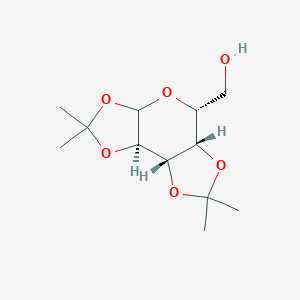
1,2:3,4-Di-O-isopropylidene-alpha-D-galactopyranose
Overview
Description
Synthesis Analysis
The synthesis of 1,2:3,4-Di-O-isopropylidene-α-D-galactopyranose involves the reaction of galactopyranosides with 2,2-dimethoxypropane, leading to various isopropylidene acetals, among which the 3,4-O-isopropylidene derivatives can be obtained with high yield. These intermediates are valuable for selective syntheses of substituted galactose derivatives (Barili et al., 1986).
Molecular Structure Analysis
The molecular structure of derivatives related to 1,2:3,4-Di-O-isopropylidene-α-D-galactopyranose has been elucidated through X-ray crystallography, showing variations in ring conformations. For example, the crystal structure of a related methyl derivative reveals slightly distorted β-D-galactosyl rings due to the isopropylidene group, indicating that the modification impacts the ring's conformation and, subsequently, its chemical behavior (Hoogendorp, Kok, & Romers, 1983).
Chemical Reactions and Properties
1,2:3,4-Di-O-isopropylidene-α-D-galactopyranose undergoes various chemical reactions, demonstrating its utility in synthesizing complex molecules. For instance, its reaction with epichlorohydrin yields diastereomers, highlighting its role in producing compounds with specific chiral centers (Köll et al., 1994).
Physical Properties Analysis
The physical properties, such as crystal structure and solubility, are influenced by the isopropylidene group. The crystallographic study of a related derivative shows an orthorhombic system with specific cell dimensions, indicating that the protective groups can significantly affect the crystalline form and stability of the compound (Krajewski et al., 1986).
Chemical Properties Analysis
The chemical properties of 1,2:3,4-Di-O-isopropylidene-α-D-galactopyranose, such as reactivity towards different chemical reagents, are pivotal for its application in synthetic chemistry. Studies show that the compound serves as a versatile intermediate for generating various galactose derivatives, demonstrating selective reactivity that can be harnessed for constructing complex molecular architectures (Barili et al., 1986).
Scientific Research Applications
Polymerization Applications : It's used in the polymerization of acrylamide (Shasha, Doane, & Russell, 1971).
Synthesis of ROMP Homopolymers and Multi-Block Copolymers : This compound aids in synthesizing ROMP homopolymers and multi-block copolymers containing sugars with low polydispersities (Nomura & Schrock, 1995).
Starting Material for Various Derivatives : It serves as a starting material for synthesizing various derivatives, including carbonyl hydrazones and aryl hydrazones (Liao Gen, Qian Zhao, & C. Ling, 2005).
Applications in Organic Synthesis and Structural Biology : Its synthesis has potential applications in organic synthesis and structural biology (Krajewski et al., 1985).
Sugar Ortho Ester Synthesis : It is used in synthesizing sugar ortho esters from various sources (Bochkov & Kalinevitch, 1974).
Low Toxicity for Biological/Pharmacological Studies : This compound is non-toxic, making it promising for future biological and/or pharmacological studies (Medeiros et al., 2020).
Carcinostatic Activity : Exhibits carcinostatic activity in certain systems, like mouse lymphocytic leukemia (Daniel & Zingaro, 1978).
Photochemical Reactions : Useful in photochemical reactions in carbohydrates (Binkley, 1969).
Aminodeoxy Sugar Synthesis : Applied in aminodeoxy sugar synthesis, though the product yields are low for general value (Flechtner & Pohlid, 1984).
X-ray Diffraction Studies : Its structure has been solved by X-ray diffraction methods, contributing to a better understanding of its conformation (Krajewski et al., 1986).
Safety And Hazards
1,2:3,4-Di-O-isopropylidene-alpha-D-galactopyranose is considered moisture-sensitive . Proper storage under inert gas conditions is recommended to prevent degradation. It is classified as a combustible liquid (Storage Class Code 10) and has a WGK 3 rating.
Future Directions
Research on 1,2:3,4-Di-O-isopropylidene-alpha-D-galactopyranose continues to explore its applications in carbohydrate-based drug design, synthetic chemistry, and bioconjugation strategies. Investigating its interactions with enzymes and receptors may reveal novel therapeutic targets.
properties
IUPAC Name |
[(1S,2R,6R,8R,9S)-4,4,11,11-tetramethyl-3,5,7,10,12-pentaoxatricyclo[7.3.0.02,6]dodecan-8-yl]methanol | |
---|---|---|
Source | PubChem | |
URL | https://pubchem.ncbi.nlm.nih.gov | |
Description | Data deposited in or computed by PubChem | |
InChI |
InChI=1S/C12H20O6/c1-11(2)15-7-6(5-13)14-10-9(8(7)16-11)17-12(3,4)18-10/h6-10,13H,5H2,1-4H3/t6-,7+,8+,9-,10-/m1/s1 | |
Source | PubChem | |
URL | https://pubchem.ncbi.nlm.nih.gov | |
Description | Data deposited in or computed by PubChem | |
InChI Key |
POORJMIIHXHXAV-SOYHJAILSA-N | |
Source | PubChem | |
URL | https://pubchem.ncbi.nlm.nih.gov | |
Description | Data deposited in or computed by PubChem | |
Canonical SMILES |
CC1(OC2C(OC3C(C2O1)OC(O3)(C)C)CO)C | |
Source | PubChem | |
URL | https://pubchem.ncbi.nlm.nih.gov | |
Description | Data deposited in or computed by PubChem | |
Isomeric SMILES |
CC1(O[C@H]2[C@H](O[C@H]3[C@@H]([C@H]2O1)OC(O3)(C)C)CO)C | |
Source | PubChem | |
URL | https://pubchem.ncbi.nlm.nih.gov | |
Description | Data deposited in or computed by PubChem | |
Molecular Formula |
C12H20O6 | |
Source | PubChem | |
URL | https://pubchem.ncbi.nlm.nih.gov | |
Description | Data deposited in or computed by PubChem | |
DSSTOX Substance ID |
DTXSID601347764 | |
Record name | 1,2:3,4-Di-O-isopropylidene-alpha-D-galactopyranose | |
Source | EPA DSSTox | |
URL | https://comptox.epa.gov/dashboard/DTXSID601347764 | |
Description | DSSTox provides a high quality public chemistry resource for supporting improved predictive toxicology. | |
Molecular Weight |
260.28 g/mol | |
Source | PubChem | |
URL | https://pubchem.ncbi.nlm.nih.gov | |
Description | Data deposited in or computed by PubChem | |
Product Name |
1,2:3,4-Di-O-isopropylidene-alpha-D-galactopyranose | |
CAS RN |
4064-06-6 | |
Record name | 1,2:3,4-Di-O-isopropylidene-α-D-galactopyranose | |
Source | CAS Common Chemistry | |
URL | https://commonchemistry.cas.org/detail?cas_rn=4064-06-6 | |
Description | CAS Common Chemistry is an open community resource for accessing chemical information. Nearly 500,000 chemical substances from CAS REGISTRY cover areas of community interest, including common and frequently regulated chemicals, and those relevant to high school and undergraduate chemistry classes. This chemical information, curated by our expert scientists, is provided in alignment with our mission as a division of the American Chemical Society. | |
Explanation | The data from CAS Common Chemistry is provided under a CC-BY-NC 4.0 license, unless otherwise stated. | |
Record name | 1,2:3,4-Di-O-isopropylidene-alpha-D-galactopyranose | |
Source | EPA DSSTox | |
URL | https://comptox.epa.gov/dashboard/DTXSID601347764 | |
Description | DSSTox provides a high quality public chemistry resource for supporting improved predictive toxicology. | |
Record name | 1,2:3,4-di-O-isopropylidene-D-galactopyranose | |
Source | European Chemicals Agency (ECHA) | |
URL | https://echa.europa.eu/substance-information/-/substanceinfo/100.021.611 | |
Description | The European Chemicals Agency (ECHA) is an agency of the European Union which is the driving force among regulatory authorities in implementing the EU's groundbreaking chemicals legislation for the benefit of human health and the environment as well as for innovation and competitiveness. | |
Explanation | Use of the information, documents and data from the ECHA website is subject to the terms and conditions of this Legal Notice, and subject to other binding limitations provided for under applicable law, the information, documents and data made available on the ECHA website may be reproduced, distributed and/or used, totally or in part, for non-commercial purposes provided that ECHA is acknowledged as the source: "Source: European Chemicals Agency, http://echa.europa.eu/". Such acknowledgement must be included in each copy of the material. ECHA permits and encourages organisations and individuals to create links to the ECHA website under the following cumulative conditions: Links can only be made to webpages that provide a link to the Legal Notice page. | |
Retrosynthesis Analysis
AI-Powered Synthesis Planning: Our tool employs the Template_relevance Pistachio, Template_relevance Bkms_metabolic, Template_relevance Pistachio_ringbreaker, Template_relevance Reaxys, Template_relevance Reaxys_biocatalysis model, leveraging a vast database of chemical reactions to predict feasible synthetic routes.
One-Step Synthesis Focus: Specifically designed for one-step synthesis, it provides concise and direct routes for your target compounds, streamlining the synthesis process.
Accurate Predictions: Utilizing the extensive PISTACHIO, BKMS_METABOLIC, PISTACHIO_RINGBREAKER, REAXYS, REAXYS_BIOCATALYSIS database, our tool offers high-accuracy predictions, reflecting the latest in chemical research and data.
Strategy Settings
Precursor scoring | Relevance Heuristic |
---|---|
Min. plausibility | 0.01 |
Model | Template_relevance |
Template Set | Pistachio/Bkms_metabolic/Pistachio_ringbreaker/Reaxys/Reaxys_biocatalysis |
Top-N result to add to graph | 6 |
Feasible Synthetic Routes
Citations
Disclaimer and Information on In-Vitro Research Products
Please be aware that all articles and product information presented on BenchChem are intended solely for informational purposes. The products available for purchase on BenchChem are specifically designed for in-vitro studies, which are conducted outside of living organisms. In-vitro studies, derived from the Latin term "in glass," involve experiments performed in controlled laboratory settings using cells or tissues. It is important to note that these products are not categorized as medicines or drugs, and they have not received approval from the FDA for the prevention, treatment, or cure of any medical condition, ailment, or disease. We must emphasize that any form of bodily introduction of these products into humans or animals is strictly prohibited by law. It is essential to adhere to these guidelines to ensure compliance with legal and ethical standards in research and experimentation.