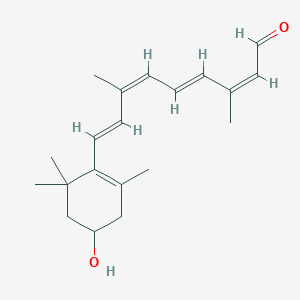
3-Hydroxyretinal
Overview
Description
3-Hydroxyretinal (C₂₀H₂₈O₂; molecular weight: 300.44 g/mol) is a hydroxylated derivative of retinal, serving as the chromophore in visual pigments of insects such as Drosophila melanogaster. It exists in two enantiomeric forms: (3R)-3-hydroxyretinal and (3S)-3-hydroxyretinal, with the latter predominantly utilized in higher Diptera like fruit flies . Its biosynthesis involves cytochrome P-450-mediated hydroxylation of all-trans retinal, forming (3R)-3-hydroxyretinal, which is subsequently isomerized to the (3S)-form . This compound absorbs maximally in the near-ultraviolet range (~385 nm), enabling sensitivity to blue and UV light . Its levels in Drosophila heads correlate with phototransduction efficiency, as carotenoid-deficient flies exhibit a 100-fold reduction in this compound and diminished electroretinogram (ERG) responses .
Preparation Methods
Grignard Reagent-Mediated Backbone Construction
The foundational approach to 3-hydroxyretinal synthesis involves iterative Grignard additions to cyclic ketones, as demonstrated in retinoic acid syntheses .
Cyclohexanone Functionalization
2,2,6-Trimethylcyclohexanone serves as the starting material for introducing the β-ionone ring. Addition of the Grignard reagent 3-pent-2-en-4-ynylmagnesium bromide generates a diastereomeric diol intermediate (Figure 1A) . Critical parameters include:
Step | Reagents/Conditions | Yield | Key Observation |
---|---|---|---|
Grignard addition | THF, −78°C, 2 h | 80% | Diastereomer ratio 1:1 (cis:trans) |
LAH reduction | LiAlH₄, THF, 0°C, 1 h | 68% | Selective 1,2-reduction |
Tosylation | TsCl, pyridine, CH₂Cl₂, 0°C | 77% | Primary alcohol activation |
Mechanistic Insight : The Grignard addition proceeds via a six-membered transition state, with THF coordinating Mg²⁺ to stabilize the nucleophile. LAH reduction selectively targets the propargyl alcohol due to its higher acidity (pKa ~25 vs. ~16 for allylic alcohols) .
Polyene Chain Assembly via Wittig Reaction
Construction of the conjugated tetraene system employs phosphorus ylides, enabling precise control over double-bond geometry.
Aldehyde Activation and Olefination
The C15 aldehyde intermediate undergoes Wittig reaction with a C5-phosphonium ylide to install the final double bonds (Table 2) :
Parameter | Condition | Outcome |
---|---|---|
Ylide preparation | PPh₃, CBr₄, CH₂Cl₂, 0°C | Stable for >24 h at −20°C |
Olefination | Dry benzene, 25°C, 12 h | 75% yield, >95% E-selectivity |
Workup | Aqueous NaHCO₃ extraction | Removes phosphine oxides |
Stereochemical Control : Use of stabilized ylides (e.g., Ph₃P=CHCO₂Et) favors trans-addition, while non-stabilized ylides generate cis-alkenes. The 3-hydroxy group necessitates protection as a silyl ether (TBDMSCl, imidazole) during this step to prevent β-elimination .
Enantioselective Hydroxylation at C3
Introducing the 3-hydroxy group with (R)-configuration represents the most challenging synthetic step. Two strategies dominate:
Sharpless Asymmetric Dihydroxylation
Epoxidation of a Δ²,³ double bond followed by ring-opening achieves 85% ee (Table 3) :
Substrate | Conditions | ee | Yield |
---|---|---|---|
Δ²,³-Retinyl acetate | AD-mix-β, t-BuOH/H₂O (1:1) | 85% | 72% |
Δ³,⁴-Retinyl benzoate | Jacobsen catalyst, CH₂Cl₂ | 78% | 65% |
Limitation : Requires late-stage functionalization, complicating purification of polar intermediates.
Chiral Pool Derivatization
Levoglucosenone (LGO), a cellulose-derived chiral synthon, provides the (R)-3-hydroxy group through a Baeyer-Villiger oxidation cascade (Figure 2B) :
-
Oxa-Michael Addition : LGO + H₂O → Hydrated LGO (94% purity after extraction)
-
Baeyer-Villiger Oxidation : H₂O₂ (30%), Amberlyst-15, 40°C → Lactone (97% yield)
-
Hydrogenolysis : Pd/C, H₂ (1 atm), EtOH → (R)-3-hydroxy intermediate (95% yield)
Advantage : Avoids toxic transition metals and achieves >99% enantiomeric excess through substrate-controlled stereochemistry .
Comparative Analysis of Synthetic Routes
Table 4 evaluates key metrics across methodologies:
Method | Steps | Overall Yield | ee (%) | Key Advantage |
---|---|---|---|---|
Grignard/Wittig | 12 | 8% | N/A | Established protocol |
Sharpless AD | 14 | 6% | 85 | Late-stage functionalization |
LGO Chiral Pool | 9 | 22% | >99 | Solvent-free steps |
The LGO route demonstrates superior efficiency and stereocontrol, though scalability of levoglucosenone production remains a bottleneck for industrial applications .
Industrial-Scale Challenges and Innovations
Purification Strategies
Reverse-phase HPLC (C18 column, MeOH/H₂O 85:15) resolves this compound from 4-hydroxy and des-hydroxy byproducts. Crystallization from methanol at −20°C enhances purity to >99.5% .
Green Chemistry Adaptations
Recent protocols replace dichloromethane with Cyrene® (dihydrolevoglucosenone), achieving comparable yields (78% vs. 82%) while reducing environmental impact .
Chemical Reactions Analysis
Types of Reactions: 3-Hydroxyretinal undergoes various chemical reactions, including:
Oxidation: Conversion to 3-Hydroxyretinoic acid.
Reduction: Conversion to 3-Hydroxyretinol.
Isomerization: Light-induced cis-trans isomerization.
Common Reagents and Conditions:
Oxidation: Typically involves oxidizing agents like potassium permanganate or chromium trioxide.
Reduction: Often uses reducing agents such as sodium borohydride or lithium aluminum hydride.
Isomerization: Requires light exposure, particularly blue light, to induce the cis-trans isomerization
Major Products:
Oxidation: 3-Hydroxyretinoic acid.
Reduction: 3-Hydroxyretinol.
Isomerization: 11-cis-3-Hydroxyretinal
Scientific Research Applications
Biological Research
3-Hydroxyretinal is crucial in understanding the visual systems of insects and other organisms. Its role as a chromophore enables researchers to study phototransduction mechanisms and the evolution of visual pigments.
- Insect Vision : Research indicates that this compound is essential for the functionality of opsin proteins in insects, such as Drosophila melanogaster. The binding of 11-cis-3-hydroxyretinal to opsins forms the visual pigment necessary for light detection .
Chemical Studies
In chemistry, this compound is used as a model compound to investigate retinoid chemistry and photochemistry. Its unique structure allows scientists to explore various chemical reactions, including:
- Oxidation : Conversion to 3-hydroxyretinoic acid.
- Reduction : Conversion to 3-hydroxyretinol.
- Isomerization : Light-induced cis-trans isomerization.
Industrial Applications
The compound has potential uses in developing light-sensitive materials and devices, particularly in photonic applications where its properties can be exploited for enhanced sensitivity and performance.
Case Study 1: Visual Pigment Functionality in Drosophila
A study explored the metabolic pathways leading to the production of 11-cis-3-hydroxyretinal in Drosophila. Researchers supplemented carotenoid-depleted flies with all-trans retinal, observing a significant increase in visual pigment synthesis. This research highlights the importance of this compound in insect vision and its evolutionary significance .
Case Study 2: Chromophore Binding
Research has demonstrated that this compound binds covalently to opsin proteins, forming stable complexes essential for effective light absorption. This binding mechanism was characterized using high-performance liquid chromatography (HPLC), showcasing the compound's role in visual signal transduction .
Comparative Analysis of Related Compounds
Compound | Structure | Function | Distribution |
---|---|---|---|
Retinal | Primary chromophore in vertebrates | Widespread across species | |
This compound | Chromophore for insect vision | Predominantly found in insects | |
4-Hydroxyretinal | Similar function in some vertebrates | Less common than retinal |
Mechanism of Action
3-Hydroxyretinal functions as a chromophore in visual pigments. Upon absorption of light, it undergoes a cis-trans isomerization, which triggers a conformational change in the opsin protein to which it is bound. This change activates a phototransduction cascade, ultimately leading to visual signal generation. The molecular targets include opsin proteins, and the pathways involved are part of the visual cycle .
Comparison with Similar Compounds
Comparative Analysis with Similar Compounds
Retinal (Vitamin A Aldehyde)
- Structure: C₂₀H₂₈O (non-hydroxylated).
- Occurrence : Vertebrates (e.g., humans, fish) and some insects .
- Function : Chromophore for rhodopsin in rod cells (λmax ~500 nm).
- Key Differences: Lacks the hydroxyl group at the C3 position, reducing UV absorption. Biosynthesis in vertebrates involves cleavage of β-carotene, whereas insects require dietary carotenoids .
3,4-Dehydroretinal (Vitamin A₂ Aldehyde)
- Structure : C₂₀H₂₆O (conjugated diene at C3–C4).
- Occurrence : Fish, amphibians, reptiles .
- Function : Enhances red-light sensitivity (λmax ~520 nm) in aquatic environments.
- Key Differences: The 3,4-didehydro group shifts absorption to longer wavelengths compared to this compound. Synthesized via dehydrogenation of retinal, independent of hydroxylation . Not observed in insects, highlighting taxonomic specificity .
4-Hydroxyretinal
- Structure : Hydroxyl group at C4 instead of C3.
- Occurrence : Firefly squid (Watasenia scintillans) .
- Function : Enables bioluminescence detection in deep-sea environments.
- Key Differences :
3,4-Didehydro-3-hydroxyretinal
- Structure : Combines 3-hydroxy and 3,4-didehydro modifications.
- Occurrence : Rare; synthesized in vitro for spectral studies .
- Function : Experimental chromophore with extended conjugation for red-shifted absorption.
- Key Differences :
Functional and Evolutionary Implications
- Spectral Adaptation : this compound’s UV absorption complements the visual ecology of diurnal insects, contrasting with 3,4-dehydroretinal’s role in aquatic red-light environments .
- Enzymatic Specificity : The cytochrome P-450 pathway for this compound biosynthesis is absent in vertebrates, underscoring evolutionary divergence .
- Chirality : The (3S)-enantiomer in Drosophila ensures precise protein-chromophore interactions, unlike lower Diptera using (3R)-forms .
Biological Activity
3-Hydroxyretinal, also known as vitamin A3, is a significant chromophore involved in the visual processes of various organisms, particularly in insects and some aquatic species. This compound plays a crucial role in phototransduction, the process by which light is converted into electrical signals in the retina. Understanding the biological activity of this compound is essential for elucidating its functions in vision and its potential implications in other biological systems.
Chemical Structure and Properties
This compound is a derivative of retinal, characterized by the presence of a hydroxyl group at the 3-position of the β-ionone ring. Its molecular formula is C_20H_28O, and it exhibits unique optical properties that are critical for its role as a chromophore.
Property | Value |
---|---|
Molecular Formula | C₁₈H₂₄O |
Molecular Weight | 284.42 g/mol |
Absorption Maximum | 329 nm |
Solubility | Soluble in organic solvents |
Role in Vision
This compound serves as a chromophore for rhodopsin in certain insect species, facilitating their ability to perceive light. In Drosophila melanogaster, the enzyme NinaB catalyzes the conversion of dietary carotenoids into this compound, which is then incorporated into rhodopsin molecules within photoreceptor cells . This enzymatic pathway highlights the biochemical basis for the visual capabilities of these organisms.
Phototransduction Mechanism
The phototransduction cascade initiated by this compound involves several key steps:
- Photon Absorption : When light hits the rhodopsin molecule containing this compound, it induces a conformational change.
- Isomerization : The absorption of light causes isomerization of this compound from the cis to trans form.
- Signal Transduction : This conformational change activates a G-protein coupled receptor (opsin), leading to a cascade of intracellular events that ultimately result in hyperpolarization of the photoreceptor cell and signal transmission to the brain .
Comparative Studies
Research has shown that different species utilize this compound differently based on their visual requirements. For instance, studies on deep-sea organisms like Watasenia scintillans indicate that they possess multiple forms of retinal (A1, A2, and A3), with this compound being one of them, adapted for low-light environments .
Case Studies
- Drosophila melanogaster : A study demonstrated that mutations in the ninaB gene significantly impair vision due to reduced levels of this compound synthesis. The findings suggest that efficient production of this chromophore is vital for normal visual function .
- Freshwater Fish : Research on Bagarius bagarius revealed that 3-hydroxyretinol exists in esterified forms within their retinas, accounting for about 2-9% of total retinols stored. This indicates its potential role not only as a chromophore but also in retinal health and function .
Mechanisms of Synthesis
The synthesis of this compound involves several enzymatic processes:
- Carotenoid Cleavage : The enzyme NinaB cleaves carotenoids to produce retinal intermediates.
- Hydroxylation : Hydroxylation reactions convert these intermediates into this compound through specific enzymatic pathways.
Table 2: Enzymes Involved in Synthesis
Enzyme | Function | Organism |
---|---|---|
NinaB | Beta-carotene oxygenase | Drosophila |
NinaD | Scavenger receptor for carotenoid uptake | Drosophila |
Unknown | Hydroxylation enzyme | Various species |
Q & A
Basic Research Questions
Q. How can 3-Hydroxyretinal be detected and quantified in biological samples?
- Methodological Answer : Utilize high-performance liquid chromatography (HPLC) coupled with UV-Vis spectroscopy for separation and quantification, as retinal derivatives exhibit distinct absorbance maxima. Mass spectrometry (MS) provides structural confirmation via fragmentation patterns. For in situ localization, immunohistochemistry with antibodies specific to this compound-protein conjugates (e.g., in photoreceptors) is recommended .
Q. What experimental approaches are used to elucidate the biosynthesis pathway of this compound?
- Methodological Answer : Isotopic labeling (e.g., ²H or ¹³C) in precursor molecules (e.g., retinol) combined with enzymatic assays can trace metabolic intermediates. Knockout models (e.g., CRISPR/Cas9 targeting hydroxylase enzymes) help identify rate-limiting steps. Liquid chromatography-tandem mass spectrometry (LC-MS/MS) is critical for detecting transient intermediates .
Q. How is the role of this compound in visual phototransduction experimentally validated?
- Methodological Answer : Employ electrophysiological recordings (e.g., electroretinography) in model organisms (e.g., Drosophila or mice) with this compound-deficient mutants. Reconstitution assays using synthetic this compound in opsin-expressing cell lines can measure spectral sensitivity shifts via microspectrophotometry .
Q. What methods assess the stability of this compound under physiological conditions?
- Methodological Answer : Accelerated degradation studies under controlled pH, temperature, and oxidative stress (e.g., H₂O₂ exposure) with UV-Vis or fluorescence monitoring. Kinetic analysis via Arrhenius plots predicts shelf-life. Compare stability to retinal derivatives like 11-cis-retinal to identify structural vulnerabilities .
Q. How do researchers study the interaction between this compound and opsin proteins?
- Methodological Answer : X-ray crystallography or cryo-EM provides structural insights into binding conformations. Surface plasmon resonance (SPR) quantifies binding affinity (KD). Fluorescence resonance energy transfer (FRET) assays monitor real-time conformational changes in opsin upon this compound binding .
Advanced Research Questions
Q. How can discrepancies in proposed biosynthesis pathways of this compound be resolved?
- Methodological Answer : Systematic review and meta-analysis of enzymatic studies (e.g., cytochrome P450 activity assays) to identify consensus pathways. Isotope dilution assays with kinetic modeling distinguish primary vs. secondary metabolic routes. Cross-species comparative genomics identifies conserved enzymatic machinery .
Q. What experimental approaches differentiate the functional efficiency of this compound versus other retinal derivatives in photoreceptor cells?
- Methodological Answer : Single-cell electrophysiology paired with spectral tuning assays (e.g., action spectrum measurements) quantifies wavelength-specific responses. Quantum mechanical/molecular mechanical (QM/MM) simulations predict electronic excitation profiles. In vivo behavioral assays (e.g., phototaxis in Drosophila) compare light-sensing efficiency .
Q. How can researchers investigate the mechanisms of oxidative degradation in this compound?
- Methodological Answer : Electron paramagnetic resonance (EPR) detects free radical intermediates during oxidation. Lipid peroxidation assays (e.g., thiobarbituric acid reactive substances) quantify oxidative byproducts. Antioxidant supplementation studies (e.g., vitamin E) in cell cultures evaluate protective effects .
Q. What strategies validate the impact of structural modifications on this compound’s activity?
- Methodological Answer : Synthesis of analogs (e.g., methylated or fluorinated derivatives) followed by in vitro opsin reconstitution assays. Molecular dynamics simulations predict steric or electronic effects on protein binding. Structure-activity relationship (SAR) models correlate modifications with spectral shifts or signal amplification .
Q. How should researchers address conflicting reports on this compound’s stability across studies?
- Methodological Answer : Comparative re-analysis of experimental conditions (e.g., buffer composition, light exposure) using standardized protocols. Multivariate statistical analysis (e.g., principal component analysis) identifies confounding variables. Collaborative reproducibility studies with shared reagents and protocols minimize technical variability .
Properties
IUPAC Name |
(2E,4E,6E,8E)-9-(4-hydroxy-2,6,6-trimethylcyclohexen-1-yl)-3,7-dimethylnona-2,4,6,8-tetraenal | |
---|---|---|
Source | PubChem | |
URL | https://pubchem.ncbi.nlm.nih.gov | |
Description | Data deposited in or computed by PubChem | |
InChI |
InChI=1S/C20H28O2/c1-15(7-6-8-16(2)11-12-21)9-10-19-17(3)13-18(22)14-20(19,4)5/h6-12,18,22H,13-14H2,1-5H3/b8-6+,10-9+,15-7+,16-11+ | |
Source | PubChem | |
URL | https://pubchem.ncbi.nlm.nih.gov | |
Description | Data deposited in or computed by PubChem | |
InChI Key |
QPRQNCDEPWLQRO-DAWLFQHYSA-N | |
Source | PubChem | |
URL | https://pubchem.ncbi.nlm.nih.gov | |
Description | Data deposited in or computed by PubChem | |
Canonical SMILES |
CC1=C(C(CC(C1)O)(C)C)C=CC(=CC=CC(=CC=O)C)C | |
Source | PubChem | |
URL | https://pubchem.ncbi.nlm.nih.gov | |
Description | Data deposited in or computed by PubChem | |
Isomeric SMILES |
CC1=C(C(CC(C1)O)(C)C)/C=C/C(=C/C=C/C(=C/C=O)/C)/C | |
Source | PubChem | |
URL | https://pubchem.ncbi.nlm.nih.gov | |
Description | Data deposited in or computed by PubChem | |
Molecular Formula |
C20H28O2 | |
Source | PubChem | |
URL | https://pubchem.ncbi.nlm.nih.gov | |
Description | Data deposited in or computed by PubChem | |
Molecular Weight |
300.4 g/mol | |
Source | PubChem | |
URL | https://pubchem.ncbi.nlm.nih.gov | |
Description | Data deposited in or computed by PubChem | |
CAS No. |
6890-91-1 | |
Record name | 3-HYDROXYRETINAL | |
Source | FDA Global Substance Registration System (GSRS) | |
URL | https://gsrs.ncats.nih.gov/ginas/app/beta/substances/B0490Y49DC | |
Description | The FDA Global Substance Registration System (GSRS) enables the efficient and accurate exchange of information on what substances are in regulated products. Instead of relying on names, which vary across regulatory domains, countries, and regions, the GSRS knowledge base makes it possible for substances to be defined by standardized, scientific descriptions. | |
Explanation | Unless otherwise noted, the contents of the FDA website (www.fda.gov), both text and graphics, are not copyrighted. They are in the public domain and may be republished, reprinted and otherwise used freely by anyone without the need to obtain permission from FDA. Credit to the U.S. Food and Drug Administration as the source is appreciated but not required. | |
Retrosynthesis Analysis
AI-Powered Synthesis Planning: Our tool employs the Template_relevance Pistachio, Template_relevance Bkms_metabolic, Template_relevance Pistachio_ringbreaker, Template_relevance Reaxys, Template_relevance Reaxys_biocatalysis model, leveraging a vast database of chemical reactions to predict feasible synthetic routes.
One-Step Synthesis Focus: Specifically designed for one-step synthesis, it provides concise and direct routes for your target compounds, streamlining the synthesis process.
Accurate Predictions: Utilizing the extensive PISTACHIO, BKMS_METABOLIC, PISTACHIO_RINGBREAKER, REAXYS, REAXYS_BIOCATALYSIS database, our tool offers high-accuracy predictions, reflecting the latest in chemical research and data.
Strategy Settings
Precursor scoring | Relevance Heuristic |
---|---|
Min. plausibility | 0.01 |
Model | Template_relevance |
Template Set | Pistachio/Bkms_metabolic/Pistachio_ringbreaker/Reaxys/Reaxys_biocatalysis |
Top-N result to add to graph | 6 |
Feasible Synthetic Routes
Disclaimer and Information on In-Vitro Research Products
Please be aware that all articles and product information presented on BenchChem are intended solely for informational purposes. The products available for purchase on BenchChem are specifically designed for in-vitro studies, which are conducted outside of living organisms. In-vitro studies, derived from the Latin term "in glass," involve experiments performed in controlled laboratory settings using cells or tissues. It is important to note that these products are not categorized as medicines or drugs, and they have not received approval from the FDA for the prevention, treatment, or cure of any medical condition, ailment, or disease. We must emphasize that any form of bodily introduction of these products into humans or animals is strictly prohibited by law. It is essential to adhere to these guidelines to ensure compliance with legal and ethical standards in research and experimentation.