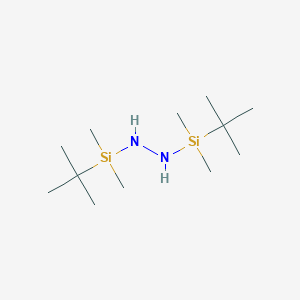
1,2-Bis-(tert-butyldimethylsilyl)hydrazine
Overview
Description
Synthesis Analysis
The synthesis of 1,2-Bis-(tert-butyldimethylsilyl)hydrazine involves the reaction of di-tert-butyldifluorosilane with monolithiated hydrazine, yielding the desired silylated hydrazine derivatives. This process is highlighted by the formation of structure-isomeric N,N- and N,N′-Bis(silyl)hydrazines through various condensation reactions and HCl elimination processes (Drost & Klingebiel, 1993).
Molecular Structure Analysis
The molecular structure of 1,2-Bis-(tert-butyldimethylsilyl)hydrazine exhibits unique features due to the presence of tert-butyldimethylsilyl groups attached to the nitrogen atoms of the hydrazine. These structural elements significantly influence the compound's reactivity and stability, making it a valuable reagent in organic synthesis.
Chemical Reactions and Properties
1,2-Bis-(tert-butyldimethylsilyl)hydrazine participates in various chemical reactions, including Wolff-Kishner reductions, preparation of vinyl halides, and synthesis of gem-dihalides. Its use in Wolff-Kishner-type reduction reactions demonstrates its utility as a superior alternative to simple hydrazones (Furrow & Myers, 2004).
Scientific Research Applications
Building Blocks for Chemical Synthesis : (Di-tert1-butylfluorsilyl)hydrazine serves as a building block for isomeric N,N- and N,N′1-bis(silyl)hydrazines, important in forming stable monosilylated hydrazines (Drost & Klingebiel, 1993).
Antineoplastic Agents : 1-acetyl-1,2-bis-(methylsulfonyl)-2-(2-chloroethyl)hydrazine exhibits promising antineoplastic activity against various cancers, indicating potential for improved drug formulations (Shyam et al., 1993).
Polymer Stabilization : Crystal polymorphism in 1,2-bis(3,5-di-tert-butyl-4-hydroxyhydrocinnamoyl)hydrazine, a polymer stabilizer, affects additive behavior and dispersion in polymer compounds (Cogen & Hilmer, 2008).
Chemical Reactions and Compounds : Dilithium bis(trimethylsilyl)hydrazine reacts with group IV halides to form various chemical compounds (Vasisht et al., 1986). Moreover, N-tert-butyldimethylsilylhydrazones (TBSH) are efficient in Wolff-Kishner-type reduction reactions and synthesizing vinyl halides and gem-dihalides (Furrow & Myers, 2004).
Stable Compound Formation : 1,1-bis(trifluoromethyl)hydrazine is a stable compound used to produce perfluorohydrazones (Bykhovskaya et al., 1984).
Cyclization and Crystal Structures : The formation of silylhydrazines from 1,2-bis(bromosilyl)ethane and 1,3-bis(bromosilyl)propane with hydrazine leads to bicyclic compounds with unique cyclization modes and crystal structures (Mitzel et al., 1993).
Fluorescent Compounds : The bis(BF2) core complex containing a 1,8-naphthyridine derivative shows intense fluorescence, useful in photophysical studies (Li et al., 2010).
Synthesizing Novel Compounds : Lithium-bis(silyl)hydrazide, Tris(silyl)hydrazine, and 1,2-diaza-3,5-disilacyclopentane are synthesized by reacting bis(silyl)hydrazines with halogensilanes (Bode et al., 1994).
Future Directions
1,2-Bis-(tert-butyldimethylsilyl)hydrazine finds utility in synthesizing metal-organic frameworks, preparing functionalized nanomaterials, and contributing to polymer synthesis . Given its versatile applications and broad synthetic capabilities, this compound holds significant importance in the field of organic chemistry .
properties
IUPAC Name |
1,2-bis[tert-butyl(dimethyl)silyl]hydrazine | |
---|---|---|
Source | PubChem | |
URL | https://pubchem.ncbi.nlm.nih.gov | |
Description | Data deposited in or computed by PubChem | |
InChI |
InChI=1S/C12H32N2Si2/c1-11(2,3)15(7,8)13-14-16(9,10)12(4,5)6/h13-14H,1-10H3 | |
Source | PubChem | |
URL | https://pubchem.ncbi.nlm.nih.gov | |
Description | Data deposited in or computed by PubChem | |
InChI Key |
AIZSLKRCSRDHKC-UHFFFAOYSA-N | |
Source | PubChem | |
URL | https://pubchem.ncbi.nlm.nih.gov | |
Description | Data deposited in or computed by PubChem | |
Canonical SMILES |
CC(C)(C)[Si](C)(C)NN[Si](C)(C)C(C)(C)C | |
Source | PubChem | |
URL | https://pubchem.ncbi.nlm.nih.gov | |
Description | Data deposited in or computed by PubChem | |
Molecular Formula |
C12H32N2Si2 | |
Source | PubChem | |
URL | https://pubchem.ncbi.nlm.nih.gov | |
Description | Data deposited in or computed by PubChem | |
DSSTOX Substance ID |
DTXSID20471430 | |
Record name | 1,2-Bis-(tert-butyldimethylsilyl)hydrazine | |
Source | EPA DSSTox | |
URL | https://comptox.epa.gov/dashboard/DTXSID20471430 | |
Description | DSSTox provides a high quality public chemistry resource for supporting improved predictive toxicology. | |
Molecular Weight |
260.57 g/mol | |
Source | PubChem | |
URL | https://pubchem.ncbi.nlm.nih.gov | |
Description | Data deposited in or computed by PubChem | |
Product Name |
1,2-Bis-(tert-butyldimethylsilyl)hydrazine | |
CAS RN |
10000-20-1 | |
Record name | 1,2-Bis-(tert-butyldimethylsilyl)hydrazine | |
Source | EPA DSSTox | |
URL | https://comptox.epa.gov/dashboard/DTXSID20471430 | |
Description | DSSTox provides a high quality public chemistry resource for supporting improved predictive toxicology. | |
Retrosynthesis Analysis
AI-Powered Synthesis Planning: Our tool employs the Template_relevance Pistachio, Template_relevance Bkms_metabolic, Template_relevance Pistachio_ringbreaker, Template_relevance Reaxys, Template_relevance Reaxys_biocatalysis model, leveraging a vast database of chemical reactions to predict feasible synthetic routes.
One-Step Synthesis Focus: Specifically designed for one-step synthesis, it provides concise and direct routes for your target compounds, streamlining the synthesis process.
Accurate Predictions: Utilizing the extensive PISTACHIO, BKMS_METABOLIC, PISTACHIO_RINGBREAKER, REAXYS, REAXYS_BIOCATALYSIS database, our tool offers high-accuracy predictions, reflecting the latest in chemical research and data.
Strategy Settings
Precursor scoring | Relevance Heuristic |
---|---|
Min. plausibility | 0.01 |
Model | Template_relevance |
Template Set | Pistachio/Bkms_metabolic/Pistachio_ringbreaker/Reaxys/Reaxys_biocatalysis |
Top-N result to add to graph | 6 |
Feasible Synthetic Routes
Citations
Disclaimer and Information on In-Vitro Research Products
Please be aware that all articles and product information presented on BenchChem are intended solely for informational purposes. The products available for purchase on BenchChem are specifically designed for in-vitro studies, which are conducted outside of living organisms. In-vitro studies, derived from the Latin term "in glass," involve experiments performed in controlled laboratory settings using cells or tissues. It is important to note that these products are not categorized as medicines or drugs, and they have not received approval from the FDA for the prevention, treatment, or cure of any medical condition, ailment, or disease. We must emphasize that any form of bodily introduction of these products into humans or animals is strictly prohibited by law. It is essential to adhere to these guidelines to ensure compliance with legal and ethical standards in research and experimentation.