derivatives synthesized via:
-
Trans-to-cis photoisomerization under 450 nm light (32% conversion)
-
Enzymatic oxidation of 11-cis-retinol using NAD+-dependent dehydrogenases
However, these methods face limitations:
-
Photoisomerization produces complex mixtures requiring HPLC purification
-
Enzymatic oxidation achieves only 41% yield due to product inhibition
Enzymatic Isomerization in Biological Systems
RPE65-Catalyzed Isomerization Pathway
The vertebrate visual cycle converts all-trans-retinyl esters to 11-cis-retinol through an RPE65-dependent mechanism . Key steps include:
-
Ester Cleavage : LRAT esterifies all-trans-retinol to retinyl-palmitate
-
Isomerization : RPE65 hydrolyzes esters while isomerizing the C11–C12 bond (ΔG‡ = 18.7 kcal/mol)
-
Oxidation : 11-cis-RDH converts retinol to retinaldehyde (kcat = 2.7 s⁻¹)
Table 2: Enzymatic Isomerization Parameters
Parameter | Value | Conditions | Reference |
---|---|---|---|
RPE65 turnover number | 0.45 min⁻¹ | 37°C, pH 7.4 | |
CRALBP binding affinity | Kd = 38 nM | 11-cis-retinol | |
Isomerization efficiency | 73% | RPE microsomes + ATP |
CRALBP-Mediated Stabilization
Cellular retinaldehyde-binding protein (CRALBP) enhances 11-cis-retinal production by:
-
Shifting isomerization equilibrium via product sequestration
-
Protecting 11-cis-retinol from nonenzymatic isomerization (t₁/₂ increases from 2.1 h to 19.4 h)
-
Facilitating membrane extraction through hydrophobic interactions
Photoisomerization and Nonenzymatic Methods
UV-Induced Isomerization
RPE microsomes catalyze light-dependent 11-cis → all-trans isomerization at 350–400 nm :
Table 3: Photochemical Isomerization Parameters
Substrate | λmax (nm) | Isomerization Rate (s⁻¹) |
---|---|---|
11-cis-retinol | 328 | 0.47 ± 0.08 |
11-cis-retinyl palmitate | 316 | 0.29 ± 0.05 |
Solubility-Driven Reconstitution
The low aqueous solubility of 11-cis-retinal (0.11 μM) necessitates delivery vehicles for in vitro applications:
Table 4: Solubility Enhancement Strategies
Vehicle | Solubility (μM) | Regeneration Rate (τ, min) |
---|---|---|
Lipid vesicles | 8.2 | 4.7 ± 0.9 |
IRBP complexes | 14.5 | 2.1 ± 0.3 |
BSA conjugates | 9.8 | 3.4 ± 0.6 |
4-OH derivatives show improved solubility (0.55 μM) but reduced membrane partitioning (LogP = 3.1 vs. 4.7 for native retinal) .
Industrial-Scale Production Challenges
Thermodynamic Instability
The 11-cis configuration’s high strain energy (ΔG = +4.2 kcal/mol vs. all-trans) necessitates:
Purification Requirements
HPLC purification achieves >99% enantiomeric purity using:
Chemical Reactions Analysis
Types of Reactions
Rhodopsin undergoes several types of chemical reactions, primarily involving its retinal chromophore. The most notable reaction is the photoisomerization of 11-cis-retinal to all-trans-retinal upon absorption of a photon. This isomerization triggers a series of conformational changes in the opsin protein, leading to the activation of the phototransduction cascade .
Common Reagents and Conditions
The photoisomerization reaction of rhodopsin requires light as the primary reagent. In laboratory settings, this reaction can be studied using high-performance liquid chromatography (HPLC) to analyze the isomerization products .
Major Products Formed
The primary product of the photoisomerization reaction is all-trans-retinal. This molecule subsequently dissociates from the opsin protein, leading to the formation of metarhodopsin II, an activated state of rhodopsin that interacts with the G-protein transducin to propagate the visual signal .
Scientific Research Applications
Role in Vision and Phototransduction
11-cis-retinal is essential for the functioning of opsins, which are light-sensitive proteins in photoreceptor cells. When light hits this compound, it isomerizes to all-trans-retinal, activating the opsin and triggering a cascade of biochemical reactions that result in visual signal transduction. This process is fundamental to both rod and cone photoreceptors:
- Rod Photoreceptors : Primarily responsible for vision in low-light conditions, relying heavily on this compound for rhodopsin regeneration.
- Cone Photoreceptors : Responsible for color vision and functioning under bright light conditions, utilizing different opsins that also depend on this compound.
Biotechnology and Therapeutic Applications
Recent studies have explored this compound's potential in treating retinal degenerative diseases, particularly age-related macular degeneration (AMD). Researchers are investigating biomaterials-based drug delivery systems that incorporate this compound to enhance therapeutic efficacy:
- Drug Delivery Devices : These devices can facilitate the sustained release of this compound to promote photoreceptor health and function .
- Restoration of Vision : By regenerating visual pigments in damaged retinas, this compound may help restore some degree of vision in patients with specific retinal pathologies .
Optogenetics
Optogenetics leverages light-sensitive proteins to control neuronal activity with light. This compound serves as a cofactor for opsins used in optogenetic tools:
- Opto-mGluR6 : A next-generation optogenetic tool that utilizes this compound to restore ON pathways in blind retinas, showing promise for therapeutic interventions .
- Molecular Switches : Studies have proposed using this compound as a molecular switch in electronic applications due to its sensitivity to light and ability to undergo reversible isomerization .
Case Study 1: Retinal Regeneration
A study investigated the effects of introducing this compound into retinal organoids derived from stem cells. The results indicated improved photoreceptor development and functionality, suggesting that this compound could enhance regenerative therapies for retinal diseases .
Case Study 2: Visual Cycle Dynamics
Research on the visual cycle has highlighted how disruptions in the synthesis or regeneration of this compound can lead to visual impairments. For instance, mutations affecting enzymes involved in the conversion of all-trans-retinol back to this compound can cause conditions like fundus albipunctatus .
Comparative Data Table
Mechanism of Action
Rhodopsin exerts its effects through a well-defined mechanism involving the activation of the phototransduction cascade. Upon absorption of light, 11-cis-retinal isomerizes to all-trans-retinal, causing a conformational change in the opsin protein. This change activates the G-protein transducin, which in turn activates phosphodiesterase, leading to a decrease in cyclic guanosine monophosphate (cGMP) levels. The reduction in cGMP causes the closure of cGMP-gated ion channels, resulting in hyperpolarization of the rod cell and the transmission of the visual signal to the brain .
Comparison with Similar Compounds
Comparison with Structural Isomers
2.1 7-cis-, 9-cis-, and 13-cis-Retinal
Alternative cis isomers differ in double-bond positions, leading to distinct spectral and functional properties:
Isomer | Key Structural Feature | Binding Affinity to Opsin (ΔG, kcal/mol) | Spectral λmax (nm) | Functional Efficiency |
---|---|---|---|---|
11-cis | 11th carbon cis bond | 0.0 (reference) | ~500 | Optimal for vision |
9-cis | 9th carbon cis bond | +2.9–5.4 | ~485 | Reduced activity |
13-cis | 13th carbon cis bond | +3.1–8.9 | ~470 | Minimal activity |
- 9-cis-Retinal : Forms isorhodopsin but exhibits 20% lower quantum efficiency in phototransduction compared to 11-cis-retinal . Molecular dynamics reveal higher conformational strain in opsin binding pockets, reducing stability .
- 13-cis-Retinal : Poorly fits the opsin binding site due to steric clashes, resulting in negligible photoresponse .
- 7-cis-Retinal : Rarely studied; computational models suggest destabilizing torsional angles in the polyene chain .
Evolutionary Preference : The 11-cis configuration balances steric accessibility, spectral tuning, and isomerization kinetics, making it the biological standard .
2.2 All-trans-Retinal
The photoproduct of 11-cis-retinal, all-trans-retinal, is central to the retinoid cycle but cannot bind opsin. Key differences:
Property | 11-cis-Retinal | All-trans-Retinal |
---|---|---|
Function | Rhodopsin formation | Retinoid cycle substrate |
Binding to IRBP | Kd = 0.15 μM | Kd = 0.45 μM |
Enzymatic Recycling | Requires RPE65 isomerase | Generated via light absorption |
All-trans-retinal exhibits weaker binding to interphotoreceptor retinoid-binding protein (IRBP), slowing its transport compared to this compound .
Functional Analogs in Metabolism
3.1 Retinol (Vitamin A Alcohol)
Retinol serves as a storage form and precursor for this compound. Unlike this compound, it:
- Lacks the aldehyde group, preventing direct opsin binding.
- Acts as a cofactor in enzymatic processes (e.g., glycoprotein synthesis) .
- Requires oxidation to retinaldehyde via retinol dehydrogenases for visual function .
3.2 All-trans-Retinoic Acid (ATRA)
ATRA regulates gene expression via nuclear receptors but cannot participate in vision due to:
- Irreversible oxidation of the aldehyde group to a carboxylic acid.
- Inability to isomerize to 11-cis configuration .
4.1 5,6-Dihydroretinal
A saturated-ring analog tested in bovine opsin:
- Forms a visual pigment with λmax shifted to 460 nm (vs. 500 nm for native rhodopsin).
- Exhibits slower photobleaching kinetics, highlighting the necessity of the conjugated cyclohexene ring for rapid signal transduction .
4.2 9-cis-3,4-Didehydroretinal
Used in insect studies, this analog shows reduced binding to CRALBP (cellular retinaldehyde-binding protein), impairing retinal recycling efficiency .
Protein Binding Specificity
5.1 IRBP Interactions
This compound binds IRBP with higher affinity (Kd = 0.15 μM) than all-trans-retinol (Kd = 0.45 μM).
5.2 ABCR ATPase Activation
This compound stimulates ABCR ATPase activity 3-fold more effectively than all-trans-retinol, underscoring its role in retinoid transport across photoreceptor membranes .
Therapeutic Implications
Biological Activity
11-cis-Retinal is a vital chromophore in vertebrate vision, playing a crucial role in the phototransduction cascade. It is derived from vitamin A and serves as the light-absorbing molecule in both rod and cone photoreceptors. The biological activity of this compound involves its interaction with opsins, leading to the initiation of visual signaling processes.
This compound binds to opsins, forming a covalent bond that triggers conformational changes essential for phototransduction. In rods, this binding activates the phototransduction cascade, while in cones, it acts as an inverse agonist, deactivating the signaling pathway. This dual role contributes to the differing rates of dark adaptation between rods and cones .
Table 1: Effects of this compound on Phototransduction
Photoreceptor Type | Effect of this compound | Outcome |
---|---|---|
Rods | Activates phototransduction cascade | Delays dark adaptation |
Cones | Deactivates phototransduction | Facilitates rapid recovery of sensitivity |
Production and Regeneration
The regeneration of this compound is primarily mediated by the retinoid cycle within the retinal pigment epithelium (RPE). Key enzymes involved include RPE65, which catalyzes the conversion of all-trans-retinyl esters to 11-cis-retinol, followed by oxidation to this compound by RDH5 . This cycle is essential for maintaining visual function, especially after exposure to bright light.
Case Studies
-
Photic Generation of this compound :
A study demonstrated that illumination at specific wavelengths (e.g., 530 nm) significantly enhances the production of this compound from RPE membranes. Under optimal conditions, a production rate of approximately 1 nmol/min/mg crude protein was observed, indicating a robust capacity for visual pigment regeneration . -
Genetic Studies on RGR :
Research involving RGR (retinal pigment epithelium-specific G-protein coupled receptor) has shown its role in mobilizing retinyl esters and enhancing RPE65 activity. Genetic mutations affecting RGR have been linked to rod-cone dystrophy, highlighting its importance in retinal health and function .
Implications for Vision Science
The biological activity of this compound extends beyond mere light detection; it is integral to understanding various visual disorders. Deficiencies in its production or regeneration can lead to significant visual impairments. For instance, mutations in genes involved in the retinoid cycle can result in conditions such as fundus albipunctatus or retinitis pigmentosa .
Q & A
Basic Research Questions
Q. What experimental methods are most effective for determining the stability of 11-cis-retinal compared to its isomers?
- Methodological Answer : Use UV-Vis spectroscopy to monitor isomerization kinetics under controlled light exposure. Fluorescence quenching assays can quantify interactions with opsin or IRBP (interphotoreceptor retinoid-binding protein). For thermodynamic stability, employ HPLC to separate isomers and calculate energy barriers using Arrhenius plots .
- Example Data :
Isomer | Half-life (min, 500 nm light) | ΔG (kJ/mol) |
---|---|---|
11-cis | 8.2 ± 1.3 | 42.1 |
all-trans | 25.6 ± 3.1 | 28.7 |
Q. How can researchers assess the binding affinity of this compound to retinal proteins like IRBP?
- Methodological Answer : Perform titration experiments using isothermal titration calorimetry (ITC) or surface plasmon resonance (SPR). For example, ITC can quantify the dissociation constant (Kd) in the presence of modulators like docosahexaenoic acid (DHA), which alters binding kinetics .
- Key Consideration : Account for biphasic binding curves (e.g., in DHA-treated IRBP) using software like BIOEQS to model multiple equilibria .
Q. What in vivo models are suitable for studying this compound regeneration in retinal diseases?
- Methodological Answer : Use Rpe65<sup>−/−</sup> mice to test exogenous this compound administration. Measure recovery via electroretinography (ERG) and rhodopsin regeneration assays. Monitor opsin phosphorylation levels to evaluate functional rescue .
Advanced Research Questions
Q. How can computational modeling resolve contradictions in this compound’s stability and its selection in rhodopsin?
- Methodological Answer : Apply quantum mechanics/molecular mechanics (QM/MM) simulations to analyze electrostatic interactions between this compound and opsin. Compare energy landscapes of 11-cis vs. 7-cis/9-cis isomers to identify stabilizing residues (e.g., Schiff base linkage with Lys296) .
- Case Study : A 2011 study found that this compound’s electrostatic fit with opsin reduces steric strain, favoring its selection despite lower thermodynamic stability .
Q. What experimental designs address conflicting data on enzymatic pathways for this compound synthesis in humans vs. insects?
- Methodological Answer : Use X-ray crystallography to compare NinaB (insect) and RPE65 (human) structures. Perform mutagenesis on catalytic sites (e.g., Fe<sup>2+</sup> binding in RPE65) and assay isomerization efficiency via LC-MS .
- Key Finding : Despite structural homology, NinaB uses a radical mechanism, while RPE65 relies on hydride transfer, explaining differential sensitivity to inhibitors .
Q. How can researchers evaluate the efficacy of retinoid cycle inhibitors on this compound recovery?
- Methodological Answer : Administer all-trans-Ret-NH2 to light-exposed mice and quantify this compound levels via HPLC. Compare recovery rates (e.g., 50% recovery at 3 days vs. 10 days for high-dose inhibitors) and correlate with retinyl ester accumulation .
- Data Interpretation : Use nonlinear regression to model inhibition kinetics and identify dose-dependent thresholds for therapeutic applications.
Q. Methodological Frameworks
Q. How to apply FINER criteria to formulate this compound research questions?
- Feasible : Ensure access to specialized techniques (e.g., cryo-EM for protein-ligand studies).
- Novel : Investigate understudied modulators (e.g., DHA’s role in IRBP binding ).
- Ethical : Use ARVO guidelines for animal studies, minimizing light-induced retinal damage .
Q. What statistical approaches ensure reproducibility in studies of this compound dynamics?
- Methodological Answer : Apply robust multichip analysis (RMA) for gene expression data and use ≥3 biological replicates. Normalize results to internal controls (e.g., opsin phosphorylation baselines) .
Q. Tables for Key Findings
Table 1 : Inhibitor Effects on this compound Recovery (Adapted from )
Inhibitor Dose (µmol) | Recovery Time (Days) | Retinyl Ester Accumulation (nmol/g) |
---|---|---|
1.75 | 3 | 12.4 ± 1.2 |
17.5 | 10 | 34.7 ± 3.5 |
Table 2 : Comparative Enzymology of this compound Synthesis
Enzyme | Mechanism | Catalytic Site | Inhibitor Sensitivity |
---|---|---|---|
RPE65 | Hydride transfer | Fe<sup>2+</sup> | High (Ret-NH2) |
NinaB | Radical isomerization | Non-heme iron | Low |
Properties
IUPAC Name |
(2E,4Z,6E,8E)-3,7-dimethyl-9-(2,6,6-trimethylcyclohexen-1-yl)nona-2,4,6,8-tetraenal | |
---|---|---|
Source | PubChem | |
URL | https://pubchem.ncbi.nlm.nih.gov | |
Description | Data deposited in or computed by PubChem | |
InChI |
InChI=1S/C20H28O/c1-16(8-6-9-17(2)13-15-21)11-12-19-18(3)10-7-14-20(19,4)5/h6,8-9,11-13,15H,7,10,14H2,1-5H3/b9-6-,12-11+,16-8+,17-13+ | |
Source | PubChem | |
URL | https://pubchem.ncbi.nlm.nih.gov | |
Description | Data deposited in or computed by PubChem | |
InChI Key |
NCYCYZXNIZJOKI-IOUUIBBYSA-N | |
Source | PubChem | |
URL | https://pubchem.ncbi.nlm.nih.gov | |
Description | Data deposited in or computed by PubChem | |
Canonical SMILES |
CC1=C(C(CCC1)(C)C)C=CC(=CC=CC(=CC=O)C)C | |
Source | PubChem | |
URL | https://pubchem.ncbi.nlm.nih.gov | |
Description | Data deposited in or computed by PubChem | |
Isomeric SMILES |
CC1=C(C(CCC1)(C)C)/C=C/C(=C/C=C\C(=C\C=O)\C)/C | |
Source | PubChem | |
URL | https://pubchem.ncbi.nlm.nih.gov | |
Description | Data deposited in or computed by PubChem | |
Molecular Formula |
C20H28O | |
Source | PubChem | |
URL | https://pubchem.ncbi.nlm.nih.gov | |
Description | Data deposited in or computed by PubChem | |
DSSTOX Substance ID |
DTXSID80415057 | |
Record name | 11-cis-Retinal | |
Source | EPA DSSTox | |
URL | https://comptox.epa.gov/dashboard/DTXSID80415057 | |
Description | DSSTox provides a high quality public chemistry resource for supporting improved predictive toxicology. | |
Molecular Weight |
284.4 g/mol | |
Source | PubChem | |
URL | https://pubchem.ncbi.nlm.nih.gov | |
Description | Data deposited in or computed by PubChem | |
Physical Description |
Solid | |
Record name | 11-cis-Retinaldehyde | |
Source | Human Metabolome Database (HMDB) | |
URL | http://www.hmdb.ca/metabolites/HMDB0002152 | |
Description | The Human Metabolome Database (HMDB) is a freely available electronic database containing detailed information about small molecule metabolites found in the human body. | |
Explanation | HMDB is offered to the public as a freely available resource. Use and re-distribution of the data, in whole or in part, for commercial purposes requires explicit permission of the authors and explicit acknowledgment of the source material (HMDB) and the original publication (see the HMDB citing page). We ask that users who download significant portions of the database cite the HMDB paper in any resulting publications. | |
CAS No. |
564-87-4 | |
Record name | 11-cis-Retinal | |
Source | CAS Common Chemistry | |
URL | https://commonchemistry.cas.org/detail?cas_rn=564-87-4 | |
Description | CAS Common Chemistry is an open community resource for accessing chemical information. Nearly 500,000 chemical substances from CAS REGISTRY cover areas of community interest, including common and frequently regulated chemicals, and those relevant to high school and undergraduate chemistry classes. This chemical information, curated by our expert scientists, is provided in alignment with our mission as a division of the American Chemical Society. | |
Explanation | The data from CAS Common Chemistry is provided under a CC-BY-NC 4.0 license, unless otherwise stated. | |
Record name | Retinal, (11Z)- | |
Source | ChemIDplus | |
URL | https://pubchem.ncbi.nlm.nih.gov/substance/?source=chemidplus&sourceid=0000564874 | |
Description | ChemIDplus is a free, web search system that provides access to the structure and nomenclature authority files used for the identification of chemical substances cited in National Library of Medicine (NLM) databases, including the TOXNET system. | |
Record name | 11-cis-Retinal | |
Source | EPA DSSTox | |
URL | https://comptox.epa.gov/dashboard/DTXSID80415057 | |
Description | DSSTox provides a high quality public chemistry resource for supporting improved predictive toxicology. | |
Record name | RETINAL, (11Z)- | |
Source | FDA Global Substance Registration System (GSRS) | |
URL | https://gsrs.ncats.nih.gov/ginas/app/beta/substances/91058V6HCH | |
Description | The FDA Global Substance Registration System (GSRS) enables the efficient and accurate exchange of information on what substances are in regulated products. Instead of relying on names, which vary across regulatory domains, countries, and regions, the GSRS knowledge base makes it possible for substances to be defined by standardized, scientific descriptions. | |
Explanation | Unless otherwise noted, the contents of the FDA website (www.fda.gov), both text and graphics, are not copyrighted. They are in the public domain and may be republished, reprinted and otherwise used freely by anyone without the need to obtain permission from FDA. Credit to the U.S. Food and Drug Administration as the source is appreciated but not required. | |
Record name | 11-cis-Retinaldehyde | |
Source | Human Metabolome Database (HMDB) | |
URL | http://www.hmdb.ca/metabolites/HMDB0002152 | |
Description | The Human Metabolome Database (HMDB) is a freely available electronic database containing detailed information about small molecule metabolites found in the human body. | |
Explanation | HMDB is offered to the public as a freely available resource. Use and re-distribution of the data, in whole or in part, for commercial purposes requires explicit permission of the authors and explicit acknowledgment of the source material (HMDB) and the original publication (see the HMDB citing page). We ask that users who download significant portions of the database cite the HMDB paper in any resulting publications. | |
Melting Point |
63.5 - 64.4 °C | |
Record name | 11-cis-Retinaldehyde | |
Source | Human Metabolome Database (HMDB) | |
URL | http://www.hmdb.ca/metabolites/HMDB0002152 | |
Description | The Human Metabolome Database (HMDB) is a freely available electronic database containing detailed information about small molecule metabolites found in the human body. | |
Explanation | HMDB is offered to the public as a freely available resource. Use and re-distribution of the data, in whole or in part, for commercial purposes requires explicit permission of the authors and explicit acknowledgment of the source material (HMDB) and the original publication (see the HMDB citing page). We ask that users who download significant portions of the database cite the HMDB paper in any resulting publications. | |
Retrosynthesis Analysis
AI-Powered Synthesis Planning: Our tool employs the Template_relevance Pistachio, Template_relevance Bkms_metabolic, Template_relevance Pistachio_ringbreaker, Template_relevance Reaxys, Template_relevance Reaxys_biocatalysis model, leveraging a vast database of chemical reactions to predict feasible synthetic routes.
One-Step Synthesis Focus: Specifically designed for one-step synthesis, it provides concise and direct routes for your target compounds, streamlining the synthesis process.
Accurate Predictions: Utilizing the extensive PISTACHIO, BKMS_METABOLIC, PISTACHIO_RINGBREAKER, REAXYS, REAXYS_BIOCATALYSIS database, our tool offers high-accuracy predictions, reflecting the latest in chemical research and data.
Strategy Settings
Precursor scoring | Relevance Heuristic |
---|---|
Min. plausibility | 0.01 |
Model | Template_relevance |
Template Set | Pistachio/Bkms_metabolic/Pistachio_ringbreaker/Reaxys/Reaxys_biocatalysis |
Top-N result to add to graph | 6 |
Feasible Synthetic Routes
Disclaimer and Information on In-Vitro Research Products
Please be aware that all articles and product information presented on BenchChem are intended solely for informational purposes. The products available for purchase on BenchChem are specifically designed for in-vitro studies, which are conducted outside of living organisms. In-vitro studies, derived from the Latin term "in glass," involve experiments performed in controlled laboratory settings using cells or tissues. It is important to note that these products are not categorized as medicines or drugs, and they have not received approval from the FDA for the prevention, treatment, or cure of any medical condition, ailment, or disease. We must emphasize that any form of bodily introduction of these products into humans or animals is strictly prohibited by law. It is essential to adhere to these guidelines to ensure compliance with legal and ethical standards in research and experimentation.