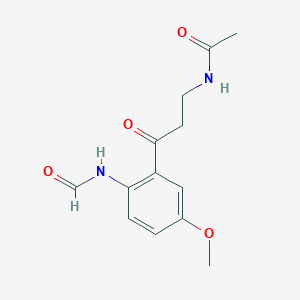
AFMK
Overview
Description
AFMK is a primary metabolite of melatonin, formed via enzymatic, pseudo-enzymatic, or non-enzymatic oxidative pathways. It is generated through pyrrole ring cleavage of melatonin by enzymes such as indoleamine 2,3-dioxygenase (IDO), myeloperoxidase (MPO), and hemoperoxidases, or via interactions with reactive oxygen species (ROS) like hydroxyl radicals and singlet oxygen (¹O₂) . This compound is recognized for its potent antioxidant properties, functioning within a free-radical scavenging cascade alongside melatonin and its tertiary metabolite, AMK (N1-acetyl-5-methoxykynuramine) . Its biosynthesis is context-dependent, observed in specific plants (e.g., rice, water hyacinth) under stress and in mammals during inflammatory or oxidative conditions .
Preparation Methods
Photosensitization with Methylene Blue
Reaction Mechanism and Protocol
AFMK synthesis via photosensitization involves melatonin oxidation under light exposure in the presence of methylene blue and oxygen. The reaction proceeds through a radical chain mechanism, where methylene blue acts as a photosensitizer, generating singlet oxygen () upon illumination . Singlet oxygen cleaves melatonin’s indole ring, forming this compound as the primary product.
Procedure :
-
Reactants : Melatonin (1 mM) dissolved in aqueous solution containing methylene blue (0.12 mg/mL).
-
Conditions : Oxygen saturation via bubbling, exposure to a 500 W tungsten lamp for 2 hours at 0–4°C .
-
Purification : this compound is isolated using high-performance liquid chromatography (HPLC) with a C-8 column and methanol-water gradient .
Yield and Scalability
This method achieves ~60% yield under optimized conditions, significantly higher than non-catalyzed reactions . However, scalability is limited by light penetration depth and prolonged reaction times (Table 1).
Chemical Oxidation Using 3-Chloroperbenzoic Acid
Reaction Design and Parameters
Kennaway et al. (1988) established a chemical oxidation route using 3-chloroperbenzoic acid (mCPBA) in dichloromethane . The reaction proceeds via electrophilic attack on melatonin’s indole ring, forming this compound without requiring light or enzymatic catalysts.
Procedure :
-
Reactants : Melatonin (2 mmol) and mCPBA (11 mmol) in dichloromethane (30 mL).
-
Conditions : Stirring at 4°C for 18 hours under inert atmosphere .
-
Purification : Sequential washing with sodium bicarbonate, drying, and preparative thin-layer chromatography (TLC) with ethyl acetate .
Efficiency and Limitations
This method yields ~32% this compound but requires stoichiometric excess of mCPBA, increasing cost and purification complexity . Side products include deformylated AMK and dimeric species, necessitating rigorous chromatographic separation (Table 1).
Peroxidase-Mediated Catalysis with Chlorpromazine
Optimized Enzymatic Synthesis
Ximenes et al. (2008) developed a peroxidase-catalyzed method using chlorpromazine (CPZ) to enhance this compound production . Horseradish peroxidase (HRP) facilitates hydrogen peroxide ()-driven oxidation, while CPZ stabilizes radical intermediates, accelerating the reaction.
Procedure :
-
Reactants : Melatonin (1 mM), HRP (10 U/mL), (5 mM), and CPZ (0.1 mM) in phosphate buffer (pH 7.4).
Advantages Over Traditional Methods
The CPZ-HRP system achieves ~60% yield in 30 minutes, doubling the efficiency of non-catalyzed methods . Catalytic turnover reduces consumption, making it cost-effective for large-scale synthesis (Table 1).
Comparative Analysis of this compound Synthesis Methods
Table 1: Key Parameters of this compound Preparation Methods
Method | Catalyst/Reagent | Reaction Time | Yield (%) | Purity (%) | Scalability |
---|---|---|---|---|---|
Photosensitization | Methylene blue + | 2 hours | 60 | 85 | Moderate |
Chemical Oxidation | mCPBA | 18 hours | 32 | 78 | Low |
Peroxidase-Catalyzed | HRP + CPZ | 0.5 hours | 60 | 90 | High |
Key Findings :
-
Photosensitization and peroxidase-catalyzed methods offer superior yields (60%) but differ in reaction kinetics and equipment requirements .
-
Chemical oxidation is simpler but less efficient, limited by side reactions .
-
Deuterated this compound (this compound-D3), used as an internal standard in mass spectrometry, is synthesized via photosensitization of deuterated melatonin .
Mechanistic Insights and Side Reactions
Radical Intermediates in Photosensitization
The methylene blue method generates melatonin radicals via Type II (singlet oxygen) mechanisms, confirmed by electron paramagnetic resonance (EPR) studies . Competing pathways may produce hydroxylated byproducts, necessitating pH control (optimal pH 7.4) .
Byproduct Formation in Chemical Oxidation
Excess mCPBA promotes over-oxidation, yielding AMK (-acetyl-5-methoxykynuramine) and kynuramine dimers . Chelating agents (e.g., EDTA) mitigate metal-catalyzed degradation during purification .
Chemical Reactions Analysis
Types of Reactions: Bicuculline undergoes various chemical reactions, including oxidation, reduction, and substitution reactions. It is particularly sensitive to light and can degrade under physiological conditions .
Common Reagents and Conditions: Common reagents used in the reactions involving bicuculline include chloroform, benzene, ethyl acetate, and methiodide. The reactions are typically carried out under controlled conditions to prevent degradation of the compound .
Major Products Formed: The major products formed from the reactions involving bicuculline include its methiodide form, which is water-soluble and more stable under physiological conditions .
Scientific Research Applications
Biological and Pharmacological Applications
Antioxidant Properties
AFMK exhibits significant antioxidant activity, which has implications in protecting cells from oxidative stress. It has been shown to scavenge free radicals and reduce oxidative damage in various biological systems. This property is particularly relevant in neuroprotection, where this compound may mitigate damage associated with neurodegenerative diseases.
Case Study: Neuroprotection
In a study involving neuronal cells exposed to oxidative stress, treatment with this compound resulted in reduced cell death and improved cell viability compared to untreated controls. The mechanism was attributed to the upregulation of antioxidant enzymes and downregulation of pro-apoptotic factors .
Role in Melatonin Metabolism
This compound is a metabolite of melatonin, and its formation is enhanced under conditions of oxidative stress. Understanding its role in melatonin metabolism can provide insights into the physiological effects of melatonin supplementation.
Table 1: Comparison of Melatonin and Its Metabolites
Compound | Chemical Structure | Antioxidant Activity | Neuroprotective Effects |
---|---|---|---|
Melatonin | C13H16N2O2S | Moderate | Yes |
This compound | C11H12N2O3 | High | Yes |
AMK | C10H10N2O2 | Low | Limited |
Implications in Obesity Management
Recent research has indicated that this compound may play a role in the management of obesity and related disorders. It has been linked to the modulation of metabolic pathways that influence body weight regulation.
Case Study: Obesity Management
A clinical trial assessing the effects of melatonin supplementation (which increases this compound levels) on obese patients showed significant reductions in body mass index (BMI) and improvements in metabolic markers such as glucose tolerance and lipid profiles .
Environmental Applications
This compound's antioxidant properties extend beyond biological systems; it also shows promise in environmental applications, particularly in mitigating UV-induced damage in aquatic ecosystems.
Case Study: UV Radiation Protection
Research demonstrated that this compound can protect marine organisms from UV-induced oxidative stress, suggesting its potential use as a natural protective agent against environmental stressors .
Mechanism of Action
Bicuculline exerts its effects by blocking gamma-aminobutyric acid type A receptors, which are ligand-gated ion channels responsible for the passing of chloride ions across the cell membrane . By inhibiting these receptors, bicuculline prevents gamma-aminobutyric acid from exerting its inhibitory effect, leading to increased neuronal excitability and convulsions . The compound also blocks calcium-activated potassium channels, further contributing to its convulsant action .
Comparison with Similar Compounds
Comparison with Structurally and Functionally Related Compounds
AFMK vs. AMK
- Formation Pathways: AMK derives from this compound via deformylation. Both metabolites are produced enzymatically (IDO, MPO) and non-enzymatically (ROS interactions) .
- Antioxidant Capacity :
- Biological Significance: In human epidermis, this compound and AMK accumulate and protect against UV-induced oxidative stress, with AMK showing antiproliferative effects on melanoma cells . In mice, however, this compound/AMK pathways are minor (<0.01% urinary excretion), overshadowed by the 6-hydroxylation pathway of melatonin .
This compound vs. Melatonin and NAS (N-Acetylserotonin)
- Antioxidant Hierarchy :
This compound vs. Glutathione (GSH)
- Mechanism : GSH directly conjugates with electrophiles, whereas this compound neutralizes ROS via electron donation .
- Efficiency : this compound reduces neuronal death in cultured hippocampal cells at micromolar concentrations, comparable to GSH in mitigating oxidative damage .
Key Research Findings and Data Tables
Table 1: Antioxidant Efficiency of this compound and Related Compounds
Table 2: Tissue-Specific Presence of this compound/AMK
Species/Tissue | This compound Detected? | AMK Detected? | Conditions | Reference |
---|---|---|---|---|
Mouse urine | No | No | Oral melatonin treatment | |
Human epidermis | Yes | Yes | UV exposure | |
Rice plants | Yes | N/A | Diurnal light cycles |
Controversies and Methodological Considerations
- Species-Specific Discrepancies : this compound is prominent in human skin and rat brains under inflammation but negligible in mice, suggesting metabolic pathway variations .
- Detection Limitations : Early studies using radioactive labeling identified this compound in rat brains, while advanced metabolomics (UPLC-TOFMS) found minimal traces in mice, highlighting sensitivity differences .
- Stress Dependence: this compound formation in plants (e.g., Eichhornia) correlates with melatonin levels under photochemical stress, but its enzymatic vs. non-enzymatic origins remain unclear .
Biological Activity
AFMK, or N-acetyl-5-methoxytryptamine, is a melatonin metabolite known for its diverse biological activities, particularly in the context of oxidative stress and cellular signaling. This article explores the biological activity of this compound, summarizing key research findings, mechanisms of action, and implications for health.
Overview of this compound
This compound is derived from melatonin through enzymatic processes and is recognized for its antioxidant properties. It plays a significant role in protecting cells from oxidative damage caused by reactive oxygen species (ROS). The compound exhibits various biological activities that contribute to its potential therapeutic effects.
This compound's biological activity can be attributed to several mechanisms:
- Antioxidant Activity : this compound scavenges free radicals and reduces oxidative stress, which is crucial in preventing cellular damage. Studies have shown that this compound can effectively neutralize ROS, thereby protecting cellular components such as lipids, proteins, and DNA from oxidative damage .
- Regulation of Cell Signaling : this compound influences various signaling pathways, including those related to apoptosis and cell survival. It has been reported to modulate the activity of several kinases involved in cell proliferation and survival .
- Neuroprotective Effects : Research indicates that this compound may protect neuronal cells from excitotoxicity and neurodegeneration. It appears to mitigate the effects of glutamate-induced toxicity in neuronal cultures by modulating intracellular calcium levels and reducing oxidative stress .
Biological Activities and Therapeutic Implications
This compound's diverse biological activities suggest potential therapeutic applications in various health conditions:
- Cancer : this compound has been investigated for its anti-cancer properties. In vitro studies demonstrate that it can inhibit the proliferation of cancer cells by inducing apoptosis through caspase activation and modulating cell cycle progression .
- Neurological Disorders : Given its neuroprotective properties, this compound may be beneficial in treating neurodegenerative diseases such as Alzheimer's and Parkinson's disease. Its ability to reduce oxidative stress and inflammation in neuronal tissues highlights its potential as a therapeutic agent .
- Inflammation : this compound has shown anti-inflammatory effects by inhibiting pro-inflammatory cytokines and reducing oxidative stress markers in various models of inflammation .
Case Studies
- Neuroprotection Against Glutamate Toxicity :
- Anticancer Activity :
- Oxidative Stress Reduction :
Summary Table of Biological Activities
Biological Activity | Mechanism | Implications |
---|---|---|
Antioxidant | Scavenges free radicals | Protects against oxidative damage |
Anti-cancer | Induces apoptosis; inhibits proliferation | Potential treatment for tumors |
Neuroprotection | Reduces excitotoxicity; protects neurons | May aid in neurodegenerative diseases |
Anti-inflammatory | Inhibits pro-inflammatory cytokines | Useful in inflammatory conditions |
Q & A
Basic Research Questions
Q. What are the primary mechanisms underlying AFMK's antioxidant activity in cellular models?
this compound scavenges reactive oxygen species (ROS) through both enzymatic and non-enzymatic pathways. It donates multiple electrons to neutralize hydroxyl radicals (•OH) and peroxynitrite (ONOO⁻), with an IC50 of 338.08 nM for •OH scavenging in vitro . Unlike vitamins C and E, this compound can undergo sequential oxidation, producing metabolites like AMK that retain antioxidant capacity. In biological models, this compound reduces DNA damage (e.g., 8-OH-dG levels) and lipid peroxidation in a dose-dependent manner .
Q. How does this compound influence apoptotic pathways in cancer cells?
this compound modulates apoptosis by inhibiting heat shock protein 70 (HSP70) production. In PANC-1 pancreatic cancer cells, this compound (0.001–10 nM) synergizes with Gemcitabine, reducing HSP70 levels from 0.47 (Gemcitabine alone) to 0.01 . This suppression enhances chemotherapeutic efficacy by destabilizing cancer cell survival mechanisms. Additionally, this compound pretreatment reduces DNA damage markers, such as comet tail length, by 50% in irradiated models .
Advanced Research Questions
Q. How can researchers design experiments to assess this compound's synergistic effects with chemotherapy agents like Gemcitabine?
- In vitro: Use dose-response matrices (e.g., 0.001–100 nM this compound + IC50 Gemcitabine) in cancer cell lines (e.g., PANC-1). Measure apoptosis via flow cytometry (Annexin V/PI staining) and HSP70 expression via Western blot .
- In vivo: Administer this compound (10 mg/kg, intraperitoneal) with Gemcitabine in xenograft models. Monitor tumor volume and oxidative stress markers (e.g., plasma total antioxidant capacity) .
- Controls: Include vehicle-only and single-agent groups to isolate synergistic effects.
Q. What methodological approaches are used to quantify this compound levels in human serum?
- Sample Preparation: Extract serum using liquid-liquid separation (e.g., acetonitrile/dichloromethane) to isolate this compound from melatonin and other metabolites .
- Quantification: Employ LC-MS/MS with a 11-point calibration curve (R² > 0.99) for sensitivity. Validate with spike-recovery tests (85–115% recovery range) .
- Confounders: Control for circadian rhythms by collecting samples at consistent times (e.g., 2:00–4:00 AM) .
Q. How can researchers resolve contradictions between in vitro and in vivo efficacy data of this compound?
- Bioavailability: Assess this compound’s stability in plasma using pharmacokinetic profiling. For example, its short half-life in vivo may require sustained-release formulations .
- Metabolic Conversion: Track this compound-to-AMK conversion rates in different tissues via isotopic labeling. AMK exhibits stronger ROS scavenging but lower tissue retention than this compound .
- Model Selection: Use transgenic mice (e.g., IDO1-knockout) to isolate this compound-specific effects from endogenous melatonin pathways .
Q. What statistical methods are appropriate for analyzing this compound's correlation with clinical outcomes?
- Multivariate Regression: Adjust for confounders (e.g., age, sleep quality) when linking serum this compound levels to tumor size or metastasis .
- Non-Parametric Tests: Apply Spearman’s rank correlation (ρ) for non-normal distributions, such as this compound’s association with lymph node involvement (ρ = 0.32, p < 0.05) .
- Survival Analysis: Use Cox proportional hazards models to evaluate this compound’s predictive value for progression-free survival in breast cancer cohorts .
Properties
IUPAC Name |
N-[3-(2-formamido-5-methoxyphenyl)-3-oxopropyl]acetamide | |
---|---|---|
Source | PubChem | |
URL | https://pubchem.ncbi.nlm.nih.gov | |
Description | Data deposited in or computed by PubChem | |
InChI |
InChI=1S/C13H16N2O4/c1-9(17)14-6-5-13(18)11-7-10(19-2)3-4-12(11)15-8-16/h3-4,7-8H,5-6H2,1-2H3,(H,14,17)(H,15,16) | |
Source | PubChem | |
URL | https://pubchem.ncbi.nlm.nih.gov | |
Description | Data deposited in or computed by PubChem | |
InChI Key |
JYWNYMJKURVPFH-UHFFFAOYSA-N | |
Source | PubChem | |
URL | https://pubchem.ncbi.nlm.nih.gov | |
Description | Data deposited in or computed by PubChem | |
Canonical SMILES |
CC(=O)NCCC(=O)C1=C(C=CC(=C1)OC)NC=O | |
Source | PubChem | |
URL | https://pubchem.ncbi.nlm.nih.gov | |
Description | Data deposited in or computed by PubChem | |
Molecular Formula |
C13H16N2O4 | |
Source | PubChem | |
URL | https://pubchem.ncbi.nlm.nih.gov | |
Description | Data deposited in or computed by PubChem | |
DSSTOX Substance ID |
DTXSID90200451 | |
Record name | N-(3-(2-(Formylamino)-5-methoxyphenyl)-3-oxopropyl)-Acetamide | |
Source | EPA DSSTox | |
URL | https://comptox.epa.gov/dashboard/DTXSID90200451 | |
Description | DSSTox provides a high quality public chemistry resource for supporting improved predictive toxicology. | |
Molecular Weight |
264.28 g/mol | |
Source | PubChem | |
URL | https://pubchem.ncbi.nlm.nih.gov | |
Description | Data deposited in or computed by PubChem | |
Physical Description |
Solid | |
Record name | Acetyl-N-formyl-5-methoxykynurenamine | |
Source | Human Metabolome Database (HMDB) | |
URL | http://www.hmdb.ca/metabolites/HMDB0004259 | |
Description | The Human Metabolome Database (HMDB) is a freely available electronic database containing detailed information about small molecule metabolites found in the human body. | |
Explanation | HMDB is offered to the public as a freely available resource. Use and re-distribution of the data, in whole or in part, for commercial purposes requires explicit permission of the authors and explicit acknowledgment of the source material (HMDB) and the original publication (see the HMDB citing page). We ask that users who download significant portions of the database cite the HMDB paper in any resulting publications. | |
CAS No. |
52450-38-1 | |
Record name | N1-Acetyl-N2-formyl-5-methoxykynuramine | |
Source | CAS Common Chemistry | |
URL | https://commonchemistry.cas.org/detail?cas_rn=52450-38-1 | |
Description | CAS Common Chemistry is an open community resource for accessing chemical information. Nearly 500,000 chemical substances from CAS REGISTRY cover areas of community interest, including common and frequently regulated chemicals, and those relevant to high school and undergraduate chemistry classes. This chemical information, curated by our expert scientists, is provided in alignment with our mission as a division of the American Chemical Society. | |
Explanation | The data from CAS Common Chemistry is provided under a CC-BY-NC 4.0 license, unless otherwise stated. | |
Record name | N-Acetyl-N-formyl-5-methoxykynurenamine | |
Source | ChemIDplus | |
URL | https://pubchem.ncbi.nlm.nih.gov/substance/?source=chemidplus&sourceid=0052450381 | |
Description | ChemIDplus is a free, web search system that provides access to the structure and nomenclature authority files used for the identification of chemical substances cited in National Library of Medicine (NLM) databases, including the TOXNET system. | |
Record name | N-(3-(2-(Formylamino)-5-methoxyphenyl)-3-oxopropyl)-Acetamide | |
Source | EPA DSSTox | |
URL | https://comptox.epa.gov/dashboard/DTXSID90200451 | |
Description | DSSTox provides a high quality public chemistry resource for supporting improved predictive toxicology. | |
Record name | N-[3-[2-(Formylamino)-5-methoxyphenyl]-3-oxopropyl]acetamide | |
Source | European Chemicals Agency (ECHA) | |
URL | https://echa.europa.eu/information-on-chemicals | |
Description | The European Chemicals Agency (ECHA) is an agency of the European Union which is the driving force among regulatory authorities in implementing the EU's groundbreaking chemicals legislation for the benefit of human health and the environment as well as for innovation and competitiveness. | |
Explanation | Use of the information, documents and data from the ECHA website is subject to the terms and conditions of this Legal Notice, and subject to other binding limitations provided for under applicable law, the information, documents and data made available on the ECHA website may be reproduced, distributed and/or used, totally or in part, for non-commercial purposes provided that ECHA is acknowledged as the source: "Source: European Chemicals Agency, http://echa.europa.eu/". Such acknowledgement must be included in each copy of the material. ECHA permits and encourages organisations and individuals to create links to the ECHA website under the following cumulative conditions: Links can only be made to webpages that provide a link to the Legal Notice page. | |
Record name | N1-ACETYL-N2-FORMYL-5-METHOXYKYNURAMINE | |
Source | FDA Global Substance Registration System (GSRS) | |
URL | https://gsrs.ncats.nih.gov/ginas/app/beta/substances/S82P8JZ4YJ | |
Description | The FDA Global Substance Registration System (GSRS) enables the efficient and accurate exchange of information on what substances are in regulated products. Instead of relying on names, which vary across regulatory domains, countries, and regions, the GSRS knowledge base makes it possible for substances to be defined by standardized, scientific descriptions. | |
Explanation | Unless otherwise noted, the contents of the FDA website (www.fda.gov), both text and graphics, are not copyrighted. They are in the public domain and may be republished, reprinted and otherwise used freely by anyone without the need to obtain permission from FDA. Credit to the U.S. Food and Drug Administration as the source is appreciated but not required. | |
Record name | Acetyl-N-formyl-5-methoxykynurenamine | |
Source | Human Metabolome Database (HMDB) | |
URL | http://www.hmdb.ca/metabolites/HMDB0004259 | |
Description | The Human Metabolome Database (HMDB) is a freely available electronic database containing detailed information about small molecule metabolites found in the human body. | |
Explanation | HMDB is offered to the public as a freely available resource. Use and re-distribution of the data, in whole or in part, for commercial purposes requires explicit permission of the authors and explicit acknowledgment of the source material (HMDB) and the original publication (see the HMDB citing page). We ask that users who download significant portions of the database cite the HMDB paper in any resulting publications. | |
Retrosynthesis Analysis
AI-Powered Synthesis Planning: Our tool employs the Template_relevance Pistachio, Template_relevance Bkms_metabolic, Template_relevance Pistachio_ringbreaker, Template_relevance Reaxys, Template_relevance Reaxys_biocatalysis model, leveraging a vast database of chemical reactions to predict feasible synthetic routes.
One-Step Synthesis Focus: Specifically designed for one-step synthesis, it provides concise and direct routes for your target compounds, streamlining the synthesis process.
Accurate Predictions: Utilizing the extensive PISTACHIO, BKMS_METABOLIC, PISTACHIO_RINGBREAKER, REAXYS, REAXYS_BIOCATALYSIS database, our tool offers high-accuracy predictions, reflecting the latest in chemical research and data.
Strategy Settings
Precursor scoring | Relevance Heuristic |
---|---|
Min. plausibility | 0.01 |
Model | Template_relevance |
Template Set | Pistachio/Bkms_metabolic/Pistachio_ringbreaker/Reaxys/Reaxys_biocatalysis |
Top-N result to add to graph | 6 |
Feasible Synthetic Routes
Disclaimer and Information on In-Vitro Research Products
Please be aware that all articles and product information presented on BenchChem are intended solely for informational purposes. The products available for purchase on BenchChem are specifically designed for in-vitro studies, which are conducted outside of living organisms. In-vitro studies, derived from the Latin term "in glass," involve experiments performed in controlled laboratory settings using cells or tissues. It is important to note that these products are not categorized as medicines or drugs, and they have not received approval from the FDA for the prevention, treatment, or cure of any medical condition, ailment, or disease. We must emphasize that any form of bodily introduction of these products into humans or animals is strictly prohibited by law. It is essential to adhere to these guidelines to ensure compliance with legal and ethical standards in research and experimentation.