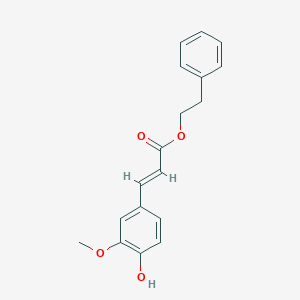
Phenylethyl 3-methylcaffeate
Overview
Description
Synthesis Analysis
The synthesis of phenylethyl 3-methylcaffeate and related compounds often involves complex organic reactions, including esterification, alkylation, and coupling reactions. For instance, the synthesis of related phenylene-containing oligoacenes using 3,4-bis(methylene)cyclobutene as a building block for sequential Diels-Alder reactions has been described, demonstrating the intricate steps involved in synthesizing complex organic molecules with phenyl groups (Parkhurst & Swager, 2012).
Molecular Structure Analysis
The molecular structure of phenylethyl 3-methylcaffeate is characterized by a phenylethyl group linked to a methylcaffeate structure. The arrangement of these groups and their electronic interactions can significantly impact the compound's reactivity and properties. Studies on similar compounds, like the synthesis and optical properties of phenylene-containing oligoacenes, provide insights into how phenyl groups and their arrangements can influence a molecule's optical and electrochemical properties (Parkhurst & Swager, 2012).
Chemical Reactions and Properties
Phenylethyl 3-methylcaffeate may undergo various chemical reactions, including esterification, oxidation, and coupling reactions. The presence of the ester group in 3-methylcaffeate provides reactive sites for chemical modifications. For example, the methoxycarbonylation of phenylethyne catalyzed by palladium complexes highlights the reactivity of phenyl groups in ester formation and cascade reactions (Núñez Magro et al., 2010).
Physical Properties Analysis
The physical properties of phenylethyl 3-methylcaffeate, such as melting point, solubility, and crystallinity, are influenced by its molecular structure. The interaction between the phenylethyl group and the methylcaffeate moiety can affect the compound's physical state and behavior in different solvents. Research on similar compounds, such as the hydrothermal synthesis and properties of new multicarboxylate ligands-based compounds, can provide insights into how molecular structures impact physical properties (Pan et al., 2008).
Chemical Properties Analysis
The chemical properties of phenylethyl 3-methylcaffeate, including reactivity, stability, and functional group behavior, are central to its potential applications. The compound's ester group and phenylethyl moiety contribute to its chemical behavior in various reactions and interactions with other molecules. For example, the synthesis of esters of acetyloxycaffeic acids and their occurrence in poplar bud exudates highlight the chemical versatility of caffeate esters and their potential for forming diverse ester derivatives (Greenaway & Whatley, 1991).
Scientific Research Applications
Application Summary
PEMC has been studied for its chemopreventive action on azoxymethane-induced colon carcinogenesis . It’s found to be a potent inhibitor of human colon adenocarcinoma cell growth, carcinogen-induced biochemical changes, and preneoplastic lesions in the rat colon .
Methods of Application
In the study, groups of rats were fed a diet containing 750 ppm of PEMC. At 7 weeks of age, all animals except those in the vehicle-treated groups were given 2 weekly subcutaneous injections of azoxymethane at a dose rate of 15 mg/kg body weight/week .
Results or Outcomes
Dietary administration of PEMC significantly inhibited the incidence and multiplicity of invasive, noninvasive, and total adenocarcinomas of the colon . It also suppressed the colon tumor volume by 43% compared to the control diet . Animals fed the PEMC diet showed significantly decreased activities of colonic mucosal and tumor phosphatidylinositol-specific phospholipase C (about 50%), but PEMC diet had no effect on phospholipase A2 . The production of hydroxyeicosatetraenoic acids via the lipoxygenase pathway from arachidonic acid was reduced in colonic mucosa and tumors (30–60%) of animals fed the PEMC diet as compared to control diet .
Safety And Hazards
Phenylethyl 3-methylcaffeate should be handled with care. It is recommended to wear personal protective equipment, ensure adequate ventilation, and avoid contact with skin, eyes, or clothing. Ingestion and inhalation should also be avoided89.
Future Directions
The potential of Phenylethyl 3-methylcaffeate in cancer chemoprevention is promising, particularly in colorectal cancer. However, more research is needed to fully understand its mechanism of action and to validate its efficacy in clinical trials1011.
properties
IUPAC Name |
2-phenylethyl (E)-3-(4-hydroxy-3-methoxyphenyl)prop-2-enoate | |
---|---|---|
Source | PubChem | |
URL | https://pubchem.ncbi.nlm.nih.gov | |
Description | Data deposited in or computed by PubChem | |
InChI |
InChI=1S/C18H18O4/c1-21-17-13-15(7-9-16(17)19)8-10-18(20)22-12-11-14-5-3-2-4-6-14/h2-10,13,19H,11-12H2,1H3/b10-8+ | |
Source | PubChem | |
URL | https://pubchem.ncbi.nlm.nih.gov | |
Description | Data deposited in or computed by PubChem | |
InChI Key |
CZQNYPBIOHVQQN-CSKARUKUSA-N | |
Source | PubChem | |
URL | https://pubchem.ncbi.nlm.nih.gov | |
Description | Data deposited in or computed by PubChem | |
Canonical SMILES |
COC1=C(C=CC(=C1)C=CC(=O)OCCC2=CC=CC=C2)O | |
Source | PubChem | |
URL | https://pubchem.ncbi.nlm.nih.gov | |
Description | Data deposited in or computed by PubChem | |
Isomeric SMILES |
COC1=C(C=CC(=C1)/C=C/C(=O)OCCC2=CC=CC=C2)O | |
Source | PubChem | |
URL | https://pubchem.ncbi.nlm.nih.gov | |
Description | Data deposited in or computed by PubChem | |
Molecular Formula |
C18H18O4 | |
Source | PubChem | |
URL | https://pubchem.ncbi.nlm.nih.gov | |
Description | Data deposited in or computed by PubChem | |
DSSTOX Substance ID |
DTXSID8021143 | |
Record name | Phenylethyl-3-methylcaffeate | |
Source | EPA DSSTox | |
URL | https://comptox.epa.gov/dashboard/DTXSID8021143 | |
Description | DSSTox provides a high quality public chemistry resource for supporting improved predictive toxicology. | |
Molecular Weight |
298.3 g/mol | |
Source | PubChem | |
URL | https://pubchem.ncbi.nlm.nih.gov | |
Description | Data deposited in or computed by PubChem | |
Product Name |
Phenylethyl 3-methylcaffeate | |
CAS RN |
71835-85-3, 132335-98-9 | |
Record name | Phenylethyl 3-methylcaffeate | |
Source | ChemIDplus | |
URL | https://pubchem.ncbi.nlm.nih.gov/substance/?source=chemidplus&sourceid=0071835853 | |
Description | ChemIDplus is a free, web search system that provides access to the structure and nomenclature authority files used for the identification of chemical substances cited in National Library of Medicine (NLM) databases, including the TOXNET system. | |
Record name | Phenylethyl-3-methylcaffeate | |
Source | EPA DSSTox | |
URL | https://comptox.epa.gov/dashboard/DTXSID8021143 | |
Description | DSSTox provides a high quality public chemistry resource for supporting improved predictive toxicology. | |
Record name | Phenethyl ferulate | |
Source | FDA Global Substance Registration System (GSRS) | |
URL | https://gsrs.ncats.nih.gov/ginas/app/beta/substances/B49B43355J | |
Description | The FDA Global Substance Registration System (GSRS) enables the efficient and accurate exchange of information on what substances are in regulated products. Instead of relying on names, which vary across regulatory domains, countries, and regions, the GSRS knowledge base makes it possible for substances to be defined by standardized, scientific descriptions. | |
Explanation | Unless otherwise noted, the contents of the FDA website (www.fda.gov), both text and graphics, are not copyrighted. They are in the public domain and may be republished, reprinted and otherwise used freely by anyone without the need to obtain permission from FDA. Credit to the U.S. Food and Drug Administration as the source is appreciated but not required. | |
Retrosynthesis Analysis
AI-Powered Synthesis Planning: Our tool employs the Template_relevance Pistachio, Template_relevance Bkms_metabolic, Template_relevance Pistachio_ringbreaker, Template_relevance Reaxys, Template_relevance Reaxys_biocatalysis model, leveraging a vast database of chemical reactions to predict feasible synthetic routes.
One-Step Synthesis Focus: Specifically designed for one-step synthesis, it provides concise and direct routes for your target compounds, streamlining the synthesis process.
Accurate Predictions: Utilizing the extensive PISTACHIO, BKMS_METABOLIC, PISTACHIO_RINGBREAKER, REAXYS, REAXYS_BIOCATALYSIS database, our tool offers high-accuracy predictions, reflecting the latest in chemical research and data.
Strategy Settings
Precursor scoring | Relevance Heuristic |
---|---|
Min. plausibility | 0.01 |
Model | Template_relevance |
Template Set | Pistachio/Bkms_metabolic/Pistachio_ringbreaker/Reaxys/Reaxys_biocatalysis |
Top-N result to add to graph | 6 |
Feasible Synthetic Routes
Citations
Disclaimer and Information on In-Vitro Research Products
Please be aware that all articles and product information presented on BenchChem are intended solely for informational purposes. The products available for purchase on BenchChem are specifically designed for in-vitro studies, which are conducted outside of living organisms. In-vitro studies, derived from the Latin term "in glass," involve experiments performed in controlled laboratory settings using cells or tissues. It is important to note that these products are not categorized as medicines or drugs, and they have not received approval from the FDA for the prevention, treatment, or cure of any medical condition, ailment, or disease. We must emphasize that any form of bodily introduction of these products into humans or animals is strictly prohibited by law. It is essential to adhere to these guidelines to ensure compliance with legal and ethical standards in research and experimentation.