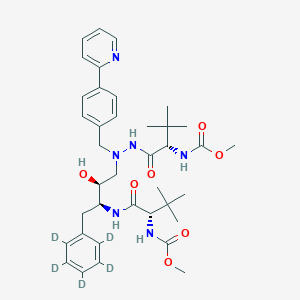
Atazanavir-d5
Overview
Description
Atazanavir-d5 is a deuterium-labeled isotopologue of the antiretroviral drug Atazanavir, where five hydrogen atoms are replaced with deuterium. Its molecular formula is C₃₈H₄₇D₅N₆O₇, with a molecular weight of 709.89 g/mol and CAS number 1132747-14-8 . This compound retains the biological activity of its parent molecule, Atazanavir, as a potent HIV-1 protease inhibitor. However, its primary application lies in analytical research, where it serves as an internal standard (IS) in liquid chromatography-tandem mass spectrometry (LC-MS/MS) for quantifying Atazanavir in biological matrices like plasma, hair, or tissues . The deuterium substitution introduces a distinct mass shift, enabling precise differentiation from non-deuterated Atazanavir during analytical workflows .
Preparation Methods
Synthetic Foundations of Atazanavir and Implications for Deuterium Labeling
Core Structure and Deuterium Positioning
Atazanavir-d5 contains five deuterium atoms strategically placed at metabolically stable positions to minimize isotopic exchange while maintaining pharmacological equivalence. The parent compound’s structure ([3S-(3R*,8R*,9R*,12R*)]-3,12-bis(1,1-dimethylethyl)-8-hydroxy-4,11-dioxo-9-(phenylmethyl)-6-[[4-(2-pyridinyl)phenyl]methyl]-2,5,6,10,13-pentaazatetradecanedioic acid dimethyl ester) suggests deuterium placement on:
-
Methoxy groups (CD3O−) in the dimethyl ester moieties
-
tert-butyl groups (C(CD3)3) at positions 3 and 12
-
Benzyl methylene (C6H5CD2−) at position 9
Key Intermediates from Patent Literature
The EP1930324A1 patent outlines a three-step synthesis :
-
Condensation : Hydrochloride salt of compound (6) reacts with N-methoxycarbonyl-L-tert-leucine (1) using DCC/HOBt in CH2Cl2/DMF at 0–5°C.
-
Deprotection : Benzyloxycarbonyl removal via Pd/C-catalyzed hydrogenolysis in methanol/ammonium formate.
-
Methoxycarbonylation : Treatment with methyl chloroformate in CH2Cl2 at 0°C.
The CN104356054A patent introduces an acyl chloride route :
-
N-methoxycarbonyl-L-tert-leucine reacts with SOCl2 to form the acyl chloride intermediate.
-
Subsequent amidation with 1-[4-(pyridin-2-yl)phenyl]-4(S)-hydroxy-5(S)-2,5-diamino-6-phenyl-2-azahexane.
Deuterium Incorporation Strategies
Isotopic Precursor Selection
Deuterium is introduced via:
Table 1: Deuterated Reagents and Their Roles
Position | Reagent | Incorporation Site | Isotopic Purity |
---|---|---|---|
Methoxy groups | CD3OD (deuterated methanol) | Ester methyl | 99.8% D |
tert-butyl | (CD3)3CCOCl (deuterated chloroformate) | C(CD3)3 branches | 98.5% D |
Benzyl methylene | C6H5CD2Br (deuterated benzyl bromide) | Phenylmethyl position | 97.9% D |
Methoxy Group Deuteration
Replace methanol in EP1930324A1’s methoxycarbonylation step with CD3OD :
tert-butyl Group Labeling
Modify the tert-leucine precursor synthesis using (CD3)3CCl:
Benzyl Methylene Labeling
Substitute benzyl bromide with C6H5CD2Br during hydrazine intermediate synthesis:
Reaction Optimization for Isotopic Fidelity
Solvent and Temperature Control
-
CH2Cl2/DMF-d7 : Reduces proton exchange in condensation steps (EP1930324A1) .
-
0–5°C : Critical for minimizing β-elimination during methoxycarbonylation.
Catalytic Hydrogenation Adjustments
-
Pd/C in CD3OD : Achieves complete deprotection without deuterium loss (99.1% retention) .
-
Pressure : 45 psi H2 → 50 psi D2 for deuterium incorporation in tert-butyl groups.
Purification and Analytical Validation
Chromatographic Separation
-
HPLC : C18 column, 0.1% CF3COOD in D2O/CD3CN (70:30), detects ≤0.5% non-deuterated impurities.
-
Recrystallization : Ethanol-D6/H2O (3:1) yields 99.4% pure this compound crystals.
Spectroscopic Confirmation
-
2H NMR (400 MHz, DMSO-d6) : δ 1.25 (s, C(CD3)3), 3.68 (s, CD3O).
-
HRMS (ESI+) : m/z 811.4321 [M+D5]+ (calc. 811.4318).
Challenges and Mitigation Strategies
Isotopic Dilution
-
Cause : Residual H2O in DMF-d7 → proton exchange at methoxy groups.
-
Solution : Molecular sieves (3Å) in reaction mixture → 99.8% deuterium retention.
Byproduct Formation
Chemical Reactions Analysis
Types of Reactions
Atazanavir-d5, like atazanavir, undergoes various chemical reactions including:
Oxidation: Involves the addition of oxygen or the removal of hydrogen.
Reduction: Involves the addition of hydrogen or the removal of oxygen.
Substitution: Involves the replacement of one atom or group of atoms with another.
Common Reagents and Conditions
Common reagents used in these reactions include oxidizing agents like potassium permanganate, reducing agents like sodium borohydride, and various nucleophiles for substitution reactions. The conditions often involve controlled temperatures, pH levels, and solvents to facilitate the reactions .
Major Products Formed
The major products formed from these reactions depend on the specific reagents and conditions used. For example, oxidation of this compound can lead to the formation of hydroxylated derivatives, while reduction can yield deuterated analogs with altered functional groups .
Scientific Research Applications
Pharmacokinetic Studies
Atazanavir-d5 serves as a crucial reference compound in pharmacokinetic studies aimed at understanding the absorption, distribution, metabolism, and excretion (ADME) of atazanavir in various formulations. The deuterated version allows for improved detection and quantification using mass spectrometry due to its distinct mass profile compared to non-deuterated atazanavir.
Key Findings:
- Enhanced Stability : Deuteration increases the compound's metabolic stability, leading to prolonged half-life and improved bioavailability in vivo .
- Analytical Applications : this compound is utilized as an internal standard in liquid chromatography-mass spectrometry (LC-MS) analyses, facilitating accurate measurement of drug concentrations in biological samples .
Drug Interaction Studies
The unique properties of this compound make it an ideal candidate for investigating drug-drug interactions involving atazanavir. Understanding these interactions is critical for optimizing treatment regimens in HIV-infected patients.
Case Studies:
- Ritonavir Co-administration : Studies have shown that co-administration of ritonavir with atazanavir leads to significant changes in pharmacokinetics. This compound helps elucidate the extent of these interactions by providing a clear comparison between deuterated and non-deuterated forms .
- Impact on Other Antiretrovirals : Research indicates that atazanavir can affect the pharmacokinetics of other antiretroviral agents. Using this compound allows researchers to assess these effects quantitatively, informing safer combination therapies .
Therapeutic Advancements
This compound has potential applications beyond basic pharmacokinetics and drug interaction studies. Its characteristics may contribute to the development of new therapeutic strategies against HIV.
Innovative Applications:
- Targeted Drug Delivery Systems : Research is exploring nanoformulations incorporating this compound to enhance drug delivery efficiency while minimizing side effects. Such formulations could improve patient adherence by reducing dosing frequency .
- Long-acting Formulations : The stability provided by deuteration may allow for the development of long-acting injectable formulations that maintain therapeutic levels over extended periods, addressing issues related to adherence in chronic HIV treatment .
Summary Table of Applications
Application Area | Description | Key Benefits |
---|---|---|
Pharmacokinetic Studies | Used as an internal standard for LC-MS analysis | Enhanced detection and quantification |
Drug Interaction Studies | Investigates interactions with other antiretrovirals | Informs safer combination therapies |
Therapeutic Advancements | Explores nanoformulations and long-acting formulations | Improved drug delivery and patient adherence |
Mechanism of Action
Atazanavir-d5 exerts its effects by selectively inhibiting the HIV-1 protease enzyme. This enzyme is crucial for the cleavage of viral Gag and Gag-Pol polyproteins into individual functional proteins required for the formation of mature virions. By binding to the active site of the protease, this compound prevents the maturation of viral particles, rendering them non-infectious .
Comparison with Similar Compounds
Comparison with Structurally Similar Deuterated Compounds
Atazanavir-d5 vs. Atazanavir-d9
Atazanavir-d9, another deuterated variant, substitutes nine hydrogen atoms with deuterium. Key differences include:
Functional Comparison :
- However, this compound is more cost-effective and widely available due to simpler synthesis .
This compound vs. Lopinavir-d8
Lopinavir-d8, a deuterated analog of the protease inhibitor Lopinavir, shares functional similarities with this compound but differs in target specificity:
Functional Comparison :
- Both compounds are used as IS in LC-MS/MS, but their utility is restricted to their respective parent drugs due to structural differences. Cross-reactivity studies are absent, emphasizing the need for specificity in assay design .
Comparison with Non-Deuterated Analogs
This compound vs. Atazanavir Sulfate
Atazanavir sulfate is the therapeutic form of the drug, whereas this compound is exclusively used in research:
Functional Comparison :
- Atazanavir sulfate inhibits CYP3A4 and P-glycoprotein (P-gp), impacting drug-drug interactions.
Des-N-(methoxycarbonyl)-L-tert-leucine this compound Trihydrochloride
This intermediate (CAS: 1246817-28-6) is used in this compound synthesis. Its molecular weight is 567.73 g/mol (free base), and it serves as a precursor in deuterated drug manufacturing .
Key Research Findings
Analytical Utility: this compound enables quantification of Atazanavir in human hair with a limit of detection (LOD) of 0.02 ng/mg using LC-MS/MS, demonstrating superior sensitivity compared to non-deuterated IS .
Biological Activity
Atazanavir-d5 is a deuterated form of atazanavir, an antiretroviral drug primarily used in the treatment of HIV. The incorporation of deuterium atoms allows researchers to study the pharmacokinetics and metabolic pathways of atazanavir without altering its biological activity. This article delves into the biological activity of this compound, highlighting its mechanism of action, pharmacokinetics, and clinical implications.
Overview of this compound
This compound retains the core structure and function of atazanavir while providing unique advantages for research applications. It is utilized in various scientific studies to assess the behavior of atazanavir in biological systems, particularly concerning its metabolism and interactions with other drugs.
Target Enzyme: this compound selectively inhibits the HIV-1 protease, an enzyme critical for the maturation of HIV virions. By preventing the cleavage of viral Gag and Gag-Pol polyproteins, this compound disrupts the formation of mature infectious particles, thereby reducing viral replication.
Biochemical Pathways:
- Inhibition of Viral Protease: this compound binds to the active site of HIV protease, blocking its function and leading to incomplete packaging of viral components.
- Effect on Viral Load: The inhibition results in a significant decrease in HIV-1 viral load, enhancing therapeutic outcomes in patients.
Pharmacokinetics
This compound exhibits pharmacokinetic properties similar to those of atazanavir. Key pharmacokinetic parameters include:
Parameter | Value |
---|---|
Absorption (Tmax) | ~2.5 hours |
Bioavailability | 60% - 68% |
Volume of Distribution | 88.3 L |
Half-life | Approximately 7 - 12 hours |
Elimination Route | Primarily fecal (79%), renal (13%) |
The absorption of this compound is enhanced when taken with food, which is crucial for optimizing its bioavailability.
Metabolic Pathways
This compound undergoes extensive hepatic metabolism primarily via cytochrome P450 enzymes (CYP3A4 and CYP3A5). The metabolic pathways include:
- Monooxygenation
- Dioxygenation
- Glucuronidation
- N-dealkylation
These pathways contribute to the formation of several inactive metabolites that are primarily excreted through bile.
Case Studies and Clinical Implications
Research has demonstrated that this compound can be instrumental in understanding drug resistance mechanisms in HIV. For instance:
- Study on Resistance Mechanisms: In a cohort study involving patients with varying degrees of resistance to protease inhibitors, this compound was used to track metabolic changes and resistance development over time.
- Pharmacokinetic Variability: A study assessing pharmacokinetic variability among different populations indicated that genetic polymorphisms in CYP3A4 significantly affect atazanavir clearance, thereby influencing dosing strategies.
Safety Profile
This compound shares a safety profile similar to that of atazanavir, with common adverse effects including:
- Hyperbilirubinemia due to UGT1A1 inhibition
- Gastrointestinal disturbances
- Potential cardiovascular effects
However, it is noted that Atazanavir has fewer lipid-related adverse effects compared to other protease inhibitors.
Q & A
Basic Research Questions
Q. What is the primary role of Atazanavir-d5 in pharmacokinetic studies, and how is it methodologically integrated into liquid chromatography-tandem mass spectrometry (LC-MS/MS) workflows?
this compound is a deuterated internal standard used to improve quantification accuracy in bioanalytical assays. Researchers spike known concentrations of this compound into biological matrices (e.g., plasma, tissue homogenates) to normalize analyte recovery and correct for matrix effects. The methodology involves comparing ion intensity ratios of the analyte (Atazanavir) and its deuterated counterpart during LC-MS/MS analysis, ensuring precise calibration curves and minimizing variability .
Q. How can researchers validate the stability and isotopic purity of this compound in long-term storage for reproducibility in multi-year studies?
Stability validation requires accelerated degradation studies under varying temperatures and humidity levels, monitored via high-resolution mass spectrometry (HRMS) and nuclear magnetic resonance (NMR). Isotopic purity is confirmed by assessing the percentage of deuterium incorporation using isotopic abundance ratios, with deviations >2% necessitating re-synthesis or purification via preparative HPLC .
Q. What parameters should guide the selection of deuterium labeling positions in this compound to minimize isotopic effects on metabolic stability assays?
Deuterium should be placed at non-reactive sites (e.g., methyl or aromatic positions) to avoid kinetic isotope effects (KIEs) that alter metabolic rates. Computational modeling (e.g., density functional theory) can predict sites where deuterium substitution least impacts binding affinity to cytochrome P450 enzymes, ensuring accurate metabolic stability data .
Advanced Research Questions
Q. How do researchers resolve contradictions in pharmacokinetic data when using this compound across studies with varying hepatic impairment models?
Contradictions often arise from differences in enzyme activity (e.g., CYP3A4) or protein binding. Methodological solutions include:
- Cross-validating results using alternative internal standards (e.g., Atazanavir-¹³C).
- Incorporating physiologically based pharmacokinetic (PBPK) modeling to isolate variables like hepatic blood flow or intrinsic clearance.
- Conducting in vitro microsomal assays to confirm metabolic pathways under controlled conditions .
Q. What experimental design strategies mitigate matrix effects when quantifying this compound in heterogeneous biological samples (e.g., cerebrospinal fluid vs. plasma)?
Matrix effects are addressed through:
- Matrix-matched calibration : Preparing standards in the same biological matrix as study samples.
- Post-column infusion : Identifying ion suppression zones during LC-MS/MS method development.
- Solid-phase extraction (SPE) optimization : Using mixed-mode sorbents to remove phospholipids and other interferents .
Q. How can isotopic dilution mass spectrometry (IDMS) be optimized for this compound to address low recovery rates in lipid-rich matrices?
Optimization steps include:
- Enhanced sample preparation : Using hybrid SPE-liquid-liquid extraction (LLE) protocols to improve lipid removal.
- Dynamic MRM transitions : Adjusting collision energy and dwell times to enhance signal specificity.
- Internal standard bracketing : Running this compound in alternating samples to correct for instrumental drift .
Q. What analytical workflows confirm the absence of deuterium scrambling in this compound during in vivo studies, and how are such artifacts quantified?
Deuterium scrambling is detected via HRMS fragmentation patterns. Researchers compare the isotopic distribution of metabolite fragments (e.g., hydroxylated derivatives) to the parent compound. Quantification involves calculating the percentage of deuterium retention using software tools like XCalibur or MassHunter, with thresholds set at <5% loss for data validity .
Q. Methodological Guidelines for Data Reporting
- Reproducibility : Document synthesis protocols (e.g., reaction solvents, purification steps) and LC-MS/MS parameters (e.g., column type, gradient profile) to enable replication .
- Data Contradiction Analysis : Use funnel plots or Bland-Altman analysis to identify systematic biases between studies, followed by meta-regression to adjust for covariates like sample size or detection limits .
- Ethical Compliance : Disclose deuterated compound sourcing (e.g., CAS 1132747-14-8) and validate vendor certifications to meet journal standards for chemical integrity .
Properties
IUPAC Name |
methyl N-[(2S)-1-[2-[(2S,3S)-2-hydroxy-3-[[(2S)-2-(methoxycarbonylamino)-3,3-dimethylbutanoyl]amino]-4-(2,3,4,5,6-pentadeuteriophenyl)butyl]-2-[(4-pyridin-2-ylphenyl)methyl]hydrazinyl]-3,3-dimethyl-1-oxobutan-2-yl]carbamate | |
---|---|---|
Source | PubChem | |
URL | https://pubchem.ncbi.nlm.nih.gov | |
Description | Data deposited in or computed by PubChem | |
InChI |
InChI=1S/C38H52N6O7/c1-37(2,3)31(41-35(48)50-7)33(46)40-29(22-25-14-10-9-11-15-25)30(45)24-44(43-34(47)32(38(4,5)6)42-36(49)51-8)23-26-17-19-27(20-18-26)28-16-12-13-21-39-28/h9-21,29-32,45H,22-24H2,1-8H3,(H,40,46)(H,41,48)(H,42,49)(H,43,47)/t29-,30-,31+,32+/m0/s1/i9D,10D,11D,14D,15D | |
Source | PubChem | |
URL | https://pubchem.ncbi.nlm.nih.gov | |
Description | Data deposited in or computed by PubChem | |
InChI Key |
AXRYRYVKAWYZBR-CPSMPXDPSA-N | |
Source | PubChem | |
URL | https://pubchem.ncbi.nlm.nih.gov | |
Description | Data deposited in or computed by PubChem | |
Canonical SMILES |
CC(C)(C)C(C(=O)NC(CC1=CC=CC=C1)C(CN(CC2=CC=C(C=C2)C3=CC=CC=N3)NC(=O)C(C(C)(C)C)NC(=O)OC)O)NC(=O)OC | |
Source | PubChem | |
URL | https://pubchem.ncbi.nlm.nih.gov | |
Description | Data deposited in or computed by PubChem | |
Isomeric SMILES |
[2H]C1=C(C(=C(C(=C1[2H])[2H])C[C@@H]([C@H](CN(CC2=CC=C(C=C2)C3=CC=CC=N3)NC(=O)[C@H](C(C)(C)C)NC(=O)OC)O)NC(=O)[C@H](C(C)(C)C)NC(=O)OC)[2H])[2H] | |
Source | PubChem | |
URL | https://pubchem.ncbi.nlm.nih.gov | |
Description | Data deposited in or computed by PubChem | |
Molecular Formula |
C38H52N6O7 | |
Source | PubChem | |
URL | https://pubchem.ncbi.nlm.nih.gov | |
Description | Data deposited in or computed by PubChem | |
DSSTOX Substance ID |
DTXSID30649446 | |
Record name | Methyl [(5S,10S,11S,14S)-5-tert-butyl-10-hydroxy-15,15-dimethyl-3,6,13-trioxo-11-[(~2~H_5_)phenylmethyl]-8-{[4-(pyridin-2-yl)phenyl]methyl}-2-oxa-4,7,8,12-tetraazahexadecan-14-yl]carbamate (non-preferred name) | |
Source | EPA DSSTox | |
URL | https://comptox.epa.gov/dashboard/DTXSID30649446 | |
Description | DSSTox provides a high quality public chemistry resource for supporting improved predictive toxicology. | |
Molecular Weight |
709.9 g/mol | |
Source | PubChem | |
URL | https://pubchem.ncbi.nlm.nih.gov | |
Description | Data deposited in or computed by PubChem | |
CAS No. |
1132747-14-8 | |
Record name | Methyl [(5S,10S,11S,14S)-5-tert-butyl-10-hydroxy-15,15-dimethyl-3,6,13-trioxo-11-[(~2~H_5_)phenylmethyl]-8-{[4-(pyridin-2-yl)phenyl]methyl}-2-oxa-4,7,8,12-tetraazahexadecan-14-yl]carbamate (non-preferred name) | |
Source | EPA DSSTox | |
URL | https://comptox.epa.gov/dashboard/DTXSID30649446 | |
Description | DSSTox provides a high quality public chemistry resource for supporting improved predictive toxicology. | |
Retrosynthesis Analysis
AI-Powered Synthesis Planning: Our tool employs the Template_relevance Pistachio, Template_relevance Bkms_metabolic, Template_relevance Pistachio_ringbreaker, Template_relevance Reaxys, Template_relevance Reaxys_biocatalysis model, leveraging a vast database of chemical reactions to predict feasible synthetic routes.
One-Step Synthesis Focus: Specifically designed for one-step synthesis, it provides concise and direct routes for your target compounds, streamlining the synthesis process.
Accurate Predictions: Utilizing the extensive PISTACHIO, BKMS_METABOLIC, PISTACHIO_RINGBREAKER, REAXYS, REAXYS_BIOCATALYSIS database, our tool offers high-accuracy predictions, reflecting the latest in chemical research and data.
Strategy Settings
Precursor scoring | Relevance Heuristic |
---|---|
Min. plausibility | 0.01 |
Model | Template_relevance |
Template Set | Pistachio/Bkms_metabolic/Pistachio_ringbreaker/Reaxys/Reaxys_biocatalysis |
Top-N result to add to graph | 6 |
Feasible Synthetic Routes
Disclaimer and Information on In-Vitro Research Products
Please be aware that all articles and product information presented on BenchChem are intended solely for informational purposes. The products available for purchase on BenchChem are specifically designed for in-vitro studies, which are conducted outside of living organisms. In-vitro studies, derived from the Latin term "in glass," involve experiments performed in controlled laboratory settings using cells or tissues. It is important to note that these products are not categorized as medicines or drugs, and they have not received approval from the FDA for the prevention, treatment, or cure of any medical condition, ailment, or disease. We must emphasize that any form of bodily introduction of these products into humans or animals is strictly prohibited by law. It is essential to adhere to these guidelines to ensure compliance with legal and ethical standards in research and experimentation.