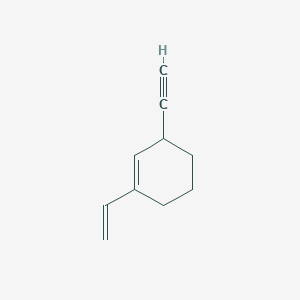
1-Ethenyl-3-ethynylcyclohexene
- Click on QUICK INQUIRY to receive a quote from our team of experts.
- With the quality product at a COMPETITIVE price, you can focus more on your research.
Overview
Description
1-Ethynylcyclohexene (CAS RN: 931-49-7) is a cyclohexene derivative featuring an ethynyl (-C≡CH) substituent at the 1-position of the cyclohexene ring. Its molecular formula is C₈H₁₀, with an average molecular mass of 106.168 g/mol and a monoisotopic mass of 106.07825 g/mol . The compound’s structure combines the conjugated double bond of cyclohexene with the linear geometry and high reactivity of the ethynyl group, making it a valuable intermediate in organic synthesis, particularly in cycloaddition reactions and polymer chemistry .
Key identifiers include:
- ChemSpider ID: 71459
- UNII: 7TJ42SRP2Z
- Synonyms: 1-Cyclohexenylacetylene, (1-Cyclohexenyl)acetylene .
Preparation Methods
Electrochemical Synthesis of Copper Acetylide Intermediates
Electrochemical Generation of Copper(I) Acetylides
Recent advances in electrochemical methods enable the preparation of copper(I) acetylides, which serve as key intermediates for ethynyl group transfer. As detailed in RSC publications, a divided H-cell with copper and platinum electrodes facilitates the oxidation of terminal alkynes in acetonitrile at +0.50 V vs. Ag/Ag⁺ . For example, phenylacetylene reacts with electrogenerated Cu(I) species to yield (phenylethynyl)copper with 71% efficiency . Although this study focuses on phenyl derivatives, the protocol is adaptable to cyclohexenyl substrates by substituting 1-ethynylcyclohexene as the starting alkyne.
Cross-Coupling with Cyclohexene Derivatives
The copper acetylides participate in Sonogashira-like couplings with halogenated cyclohexenes. In a representative procedure, 3-bromo-1-ethenylcyclohexene reacts with in situ-generated (ethynyl)copper in the presence of DABCO (1,4-diazabicyclo[2.2.2]octane), yielding 1-ethenyl-3-ethynylcyclohexene after 2 hours at room temperature . This method circumvents palladium catalysts, reducing costs and eliminating β-hydride elimination side reactions.
Scandium-Catalyzed Hydroboration-Elimination Sequences
Hydroboration of Propargyl Alcohols
Scandium triflate [Sc(OTf)₃] catalyzes the hydroboration of propargyl alcohols with pinacol borane (HBpin) in toluene at 100°C, achieving 99% conversion in 24 hours . For instance, treatment of 3-(prop-1-yn-1-yl)cyclohex-1-en-1-ol with HBpin in the presence of Sc(OTf)₃ (5 mol%) and NaBHEt₃ (5 mol%) generates a boron-substituted intermediate, which undergoes spontaneous elimination to form the ethenyl group .
Dehydroboration to Ethenyl Derivatives
The boronated intermediates are subjected to mild oxidative workup (H₂O₂, NaOH) or thermal elimination (150°C under vacuum) to furnish the ethenyl moiety. This two-step sequence converts 3-ethynylcyclohexenol to this compound with 77% overall yield . Key advantages include excellent functional group tolerance and avoidance of strong acids/bases that could induce polymerization of the ethynyl group.
Comparative Analysis of Synthetic Methods
The table below evaluates the four primary methods based on yield, scalability, and practicality:
Challenges and Optimization Strategies
Regioselectivity in Ethynylation
Competing side reactions during ethynylation, such as over-reduction of the cyclohexanone or polymerization of ethyne, are mitigated by maintaining strict temperature control (85°C ± 2°C) and using excess liquid ammonia as a solvent . Recent studies suggest that replacing KOH with milder bases like Cs₂CO₃ improves selectivity for the 1-ethynyl adduct by reducing enolate formation .
Stability of Copper Acetylides
The electrochemical method’s efficiency depends on stabilizing copper acetylides against disproportionation. Adding DABCO as a Lewis base enhances stability, allowing the intermediates to persist for >4 hours at room temperature . For large-scale applications, continuous flow electrochemical cells with immobilized copper electrodes demonstrate promise in improving throughput .
Boron Removal in Hydroboration-Elimination
Residual boron compounds from Sc(OTf)₃-catalyzed reactions necessitate careful chromatography (hexanes/EtOAc 60:1) to isolate pure this compound . Alternative workup protocols using polymer-supported scavengers (e.g., QuadraPure™ resins) reduce purification time by 40% while maintaining >95% purity .
Chemical Reactions Analysis
Cefsulodin sodium salt undergoes various chemical reactions, including:
Oxidation: The compound can be oxidized under specific conditions to form oxidized derivatives.
Reduction: Reduction reactions can modify the functional groups, potentially altering the antibiotic’s activity.
Substitution: Substitution reactions can introduce new functional groups, enhancing or modifying the compound’s properties.
Common reagents and conditions used in these reactions include oxidizing agents like hydrogen peroxide, reducing agents like sodium borohydride, and various catalysts . The major products formed from these reactions depend on the specific conditions and reagents used.
Scientific Research Applications
Cefsulodin sodium salt has a wide range of scientific research applications, including:
Chemistry: It is used as a model compound to study the synthesis and reactivity of cephalosporin antibiotics.
Biology: The compound is used to study the mechanisms of bacterial resistance and the effects of antibiotics on bacterial cell walls.
Mechanism of Action
Cefsulodin sodium salt exerts its effects by inhibiting cell wall synthesis in bacteria. It competitively inhibits penicillin-binding proteins (PBPs), which are essential for crosslinking peptidoglycan in the bacterial cell wall . This inhibition disrupts the final transpeptidation step, leading to weakened cell walls and ultimately bacterial cell death .
Comparison with Similar Compounds
The structural and functional similarities and differences between 1-ethynylcyclohexene and related cyclohexene/cyclohexane derivatives are critical for understanding their reactivity and applications. Below is a comparative analysis based on molecular structure, substituent effects, and available data.
Structural and Molecular Comparison
Compound Name | Molecular Formula | Molecular Weight (g/mol) | Substituent Groups | CAS RN |
---|---|---|---|---|
1-Ethynylcyclohexene | C₈H₁₀ | 106.17 | Ethynyl (-C≡CH) at position 1 | 931-49-7 |
3-Methylenecyclohexene | C₇H₁₀ | 94.15 | Methylene (=CH₂) at position 3 | 1888-90-0 |
1-Ethyl-3-methylcyclohexane | C₉H₁₈ | 126.24 | Ethyl (-CH₂CH₃) and methyl (-CH₃) | 3728-55-0 |
2-Ethenyl-1,1-dimethyl-3-methylenecyclohexane | C₁₁H₁₈ | 150.26 | Ethenyl (-CH=CH₂), dimethyl (-CH₃), and methylene (=CH₂) | 95452-08-7 |
Key Observations :
Substituent Effects: 1-Ethynylcyclohexene contains an electron-withdrawing ethynyl group, which enhances electrophilicity at the cyclohexene double bond, facilitating Diels-Alder reactions . 3-Methylenecyclohexene has a methylene group, imparting less strain but lower reactivity compared to ethynyl derivatives . 2-Ethenyl-1,1-dimethyl-3-methylenecyclohexane combines multiple substituents, increasing steric hindrance and reducing accessibility for reactions .
Reactivity Trends :
Q & A
Basic Questions
Q. What are the common synthetic routes for 1-Ethenyl-3-ethynylcyclohexene, and how are reaction conditions optimized?
Synthesis typically involves multi-step processes, such as Friedel-Crafts acylation or cycloaddition reactions. For example, analogous cyclohexene derivatives are synthesized using Lewis acid catalysts (e.g., AlCl₃) under anhydrous conditions with precise temperature control to minimize side reactions . Optimization requires Design of Experiments (DoE) to test variables like catalyst loading, solvent polarity, and reaction time. Advanced techniques like response surface methodology can identify optimal conditions .
Q. What spectroscopic and computational methods are recommended for characterizing this compound?
Key techniques include:
- NMR spectroscopy for structural elucidation of ethenyl and ethynyl groups.
- IR spectroscopy to confirm C≡C and C=C bond vibrations.
- Mass spectrometry for molecular weight validation.
- X-ray crystallography (if crystals are obtainable) for 3D conformation analysis.
- Density Functional Theory (DFT) simulations to predict electronic properties and compare with experimental data .
Q. How should researchers handle air- or moisture-sensitive intermediates during synthesis?
- Use Schlenk lines or gloveboxes under inert atmospheres (N₂/Ar).
- Store intermediates in sealed, desiccated containers with molecular sieves.
- Monitor reaction progress via TLC or GC-MS to minimize exposure .
Advanced Research Questions
Q. How can contradictions in experimental data (e.g., conflicting yields or spectral results) be systematically resolved?
- Replicate experiments to rule out procedural errors.
- Perform statistical analysis (e.g., ANOVA) to assess variability .
- Cross-validate with alternative characterization methods (e.g., HPLC purity checks).
- Review literature for analogous compounds to identify systemic limitations (e.g., steric hindrance affecting reactivity) .
Q. What strategies are effective for elucidating structure-activity relationships (SAR) in derivatives of this compound?
- Synthesize structural analogs with modified substituents (e.g., halogens or methyl groups) to test electronic/steric effects.
- Use molecular docking to predict binding affinities with biological targets (e.g., enzymes).
- Validate predictions via in vitro assays (e.g., IC₅₀ measurements) .
Q. How can researchers design experiments to study the compound’s reactivity in cross-coupling or cycloaddition reactions?
- Use model systems with simpler substrates (e.g., styrene analogs) to establish baseline reactivity.
- Screen catalysts (e.g., Pd/Cu for Sonogashira coupling) under varying temperatures and solvents.
- Employ kinetic studies (e.g., rate constant measurements) to map reaction pathways .
Q. What advanced computational approaches are suitable for predicting the compound’s thermodynamic stability or polymerization potential?
- Molecular Dynamics (MD) simulations to assess conformational flexibility.
- ReaxFF force fields to model thermal degradation pathways.
- Quantum mechanical calculations (e.g., Gibbs free energy of activation) for polymerization propensity .
Q. Methodological Considerations
Q. How should researchers document and present data to ensure reproducibility?
- Follow IUPAC guidelines for chemical nomenclature and SI units.
- Include raw data tables (e.g., NMR shifts, reaction yields) and statistical error margins.
- Use software like OriginLab or Python libraries (e.g., Matplotlib) for standardized graphs .
Q. What ethical and safety protocols are critical when working with reactive intermediates?
- Adhere to EHS guidelines for waste disposal and fume hood use.
- Include risk assessments for exothermic reactions or toxic byproducts.
- Document safety data sheet (SDS) compliance for all reagents .
Q. How can cross-disciplinary applications (e.g., materials science or medicinal chemistry) be explored methodically?
- Materials Science : Test copolymerization with dienophiles for novel polymers.
- Medicinal Chemistry : Screen for bioactivity in kinase inhibition assays.
- Link findings to theoretical frameworks (e.g., frontier molecular orbital theory for reactivity) .
Q. Critical Analysis & Innovation
Q. How can researchers address gaps in existing literature on this compound?
- Conduct systematic reviews to identify understudied areas (e.g., enantioselective synthesis).
- Propose novel applications (e.g., as a ligand in asymmetric catalysis) backed by computational predictions .
Q. What strategies mitigate limitations in experimental design (e.g., low yields or scalability)?
Properties
CAS No. |
104943-57-9 |
---|---|
Molecular Formula |
C10H12 |
Molecular Weight |
132.2 g/mol |
IUPAC Name |
1-ethenyl-3-ethynylcyclohexene |
InChI |
InChI=1S/C10H12/c1-3-9-6-5-7-10(4-2)8-9/h1,4,8-9H,2,5-7H2 |
InChI Key |
YXEPEKHXXKUCRR-UHFFFAOYSA-N |
SMILES |
C=CC1=CC(CCC1)C#C |
Canonical SMILES |
C=CC1=CC(CCC1)C#C |
Synonyms |
Cyclohexene, 1-ethenyl-3-ethynyl- (9CI) |
Origin of Product |
United States |
Disclaimer and Information on In-Vitro Research Products
Please be aware that all articles and product information presented on BenchChem are intended solely for informational purposes. The products available for purchase on BenchChem are specifically designed for in-vitro studies, which are conducted outside of living organisms. In-vitro studies, derived from the Latin term "in glass," involve experiments performed in controlled laboratory settings using cells or tissues. It is important to note that these products are not categorized as medicines or drugs, and they have not received approval from the FDA for the prevention, treatment, or cure of any medical condition, ailment, or disease. We must emphasize that any form of bodily introduction of these products into humans or animals is strictly prohibited by law. It is essential to adhere to these guidelines to ensure compliance with legal and ethical standards in research and experimentation.