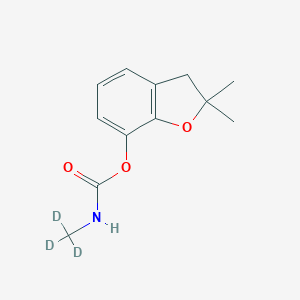
Carbofuran-d3
Overview
Description
Synthesis Analysis
The synthesis of Carbofuran-d3 involves specialized methods to incorporate deuterium atoms into the Carbofuran molecule. While specific synthesis pathways for Carbofuran-d3 are not directly found, studies on related carbocyclic systems and the synthesis of tetrahydrofuran derivatives provide insight into complex synthesis strategies that could be adapted for Carbofuran-d3. These include methods like palladium-catalyzed carboetherification and carboamination reactions, which are powerful tools for constructing heterocycles from simple starting materials (Wolfe, 2007).
Molecular Structure Analysis
Molecular structure analysis of Carbofuran-d3, like its parent compound Carbofuran, would involve understanding the arrangement of atoms and the incorporation of deuterium. Techniques such as NMR could be pivotal in elucidating these structural details, as demonstrated in studies on related compounds where NMR studies helped understand the solution structures of oligomers derived from tetrahydrofuran amino acids (Hungerford et al., 2000).
Chemical Reactions and Properties
Carbofuran-d3, by virtue of its structural similarity to Carbofuran, is expected to undergo similar chemical reactions, including hydrolysis, oxidation, and photodegradation. Studies on Carbofuran have documented various degradation pathways, such as hydrolysis to 3-hydroxycarbofuran and photodegradation leading to multiple photoproducts, which could similarly apply to Carbofuran-d3 with considerations for the effects of deuterium substitution (Raha & Das, 1990).
Physical Properties Analysis
The physical properties of Carbofuran-d3, including its boiling point, melting point, and solubility, are expected to be slightly altered compared to Carbofuran due to the presence of deuterium. These properties are crucial for understanding the behavior of Carbofuran-d3 in various environments and for developing analytical methods for its detection and quantification, as seen in studies employing voltammetric detection techniques for Carbofuran (Jirasirichote et al., 2017).
Chemical Properties Analysis
The chemical properties analysis of Carbofuran-d3 would focus on its reactivity, stability, and interaction with biological systems, which might be influenced by the deuterium atoms. This includes its metabolism by cytochrome P450 in various species, offering insights into its biotransformation and the role of specific enzymes in this process (Usmani, Hodgson, & Rose, 2004).
Scientific Research Applications
Degradation by Bacteria
Chryseobacterium sp. BSC2-3, a carbofuran-degrading bacterium, transforms carbofuran into 3-hydroxycarbofuran. This process leads to metabolic changes, producing disease resistance inducers and plant growth regulators (Park et al., 2022).
Ecotoxic Effects on Fish
Interaction between carbofuran and carbon nanotubes enhances ecotoxic effects on the gills of Nile tilapia (Campos-Garcia et al., 2016).
Effects on Heart Tissue in Rats
Exposure to carbofuran in rats resulted in decreased heart tissue lactate dehydrogenase activity and increased malondialdehyde levels. Pretreatment with curcumin reversed these effects (Jaiswal et al., 2017).
Photo-Fenton Degradation
Carbofuran degradation and mineralization efficiencies increase with higher H2O2 and Fe3+ dosages and decrease with higher carbofuran concentrations (Lu et al., 2012).
Detection in Food
A specific and sensitive monoclonal antibody has been developed for the detection of carbofuran in fruits and vegetables (Yao et al., 2017).
Effect on Microorganisms
Application of carbofuran resulted in 100-300% increases in bacterial plus actinomycetal populations and an extended period of CO2 evolution (Mathur et al., 1976).
Nephrotoxicity
Carbofuran exhibits nephrotoxic effects, possibly due to increased oxidative stress (Kaur et al., 2012).
Electrochemical Sensor Development
A highly sensitive and selective electrochemical sensor based on Gadolinium Sulfide Rod-Embedded RGO has been developed for carbofuran detection, suitable for environmental and food sample testing (Mariyappan et al., 2021).
Voltammetric Detection
An accurate and precise electrochemical method using screen-printed carbon electrodes modified with graphene oxide and gold nanoparticles has been developed for carbofuran detection, promising for simple, low-cost analysis in agricultural fields (Jirasirichote et al., 2017).
Aquatic Fate and Toxicity
Carbofuran, a widely used insecticide, has a short environmental half-life but is highly toxic to fish, with LC50s below 1 mg/l (Trotter et al., 1991).
Safety And Hazards
properties
IUPAC Name |
(2,2-dimethyl-3H-1-benzofuran-7-yl) N-(trideuteriomethyl)carbamate | |
---|---|---|
Source | PubChem | |
URL | https://pubchem.ncbi.nlm.nih.gov | |
Description | Data deposited in or computed by PubChem | |
InChI |
InChI=1S/C12H15NO3/c1-12(2)7-8-5-4-6-9(10(8)16-12)15-11(14)13-3/h4-6H,7H2,1-3H3,(H,13,14)/i3D3 | |
Source | PubChem | |
URL | https://pubchem.ncbi.nlm.nih.gov | |
Description | Data deposited in or computed by PubChem | |
InChI Key |
DUEPRVBVGDRKAG-HPRDVNIFSA-N | |
Source | PubChem | |
URL | https://pubchem.ncbi.nlm.nih.gov | |
Description | Data deposited in or computed by PubChem | |
Canonical SMILES |
CC1(CC2=C(O1)C(=CC=C2)OC(=O)NC)C | |
Source | PubChem | |
URL | https://pubchem.ncbi.nlm.nih.gov | |
Description | Data deposited in or computed by PubChem | |
Isomeric SMILES |
[2H]C([2H])([2H])NC(=O)OC1=CC=CC2=C1OC(C2)(C)C | |
Source | PubChem | |
URL | https://pubchem.ncbi.nlm.nih.gov | |
Description | Data deposited in or computed by PubChem | |
Molecular Formula |
C12H15NO3 | |
Source | PubChem | |
URL | https://pubchem.ncbi.nlm.nih.gov | |
Description | Data deposited in or computed by PubChem | |
DSSTOX Substance ID |
DTXSID70583577 | |
Record name | 2,2-Dimethyl-2,3-dihydro-1-benzofuran-7-yl (~2~H_3_)methylcarbamate | |
Source | EPA DSSTox | |
URL | https://comptox.epa.gov/dashboard/DTXSID70583577 | |
Description | DSSTox provides a high quality public chemistry resource for supporting improved predictive toxicology. | |
Molecular Weight |
224.27 g/mol | |
Source | PubChem | |
URL | https://pubchem.ncbi.nlm.nih.gov | |
Description | Data deposited in or computed by PubChem | |
Product Name |
Carbofuran-d3 | |
CAS RN |
1007459-98-4 | |
Record name | 2,2-Dimethyl-2,3-dihydro-1-benzofuran-7-yl (~2~H_3_)methylcarbamate | |
Source | EPA DSSTox | |
URL | https://comptox.epa.gov/dashboard/DTXSID70583577 | |
Description | DSSTox provides a high quality public chemistry resource for supporting improved predictive toxicology. | |
Record name | 1007459-98-4 | |
Source | European Chemicals Agency (ECHA) | |
URL | https://echa.europa.eu/information-on-chemicals | |
Description | The European Chemicals Agency (ECHA) is an agency of the European Union which is the driving force among regulatory authorities in implementing the EU's groundbreaking chemicals legislation for the benefit of human health and the environment as well as for innovation and competitiveness. | |
Explanation | Use of the information, documents and data from the ECHA website is subject to the terms and conditions of this Legal Notice, and subject to other binding limitations provided for under applicable law, the information, documents and data made available on the ECHA website may be reproduced, distributed and/or used, totally or in part, for non-commercial purposes provided that ECHA is acknowledged as the source: "Source: European Chemicals Agency, http://echa.europa.eu/". Such acknowledgement must be included in each copy of the material. ECHA permits and encourages organisations and individuals to create links to the ECHA website under the following cumulative conditions: Links can only be made to webpages that provide a link to the Legal Notice page. | |
Synthesis routes and methods I
Procedure details
Synthesis routes and methods II
Procedure details
Synthesis routes and methods III
Procedure details
Retrosynthesis Analysis
AI-Powered Synthesis Planning: Our tool employs the Template_relevance Pistachio, Template_relevance Bkms_metabolic, Template_relevance Pistachio_ringbreaker, Template_relevance Reaxys, Template_relevance Reaxys_biocatalysis model, leveraging a vast database of chemical reactions to predict feasible synthetic routes.
One-Step Synthesis Focus: Specifically designed for one-step synthesis, it provides concise and direct routes for your target compounds, streamlining the synthesis process.
Accurate Predictions: Utilizing the extensive PISTACHIO, BKMS_METABOLIC, PISTACHIO_RINGBREAKER, REAXYS, REAXYS_BIOCATALYSIS database, our tool offers high-accuracy predictions, reflecting the latest in chemical research and data.
Strategy Settings
Precursor scoring | Relevance Heuristic |
---|---|
Min. plausibility | 0.01 |
Model | Template_relevance |
Template Set | Pistachio/Bkms_metabolic/Pistachio_ringbreaker/Reaxys/Reaxys_biocatalysis |
Top-N result to add to graph | 6 |
Feasible Synthetic Routes
Citations
Disclaimer and Information on In-Vitro Research Products
Please be aware that all articles and product information presented on BenchChem are intended solely for informational purposes. The products available for purchase on BenchChem are specifically designed for in-vitro studies, which are conducted outside of living organisms. In-vitro studies, derived from the Latin term "in glass," involve experiments performed in controlled laboratory settings using cells or tissues. It is important to note that these products are not categorized as medicines or drugs, and they have not received approval from the FDA for the prevention, treatment, or cure of any medical condition, ailment, or disease. We must emphasize that any form of bodily introduction of these products into humans or animals is strictly prohibited by law. It is essential to adhere to these guidelines to ensure compliance with legal and ethical standards in research and experimentation.