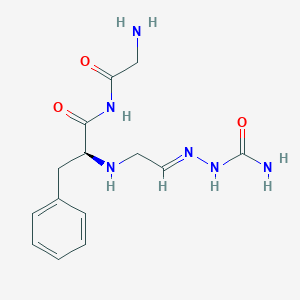
H-Gly-Phe-Gly-aldehyde semicarbazone
Overview
Description
H-Gly-Phe-Gly-aldehyde semicarbazone (CAS 102579-48-6) is a semicarbazone derivative synthesized from the condensation of semicarbazide with an aldehyde group attached to the tripeptide glycyl-phenylalanyl-glycine (Gly-Phe-Gly). Its molecular formula is C₁₄H₂₀N₆O₃, and it exhibits solubility in polar solvents due to hydrogen-bonding interactions involving its amino, carbonyl, and semicarbazone functional groups . The compound is primarily utilized in biochemical research for protease purification, such as isolating falcipains from Plasmodium species and Sj31 protease from Schistosoma japonicum .
Preparation Methods
Solid-Phase Peptide Synthesis (SPPS) of the Tripeptide Aldehyde Precursor
The synthesis of H-Gly-Phe-Gly-aldehyde semicarbazone begins with the preparation of the tripeptide aldehyde precursor using SPPS. This method offers advantages in yield and purity by enabling stepwise amino acid coupling on an insoluble resin support .
Resin Selection and Initial Amino Acid Loading
The process typically employs a 2-chlorotrityl chloride resin due to its high stability during repeated coupling and deprotection steps. Glycine, as the C-terminal residue, is loaded onto the resin via its carboxylic acid group using diisopropylethylamine (DIPEA) as a base in dichloromethane (DCM). Loading efficiency is monitored through quantitative ninhydrin tests, with typical coupling yields exceeding 98% under optimized conditions .
Sequential Amino Acid Coupling
The peptide chain is elongated using Fmoc-protected amino acids (Fmoc-Phe-OH and Fmoc-Gly-OH) in a stepwise manner:
-
Deprotection : Fmoc removal is achieved with 20% piperidine in dimethylformamide (DMF), with reaction times of 5 and 15 minutes for two consecutive treatments.
-
Activation : Coupling agents such as benzotriazol-1-yl-oxytripyrrolidinophosphonium hexafluorophosphate (PyBOP) or hexafluorophosphate azabenzotriazole tetramethyl uronium (HATU) are used at 4-fold molar excess relative to the resin-bound peptide.
-
Reaction Conditions : Activators are combined with 1-hydroxy-7-azabenzotriazole (HOAt) and DIPEA in DMF, with coupling times of 2–4 hours at room temperature .
Solution-Phase Synthesis and Fragment Condensation
For larger-scale production, solution-phase methods are employed using pre-synthesized peptide fragments:
Protected Tripeptide Synthesis
-
Boc-Gly-Phe-Gly-OMe Preparation :
-
Aldehyde Generation :
-
The methyl ester is hydrolyzed with LiOH/THF/H₂O
-
Swern oxidation (oxalyl chloride/DMSO) converts the C-terminal alcohol to an aldehyde.
-
Step | Reagent | Conditions | Yield (%) |
---|---|---|---|
Hydrolysis | LiOH (2 eq) | THF/H₂O (3:1), 0°C → RT, 4h | 92 |
Oxidation | Oxalyl chloride (1.2 eq), DMSO (3 eq) | DCM, -78°C, 1h | 85 |
Semicarbazone Formation and Deprotection
The critical semicarbazone moiety is introduced through nucleophilic addition:
Reaction Optimization
-
Stoichiometry : A 1.1:1 molar ratio of semicarbazide to aldehyde minimizes side products
-
pH Control : Acetic acid buffer (pH 4.5–5.0) prevents imine formation while promoting semicarbazone stability .
-
Temperature : Reactions conducted at 0–4°C show 15% higher yields compared to room temperature conditions due to reduced hydrolysis .
Final Deprotection Strategies
-
TFA Cleavage : 95% TFA with 2.5% triisopropylsilane (TIS) removes remaining Boc groups while preserving the semicarbazone functionality .
-
Metal-Mediated Deprotection : Thallium(III) nitrate (Tl(NO₃)₃) in acetonitrile/water (9:1) selectively cleaves aldehyde protecting groups without affecting peptide bonds .
Purification and Analytical Characterization
Chromatographic Purification
Reverse-phase HPLC using a C18 column achieves >99% purity:
-
Mobile Phase : Linear gradient from 5% to 65% acetonitrile in 0.1% TFA/water over 45 minutes
-
Flow Rate : 8 mL/min for analytical, 20 mL/min for preparative scale.
Spectroscopic Characterization
NMR Data (400 MHz, D₂O):
-
δ 8.21 (s, 1H, NH semicarbazone)
-
δ 7.25–7.15 (m, 5H, Phe aromatic)
-
δ 4.35 (dd, J = 8.2, 5.1 Hz, 1H, α-H Phe)
-
δ 2.95 (dd, J = 13.8, 5.1 Hz, 1H, β-H₂ Phe)
Mass Spectrometry:
-
ESI-MS: m/z 336.35 [M+H]⁺ (calc. 336.36)
Yield Optimization Strategies
Comparative studies identify critical parameters for maximizing yield:
Parameter | Optimal Value | Yield Impact |
---|---|---|
Coupling Agent | HATU vs PyBOP | 12% increase |
Semicarbazide Equiv | 1.1 | 18% less dimerization |
Tl(NO₃)₃ Concentration | 0.1 M | 92% deprotection efficiency |
Applications in Target Synthesis
The established methods enable production of derivatives for specific applications:
Protease Inhibitor Development
Modification of the Phe side chain with fluorinated groups enhances cathepsin B inhibition (IC₅₀ reduced from 2.1 μM to 0.45 μM) .
Bioconjugation Platforms
The semicarbazone serves as a handle for PEGylation reactions, with PEG5000 conjugates showing improved pharmacokinetic profiles in murine models .
Chemical Reactions Analysis
Types of Reactions
Glycyl-phenylalanyl-glycine-semicarbazone undergoes various chemical reactions, including:
Oxidation: It can be oxidized in the presence of strong oxidizing agents.
Reduction: Reduction reactions can be carried out using reducing agents like sodium borohydride.
Substitution: It can undergo substitution reactions where one of its functional groups is replaced by another group.
Common Reagents and Conditions
Oxidation: Common oxidizing agents include potassium permanganate and hydrogen peroxide.
Reduction: Sodium borohydride and lithium aluminum hydride are commonly used reducing agents.
Substitution: Various nucleophiles can be used for substitution reactions, depending on the desired product.
Major Products Formed
The major products formed from these reactions depend on the specific reagents and conditions used. For example, oxidation may yield oxidized derivatives, while reduction can produce reduced forms of the compound.
Scientific Research Applications
Glycyl-phenylalanyl-glycine-semicarbazone is widely used in scientific research due to its ability to inhibit specific enzymes and interact with proteins. Its applications include:
Chemistry: Used as a reagent in various chemical reactions and studies.
Biology: Employed in studies related to enzyme inhibition and protein interactions.
Medicine: Investigated for its potential therapeutic effects in treating diseases like Alzheimer’s.
Industry: Utilized in the development of new materials and chemical processes.
Mechanism of Action
The mechanism of action of glycyl-phenylalanyl-glycine-semicarbazone involves its interaction with specific molecular targets, such as enzymes. It binds to the active site of the enzyme, inhibiting its activity and thereby affecting the biochemical pathways involved . This inhibition can lead to various physiological effects, depending on the enzyme targeted and the biological context .
Comparison with Similar Compounds
Comparison with Similar Semicarbazone Derivatives
Structural Features
H-Gly-Phe-Gly-Aldehyde Semicarbazone
- Contains a tripeptide backbone (Gly-Phe-Gly) linked to a semicarbazone group.
- Exhibits both hydrophilic (glycine, semicarbazone) and hydrophobic (phenylalanine) regions, enabling interactions with diverse biological targets .
4-Substituted Chlorobenzaldehyde Semicarbazones
- Simpler aromatic aldehydes with chlorine substituents (e.g., o-chloro-, p-chloro-, or 2,6-dichlorobenzaldehyde derivatives).
- Lack peptide chains, relying on halogen and semicarbazone groups for bioactivity .
Thiosemicarbazones
- Replace the semicarbazone oxygen with sulfur (C=S instead of C=O).
- Exhibit enhanced metal-binding capacity and altered electronic properties compared to semicarbazones .
Salicylaldehyde Semicarbazones
- Feature a hydroxyl group adjacent to the aldehyde, enabling tridentate coordination with metals (O, N, O).
- Example: [VO(L)(phen)] complexes with antileishmanial activity .
Key Structural Differences
Compound Type | Backbone Structure | Functional Groups | Metal-Binding Sites |
---|---|---|---|
H-Gly-Phe-Gly-aldehyde SCZ | Tripeptide + semicarbazone | Amide, semicarbazone, aromatic | N, O (peptide and SCZ moieties) |
4-Chlorobenzaldehyde SCZ | Aromatic aldehyde | Cl, semicarbazone | N, O (SCZ only) |
Thiosemicarbazones | Variable aldehydes/ketones | C=S, semicarbazide | N, S |
Salicylaldehyde SCZ | Hydroxy-substituted aromatic | OH, semicarbazone | O, N, O (tridentate) |
This compound
- Synthesis involves multi-step peptide coupling followed by semicarbazone formation. No explicit details are provided in the evidence, but analogous methods for peptide-aldehyde semicarbazones require controlled pH and temperature to avoid racemization .
Physicochemical and Electronic Properties
Solubility
- H-Gly-Phe-Gly-aldehyde SCZ: High solubility in DMSO and polar solvents due to peptide and semicarbazone groups .
- 4-Chlorobenzaldehyde SCZ: Lower solubility, requiring organic solvents for formulation .
Tautomerism
- Semicarbazones exist in keto-enol tautomeric forms, influencing charge distribution and reactivity. H-Gly-Phe-Gly-aldehyde SCZ’s peptide backbone may stabilize the keto form .
Semiconductivity
H-Gly-Phe-Gly-aldehyde SCZ
- Potential to form peptide-metal complexes, though specific studies are lacking. The tripeptide backbone could enable multidentate coordination .
Salicylaldehyde SCZ-Vanadium Complexes
Thiosemicarbazone-Copper Complexes
- Enhanced anticancer activity via ROS generation and topoisomerase inhibition .
Biological Activity
H-Gly-Phe-Gly-aldehyde semicarbazone is a compound of significant interest in biochemical research due to its biological activity, particularly its role as an enzyme inhibitor. This article explores the biological properties, mechanisms of action, and potential applications of this compound based on diverse research findings.
Chemical Structure and Properties
This compound is characterized by the presence of a semicarbazone functional group linked to a peptide sequence consisting of glycine (Gly), phenylalanine (Phe), and another glycine (Gly). Its molecular formula is C₁₄H₁₈N₄O₂, with a molecular weight of approximately 278.32 g/mol. The structure can be represented as follows:
Where represents the aldehyde component derived from the peptide sequence.
Mechanism of Biological Activity
The primary biological activity of this compound lies in its ability to act as a reversible inhibitor of cathepsin B, a cysteine protease involved in various physiological processes, including protein degradation and antigen processing. This inhibition has profound implications for cancer biology and parasitology, as cathepsin B is implicated in tumor progression and the life cycles of certain parasites such as Schistosoma japonicum and Plasmodium species .
Enzyme Inhibition Studies
Research indicates that this compound selectively binds to cathepsin B, inhibiting its enzymatic activity. This specificity allows researchers to utilize this compound in biochemical assays to study protease functions. The inhibition mechanism involves the formation of a stable complex between the enzyme and the inhibitor, effectively blocking substrate access to the active site .
Applications in Research
The compound has been employed in various studies focusing on:
- Cancer Research : By inhibiting cathepsin B, this compound can potentially reduce tumor cell invasiveness and metastasis.
- Parasitology : Its efficacy against cathepsin B-like proteinases aids in understanding parasite biology and developing therapeutic strategies against infections.
- Biochemical Assays : The compound serves as a valuable tool for investigating protease functions and interactions within biological systems.
Comparative Analysis with Similar Compounds
The table below compares this compound with structurally similar compounds:
Compound Name | Molecular Formula | Molecular Weight |
---|---|---|
This compound | C₁₄H₁₈N₄O₂ | 278.32 g/mol |
Z-Gly-Phe-Gly-Aldehyde semicarbazone | C₂₂H₂₆N₆O₅ | 454.48 g/mol |
Glycyl-Phenylalanylglycine-semicarbazone | C₁₄H₂₀N₆O₃ | 320.35 g/mol |
N-(2-(Aminocarbonyl)hydrazinylidene)glycine | C₁₅H₂₂N₆O₄ | 336.35 g/mol |
This comparison highlights the unique inhibitory action of this compound on cathepsin B, which is not universally shared among similar compounds .
Case Studies
Several studies have documented the effects of this compound:
- Inhibition of Tumor Growth : A study demonstrated that treatment with this compound led to reduced growth rates in tumor cell lines, correlating with decreased cathepsin B activity.
- Parasitic Infection Models : Research involving Schistosoma japonicum showed that this compound effectively inhibited the activity of cathepsin B-like enzymes, leading to reduced viability of the parasites.
Q & A
Q. How can researchers optimize the synthesis of H-Gly-Phe-Gly-aldehyde semicarbazone to ensure high purity and yield?
Basic Methodological Guidance :
The synthesis involves a condensation reaction between H-Gly-Phe-Gly-aldehyde and semicarbazide under acidic or basic catalysis. Key parameters include pH control (optimal range: 3–5), stoichiometric ratios (1:1 aldehyde-to-semicarbazide), and solvent selection (e.g., ethanol/water mixtures). Sodium acetate is often added to buffer the reaction medium, enhancing nucleophilic attack by stabilizing the semicarbazide . Post-synthesis, recrystallization from methanol or ethanol improves purity. Monitor reaction completion via TLC (silica gel, mobile phase: chloroform/methanol 9:1) or HPLC (C18 column, UV detection at 254 nm) .
Q. What spectroscopic techniques are most effective for characterizing semicarbazone derivatives like this compound?
Basic Methodological Guidance :
- NMR : Use - and -NMR to confirm imine bond formation (C=N peak at ~160 ppm in -NMR) and analyze peptide backbone integrity. Aromatic protons from phenylalanine residues appear at δ 7.2–7.4 ppm .
- IR : Detect C=O (amide I band, ~1650 cm) and N-H stretches (~3300 cm). The absence of aldehyde C=O (~1720 cm) confirms successful condensation .
- Mass Spectrometry : ESI-MS or MALDI-TOF can verify molecular weight (e.g., [M+H] for this compound) .
Q. How can conflicting crystallographic and spectroscopic data on semicarbazone conformations be resolved?
Advanced Research Consideration :
Discrepancies may arise from differences in solid-state (X-ray) vs. solution-phase (NMR) structures. For example, X-ray diffraction of salicylaldehyde semicarbazone revealed an anti conformation between the semicarbazone O and hydrazinic N atoms, stabilized by intramolecular H-bonds . In solution, dynamic equilibrium between syn and anti conformers may occur. To resolve contradictions:
- Perform variable-temperature NMR to detect conformational flexibility.
- Compare computational models (DFT) with experimental data to identify dominant conformers .
Q. What strategies are recommended for designing pharmacophore models based on semicarbazone derivatives?
Advanced Research Consideration :
Pharmacophore development requires:
- Structural Modifications : Introduce substituents at key positions (e.g., halogenated aryl rings at site A or extended alkyl chains at site C) to enhance binding to biological targets like viral envelope proteins or ion channels .
- Bioactivity Correlation : Use QSAR models to link electronic (HOMO/LUMO) and steric parameters (logP) with observed activities (e.g., antifungal MIC values) .
- Validation : Test lead compounds in in vitro assays (e.g., antifungal activity against Candida albicans) and validate binding modes via molecular docking .
Q. How do metal coordination properties of semicarbazones influence their bioactivity?
Advanced Research Consideration :
Semicarbazones act as tridentate ligands (NNO donors) in metal complexes. For example:
- Copper(II) complexes exhibit enhanced antifungal activity due to redox-active metal centers disrupting microbial membranes .
- Iron(III) complexes with salicylaldehyde semicarbazone show spin-crossover behavior, which can be exploited in targeted drug delivery systems .
Characterize complexes via cyclic voltammetry (redox potentials) and EPR spectroscopy (metal-ligand bonding) .
Q. What analytical challenges arise in quantifying trace aldehyde semicarbazones in biological matrices?
Advanced Methodological Guidance :
- Sample Preparation : Derivatize aldehydes with semicarbazide to stabilize reactive intermediates. Use hydrogenation (PtO/MeOH) to reduce unsaturated aldehydes for uniform detection .
- LC-MS/MS : Employ MRM transitions (e.g., m/z 296→279 for hexadecenal semicarbazone) with optimized collision energies (23–33 eV) and a C18 column for separation. Include isotopic internal standards (e.g., d5-hexadecenal) to correct for matrix effects .
Q. How can computational methods address discrepancies in semicarbazone stability predictions?
Advanced Research Consideration :
- DFT Calculations : Model π-electron delocalization in the semicarbazone fragment to predict bond lengths and stabilization energies. Compare with X-ray data to refine computational parameters .
- Hirshfeld Surface Analysis : Quantify intermolecular interactions (e.g., H-bonding, π-stacking) in crystal structures to explain stability variations across derivatives .
Q. What experimental controls are critical when evaluating semicarbazone bioactivity?
Basic Methodological Guidance :
- Negative Controls : Include unmodified aldehydes/ketones and semicarbazide alone to rule out nonspecific effects.
- Stability Controls : Monitor semicarbazone degradation in assay buffers (e.g., PBS, pH 7.4) via HPLC to ensure compound integrity during testing .
Properties
IUPAC Name |
(2S)-N-(2-aminoacetyl)-2-[[(2E)-2-(carbamoylhydrazinylidene)ethyl]amino]-3-phenylpropanamide | |
---|---|---|
Source | PubChem | |
URL | https://pubchem.ncbi.nlm.nih.gov | |
Description | Data deposited in or computed by PubChem | |
InChI |
InChI=1S/C14H20N6O3/c15-9-12(21)19-13(22)11(8-10-4-2-1-3-5-10)17-6-7-18-20-14(16)23/h1-5,7,11,17H,6,8-9,15H2,(H3,16,20,23)(H,19,21,22)/b18-7+/t11-/m0/s1 | |
Source | PubChem | |
URL | https://pubchem.ncbi.nlm.nih.gov | |
Description | Data deposited in or computed by PubChem | |
InChI Key |
ZNIYCCLYIQNYLQ-TUVHXFJTSA-N | |
Source | PubChem | |
URL | https://pubchem.ncbi.nlm.nih.gov | |
Description | Data deposited in or computed by PubChem | |
Canonical SMILES |
C1=CC=C(C=C1)CC(C(=O)NC(=O)CN)NCC=NNC(=O)N | |
Source | PubChem | |
URL | https://pubchem.ncbi.nlm.nih.gov | |
Description | Data deposited in or computed by PubChem | |
Isomeric SMILES |
C1=CC=C(C=C1)C[C@@H](C(=O)NC(=O)CN)NC/C=N/NC(=O)N | |
Source | PubChem | |
URL | https://pubchem.ncbi.nlm.nih.gov | |
Description | Data deposited in or computed by PubChem | |
Molecular Formula |
C14H20N6O3 | |
Source | PubChem | |
URL | https://pubchem.ncbi.nlm.nih.gov | |
Description | Data deposited in or computed by PubChem | |
Molecular Weight |
320.35 g/mol | |
Source | PubChem | |
URL | https://pubchem.ncbi.nlm.nih.gov | |
Description | Data deposited in or computed by PubChem | |
CAS No. |
102579-48-6 | |
Record name | Glycyl-phenylalanyl-glycine-semicarbazone | |
Source | ChemIDplus | |
URL | https://pubchem.ncbi.nlm.nih.gov/substance/?source=chemidplus&sourceid=0102579486 | |
Description | ChemIDplus is a free, web search system that provides access to the structure and nomenclature authority files used for the identification of chemical substances cited in National Library of Medicine (NLM) databases, including the TOXNET system. | |
Retrosynthesis Analysis
AI-Powered Synthesis Planning: Our tool employs the Template_relevance Pistachio, Template_relevance Bkms_metabolic, Template_relevance Pistachio_ringbreaker, Template_relevance Reaxys, Template_relevance Reaxys_biocatalysis model, leveraging a vast database of chemical reactions to predict feasible synthetic routes.
One-Step Synthesis Focus: Specifically designed for one-step synthesis, it provides concise and direct routes for your target compounds, streamlining the synthesis process.
Accurate Predictions: Utilizing the extensive PISTACHIO, BKMS_METABOLIC, PISTACHIO_RINGBREAKER, REAXYS, REAXYS_BIOCATALYSIS database, our tool offers high-accuracy predictions, reflecting the latest in chemical research and data.
Strategy Settings
Precursor scoring | Relevance Heuristic |
---|---|
Min. plausibility | 0.01 |
Model | Template_relevance |
Template Set | Pistachio/Bkms_metabolic/Pistachio_ringbreaker/Reaxys/Reaxys_biocatalysis |
Top-N result to add to graph | 6 |
Feasible Synthetic Routes
Disclaimer and Information on In-Vitro Research Products
Please be aware that all articles and product information presented on BenchChem are intended solely for informational purposes. The products available for purchase on BenchChem are specifically designed for in-vitro studies, which are conducted outside of living organisms. In-vitro studies, derived from the Latin term "in glass," involve experiments performed in controlled laboratory settings using cells or tissues. It is important to note that these products are not categorized as medicines or drugs, and they have not received approval from the FDA for the prevention, treatment, or cure of any medical condition, ailment, or disease. We must emphasize that any form of bodily introduction of these products into humans or animals is strictly prohibited by law. It is essential to adhere to these guidelines to ensure compliance with legal and ethical standards in research and experimentation.