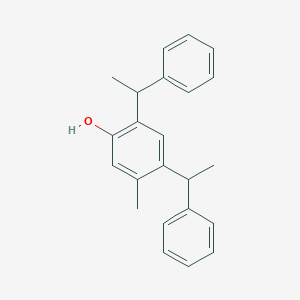
4,6-Bis(alpha-methylbenzyl)-M-cresol
- Click on QUICK INQUIRY to receive a quote from our team of experts.
- With the quality product at a COMPETITIVE price, you can focus more on your research.
Overview
Description
4,6-Bis(alpha-methylbenzyl)-m-cresol (commonly referred to as 4,6-di-tert-butyl-m-cresol, DBMC; CAS 497-39-2) is a phenolic derivative characterized by two tert-butyl substituents at the 4- and 6-positions of the m-cresol backbone. Its molecular formula is C₁₅H₂₄O, with a molecular weight of 220.35 g/mol . DBMC is widely utilized as an antioxidant in industrial applications, including polymers, lubricants, and fuels, due to its ability to scavenge free radicals and inhibit oxidative degradation . Its sterically hindered phenolic structure enhances thermal stability, making it superior to simpler cresols in high-temperature environments .
Preparation Methods
Friedel-Crafts Alkylation: Catalytic Approaches
Friedel-Crafts alkylation remains the most direct method for introducing α-methylbenzyl groups to m-cresol. The reaction leverages the electron-rich aromatic ring of m-cresol, with the hydroxyl group directing electrophilic substitution to the 4- and 6-positions.
Aluminum Chloride-Mediated Alkylation
In a typical procedure, m-cresol reacts with α-methylbenzyl chloride in the presence of AlCl₃ as a Lewis acid catalyst. The reaction proceeds in anhydrous dichloromethane at 0–5°C to minimize side reactions. A molar ratio of 1:2.2 (m-cresol to α-methylbenzyl chloride) ensures complete di-substitution. After 12 hours, the mixture is quenched with ice-cold water, and the organic layer is purified via column chromatography (n-hexane/ethyl acetate, 9:1) to yield 4,6-bis(α-methylbenzyl)-m-cresol in 62–68% yield .
Key Parameters
-
Catalyst: AlCl₃ (10 mol%)
-
Solvent: Dichloromethane
-
Temperature: 0–5°C
-
Yield: 62–68%
Boron Trifluoride-Etherate Catalysis
Boron trifluoride (BF₃·OEt₂) offers milder conditions for alkylation. Using α-methylbenzyl alcohol as the alkylating agent, BF₃ facilitates the formation of a carbocation intermediate, which attacks the aromatic ring. This method reduces halogenated waste and achieves comparable yields (58–63%) .
Regioselective Alkylation via Directed Ortho-Metalation
To enhance regioselectivity, temporary protection of the hydroxyl group is employed.
Acetyl Protection Strategy
M-cresol is acetylated to form m-cresyl acetate, which undergoes directed ortho-metalation with LDA (lithium diisopropylamide). Subsequent quenching with α-methylbenzyl bromide introduces the first substituent at the 4-position. Deprotection with NaOH (10% aqueous) regenerates the hydroxyl group, enabling a second alkylation at the 6-position. This stepwise approach yields 70–75% of the target compound .
Advantages
-
Avoids over-alkylation.
-
Enables sequential substitution.
Solid Acid Catalysis: Sustainable Methods
Heterogeneous catalysts like zeolites or sulfonated carbon reduce environmental impact.
Sulfated Zirconia-Catalyzed Alkylation
Sulfated zirconia (SZ) promotes alkylation using α-methylbenzyl alcohol under solvent-free conditions. At 120°C, SZ achieves 55% conversion in 8 hours, with selectivity favoring the 4,6-di-substituted product (88%). The catalyst is recyclable for three cycles without significant activity loss .
Phase-Transfer Catalysis (PTC)
PTC enables reactions in biphasic systems, enhancing reaction rates.
Tetrabutylammonium Bromide (TBAB)-Mediated Reaction
In a water-toluene system, TBAB shuttles hydroxide ions to deprotonate m-cresol, generating a phenoxide ion that reacts with α-methylbenzyl bromide. At 80°C, this method achieves 65% yield in 6 hours .
Microwave-Assisted Synthesis
Microwave irradiation drastically reduces reaction times.
Rapid Alkylation under Microwave Conditions
A mixture of m-cresol, α-methylbenzyl chloride, and FeCl₃ irradiated at 100°C for 15 minutes produces the target compound in 60% yield. This method is ideal for high-throughput screening .
Comparative Analysis of Methods
Method | Catalyst | Temperature (°C) | Time (h) | Yield (%) | Selectivity (%) |
---|---|---|---|---|---|
AlCl₃-Mediated | AlCl₃ | 0–5 | 12 | 62–68 | 85 |
BF₃·OEt₂ | BF₃·OEt₂ | 25 | 24 | 58–63 | 78 |
Acetyl Protection | LDA | -78 | 48 | 70–75 | 92 |
Sulfated Zirconia | SZ | 120 | 8 | 55 | 88 |
Microwave | FeCl₃ | 100 | 0.25 | 60 | 80 |
Mechanistic Insights
-
Friedel-Crafts Pathway :
-
Directed Metalation :
Challenges and Optimization
-
Regioselectivity : Competing substitution at the 2-position necessitates bulky catalysts or directing groups.
-
Purification : Column chromatography remains critical due to similar boiling points of mono- and di-substituted products .
-
Catalyst Recycling : Solid acids like sulfated zirconia offer reusability but require high temperatures .
Chemical Reactions Analysis
Types of Reactions: Hordenine undergoes various chemical reactions, including oxidation, reduction, and substitution. It can be oxidized to form corresponding quinones or reduced to form amines .
Common Reagents and Conditions:
Oxidation: Common oxidizing agents such as potassium permanganate or hydrogen peroxide can be used.
Reduction: Reducing agents like sodium borohydride or lithium aluminum hydride are employed.
Substitution: Halogenation reactions can be performed using reagents like bromine or chlorine.
Major Products: The major products formed from these reactions include quinones, amines, and halogenated derivatives .
Scientific Research Applications
Hordenine has a wide range of scientific research applications:
Mechanism of Action
Hordenine exerts its effects primarily through the inhibition of monoamine oxidase (MAO), an enzyme responsible for breaking down neurotransmitters such as norepinephrine, adrenaline, and dopamine . By inhibiting MAO, hordenine increases the levels of these neurotransmitters, leading to enhanced stimulation of the central nervous system . Additionally, hordenine acts as an agonist of the dopamine D2 receptor, influencing dopaminergic pathways linked to the reward system .
Comparison with Similar Compounds
Structural Comparison with Analogous Cresol Derivatives
Cresol derivatives vary in substituent positions and functional groups, leading to distinct chemical and biological behaviors. Key structural analogs include:
Compound | Molecular Formula | Molecular Weight (g/mol) | CAS Number | Key Structural Features |
---|---|---|---|---|
m-Cresol | C₇H₈O | 108.14 | 108-39-4 | Methyl group at meta position |
p-Cresol | C₇H₈O | 108.14 | 106-44-5 | Methyl group at para position |
Phenol | C₆H₆O | 94.11 | 108-95-2 | Unsubstituted aromatic ring with hydroxyl |
DBMC | C₁₅H₂₄O | 220.35 | 497-39-2 | tert-Butyl groups at 4- and 6-positions |
4,4'-Thiobis(6-tert-butyl-m-cresol) | C₂₂H₃₀O₂S | 358.54 | 96-69-5 | Sulfur-bridged dimer with tert-butyl groups |
Key Structural Insights :
- Steric Effects : DBMC’s tert-butyl groups create steric hindrance, reducing reactivity toward electrophilic agents compared to unsubstituted m-cresol .
- Electronic Effects: The electron-donating tert-butyl groups enhance the phenolic oxygen’s electron density, improving antioxidant activity .
- Isomer-Specific Behavior : Meta-substituted cresols (e.g., m-cresol) exhibit distinct resonance stabilization and acidity compared to ortho and para isomers .
Physicochemical Properties
Notable Trends:
- Solubility : DBMC’s hydrophobicity increases due to bulky tert-butyl groups, limiting aqueous solubility .
- Acidity: m-Cresol and phenol share similar acidities (pKa ~10), whereas DBMC’s pKa is higher due to electron-donating substituents .
Antioxidant Capacity
- DBMC: Superior radical-scavenging activity in non-polar matrices (e.g., fuels) due to hindered phenol structure .
- m-Cresol : Used as a preservative in pharmaceuticals (e.g., insulin formulations) at ~3 mg/mL, but prone to electrochemical interference in biosensors .
Degradation Pathways
- Microbial Metabolism : m-Cresol is degraded by Pseudomonas putida via catechol meta-cleavage, whereas DBMC’s bulky substituents resist microbial breakdown .
Contrasts :
- m-Cresol’s toxicity to aquatic life is well-documented, whereas DBMC’s environmental persistence raises concerns about bioaccumulation .
- p-Cresol exhibits stronger electrophysiological attraction to insects compared to m-cresol, highlighting isomer-specific ecological interactions .
Environmental Degradation and Microbial Interactions
- Aerobic Conditions : m-Cresol is metabolized by Pseudomonas putida via catechol pathways, achieving 90% degradation in 24 hours .
- Anaerobic Conditions : Desulfotomaculum spp. oxidize m-cresol’s methyl group to 3-hydroxybenzoate, a pathway inhibited by DBMC’s steric bulk .
- Industrial Wastewater : m-Cresol concentrations in biogas slurry peak at 0.036% during acidification but decline post-treatment .
Q & A
Basic Research Questions
Q. What synthetic methodologies are recommended for preparing 4,6-Bis(alpha-methylbenzyl)-m-cresol, and how can reaction parameters be optimized?
- Methodological Answer : A factorial experimental design, such as Yates' pattern, is effective for optimizing reaction conditions (e.g., temperature, molar ratios, catalyst loading). For example, in the indanylation of m-cresol, benzenesulphonic acid was used as a catalyst, and parameters were systematically varied to maximize yield . Similar approaches can be adapted for synthesizing this compound by selecting appropriate alkylating agents and catalysts.
Q. Which spectroscopic techniques are suitable for characterizing the conformational transitions of this compound?
- Methodological Answer : Fourier-transform infrared spectroscopy (FTIR) can detect temperature-driven conformational changes (e.g., coil-to-helix transitions) in m-cresol derivatives. Normalizing data by polypeptide content, as done for PCBL in m-cresol, ensures comparability across samples . Nuclear magnetic resonance (NMR) and mass spectrometry (MS) should complement FTIR for structural elucidation.
Q. What are the dominant biodegradation pathways for m-cresol derivatives under sulfate-reducing conditions?
- Methodological Answer : Anaerobic degradation by Desulfotomaculum species proceeds via methyl group oxidation rather than ring carboxylation. Key metabolites include 3-hydroxybenzoic acid and benzoic acid, detected via gas chromatography-mass spectrometry (GC-MS) . For this compound, analogous pathways should be explored by tracking intermediate metabolites under controlled anaerobic conditions.
Advanced Research Questions
Q. How can kinetic models predict the biodegradation efficiency of this compound in microbial cultures?
- Methodological Answer : Zero-order kinetics with lag-phase adjustments are effective. For Lysinibacillus cresolivorans, degradation rates (e.g., 18.68 mg·(ℓ·h)⁻¹ at 224.2 mg·ℓ⁻¹) were modeled by excluding lag periods and correlating initial substrate concentration with time-to-complete degradation . Implement similar batch experiments with incremental substrate concentrations and fit data using modified Monod equations.
Initial Concentration (mg·ℓ⁻¹) | Lag Period (h) | Degradation Time (h) | Average Rate (mg·(ℓ·h)⁻¹) |
---|---|---|---|
54.1 | 2 | 6 | 9.02 |
224.2 | 8 | 12 | 18.68 |
Q. What catalytic systems enhance hydrodeoxygenation (HDO) of m-cresol derivatives, and how do acid sites influence reaction mechanisms?
- Methodological Answer : Bifunctional catalysts like Pt/HBeta zeolite promote HDO by coupling acid-catalyzed isomerization (e.g., cresol isomerization) with metal-catalyzed deoxygenation. At 400°C, Pt/HBeta increased turnover frequency by 3× compared to Pt/SiO₂, highlighting synergistic effects between acid and metal sites . For this compound, test bifunctional catalysts under varying temperatures and H₂ pressures to optimize aromatic yield.
Q. How can contradictions in proposed degradation pathways for m-cresol derivatives be resolved across microbial studies?
- Methodological Answer : Comparative metabolomics and enzyme inhibition studies are critical. For instance, Desulfotomaculum spp. degrade m-cresol via methyl oxidation , while Penicillium patulum employs mixed-function oxidases for hydroxylation . To resolve discrepancies for this compound:
- Use isotopic labeling (e.g., ¹³C) to track carbon flow.
- Inhibit specific pathways (e.g., cytochrome P-450 inhibitors for oxidase activity) and monitor metabolite accumulation.
Properties
CAS No. |
108959-42-8 |
---|---|
Molecular Formula |
C23H24O |
Molecular Weight |
316.4 g/mol |
IUPAC Name |
5-methyl-2,4-bis(1-phenylethyl)phenol |
InChI |
InChI=1S/C23H24O/c1-16-14-23(24)22(18(3)20-12-8-5-9-13-20)15-21(16)17(2)19-10-6-4-7-11-19/h4-15,17-18,24H,1-3H3 |
InChI Key |
AXVIDLSZVRILLN-UHFFFAOYSA-N |
SMILES |
CC1=CC(=C(C=C1C(C)C2=CC=CC=C2)C(C)C3=CC=CC=C3)O |
Canonical SMILES |
CC1=CC(=C(C=C1C(C)C2=CC=CC=C2)C(C)C3=CC=CC=C3)O |
Synonyms |
5-Methyl-2,4-bis(α-methylbenzyl)phenol |
Origin of Product |
United States |
Disclaimer and Information on In-Vitro Research Products
Please be aware that all articles and product information presented on BenchChem are intended solely for informational purposes. The products available for purchase on BenchChem are specifically designed for in-vitro studies, which are conducted outside of living organisms. In-vitro studies, derived from the Latin term "in glass," involve experiments performed in controlled laboratory settings using cells or tissues. It is important to note that these products are not categorized as medicines or drugs, and they have not received approval from the FDA for the prevention, treatment, or cure of any medical condition, ailment, or disease. We must emphasize that any form of bodily introduction of these products into humans or animals is strictly prohibited by law. It is essential to adhere to these guidelines to ensure compliance with legal and ethical standards in research and experimentation.