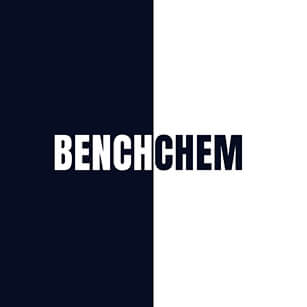
GM3
Overview
Description
GM3, also known as monosialodihexosylganglioside, is a glycosphingolipid composed of a ceramide backbone linked to a sialylated trisaccharide. It is the simplest ganglioside and serves as a precursor for more complex gangliosides. This compound is predominantly found in the outer leaflet of the plasma membrane and plays a crucial role in cell-cell communication, signal transduction, and modulation of cell growth and differentiation .
Preparation Methods
Synthetic Routes and Reaction Conditions: The synthesis of GM3 involves multiple steps, starting with the preparation of suitably protected lactoside diol, which is glycosylated with sialyl xanthate to yield the α-sialyl trisaccharide. This intermediate is then condensed with azidosphingosine using a promoter such as trimethylsilyl trifluoromethanesulfonate (TMSOTf). The resulting glycolipid is reduced with triphenylphosphine and subsequently acylated with octadecanoic acid in the presence of 1-ethyl-3-(3-dimethylaminopropyl)carbodiimide (EDC). Finally, deprotection steps yield the target this compound .
Industrial Production Methods: Industrial production of this compound is challenging due to its complex structure and low natural abundance. Chemical synthesis and chemoenzymatic methods are employed to produce this compound in larger quantities. These methods involve the use of glycosyltransferases and sialyltransferases to achieve regio- and stereoselective glycosylation, followed by purification using techniques such as high-performance liquid chromatography (HPLC) .
Chemical Reactions Analysis
Hydrolysis Reactions
GM3 is susceptible to hydrolysis by sialidases (neuraminidases), which cleave the sialic acid residue from the ganglioside. This reaction can alter the biological properties of this compound and is significant in pathological conditions where sialidase activity is elevated.
- Sialidase Activity : The hydrolysis of this compound by sialidases can lead to the formation of GM2 and other derivatives, impacting cellular signaling pathways and potentially contributing to disease states such as diabetes and neurodegenerative disorders .
Interaction with Proteins
This compound participates in various protein interactions that are crucial for cell signaling. It has been shown to bind specific receptors, modulating their activity and influencing downstream signaling cascades.
- Receptor Binding : Studies have demonstrated that this compound binds to insulin receptors (IR) and other membrane proteins, affecting their conformation and function. For instance, this compound binding can inhibit receptor signaling, leading to reduced cellular proliferation .
Physiological Roles
Research indicates that this compound plays essential roles in regulating cell growth and differentiation. Its presence on cell membranes influences lipid raft formation, which is vital for signal transduction.
- Cell Growth Inhibition : A study found that synthetic this compound mimetics could inhibit the proliferation of certain cell lines by promoting effective clustering within lipid microdomains .
Pathophysiological Implications
Alterations in this compound levels have been linked to various diseases, including diabetes and cancer. Changes in ganglioside composition can affect insulin signaling pathways and contribute to insulin resistance.
- Diabetes Association : In diabetic models, a decrease in this compound levels has been correlated with impaired insulin receptor function, highlighting its role in metabolic regulation .
Therapeutic Potential
The modulation of this compound levels presents potential therapeutic avenues for treating diseases associated with glycosphingolipid dysregulation.
- Drug Development : Research into this compound analogs has shown promise in developing therapies for conditions like nephropathy, where restoring this compound levels may improve podocyte function and reduce proteinuria .
Physiological Effects of this compound Variants
Variant | Biological Activity | Associated Conditions |
---|---|---|
Neu5Ac-GM3 | Promotes cell growth; involved in normal metabolic functions | Diabetes |
Neu5Gc-GM3 | Associated with inflammatory responses | Cancer |
Sialidase-resistant | Enhanced stability; potential therapeutic applications | Chronic diseases |
Scientific Research Applications
GM3 has a wide range of scientific research applications:
Mechanism of Action
GM3 exerts its effects by modulating cell signaling pathways. It interacts with receptor tyrosine kinases (RTKs) and other membrane proteins, influencing signal transduction. This compound can inhibit the autophosphorylation of epidermal growth factor receptor (EGFR), thereby regulating cell proliferation and differentiation. Additionally, this compound plays a role in immune modulation by interacting with immune cells and influencing their activity .
Comparison with Similar Compounds
GM1: A more complex ganglioside with additional sugar residues.
GM2: Contains one less sugar residue compared to GM1.
GD3: A disialoganglioside with two sialic acid residues.
Comparison:
Uniqueness: GM3 is the simplest ganglioside and serves as a precursor for more complex gangliosides. Its simplicity allows for easier synthesis and modification.
Biological Functions: this compound has unique roles in cell signaling and immune modulation, which are distinct from the functions of more complex gangliosides
Biological Activity
Ganglioside GM3, a member of the glycosphingolipid family, plays a significant role in cellular signaling, growth regulation, and various biological processes. Its structure, characterized by the presence of sialic acid, allows it to participate in critical interactions within the cell membrane, influencing numerous cellular functions. This article delves into the biological activity of this compound, highlighting its mechanisms of action, effects on cell proliferation, and its potential therapeutic implications.
Structural Overview
This compound is synthesized from lactosylceramide and is the simplest ganglioside. Its structure includes a ceramide backbone with a sialic acid residue attached to a lactose moiety. This unique configuration enables this compound to form clusters in lipid microdomains, which are essential for effective signal transduction.
1. Cell Proliferation Inhibition
this compound has been shown to inhibit cell proliferation in various cancer cell lines. For instance:
- Inhibition Mechanisms : this compound affects cell cycle regulators such as cyclins and cyclin-dependent kinases (CDKs). Studies indicate that this compound treatment leads to reduced expression of cyclin D1, cyclin E, CDK4, and CDK2, which are crucial for cell cycle progression .
- Cell Type Specificity : The inhibitory effects of this compound vary across different cell types. For example, it significantly inhibits proliferation in Swiss 3T3 fibroblasts and A431 epidermoid carcinoma cells but shows resistance in NIH3T3 fibroblasts and A10 smooth muscle cells .
2. Receptor Modulation
this compound modulates receptor activities by inhibiting autophosphorylation of growth factor receptors:
- EGF Receptor : In A431 and KB cells, this compound inhibits epidermal growth factor (EGF) receptor autophosphorylation, thereby reducing downstream signaling pathways that promote cell growth .
- FGF Receptor : In baby hamster kidney (BHK) cells, this compound suppresses fibroblast growth factor (FGF) receptor internalization .
Biological Activity Evaluations
Recent studies have explored the biological activities of this compound and its derivatives:
Case Studies
1. Neuroprotective Effects
Recent research indicates that this compound and its derivatives may possess neuroprotective properties. In models of neurodegeneration, this compound has been shown to promote neurite outgrowth and protect against neuronal damage .
2. Insulin Resistance
In mouse models with obesity induced by high-fat diets, inhibition of this compound synthesis improved insulin sensitivity and reduced chronic inflammation. This suggests a potential role for this compound modulation in metabolic disorders .
Q & A
Basic Research Questions
Q. What experimental methodologies are commonly used to quantify GM3 in cellular membranes, and how do researchers validate their accuracy?
- Methodological Answer: this compound quantification typically employs high-performance thin-layer chromatography (HPTLC) combined with densitometry or mass spectrometry (LC-MS/MS). Validation involves spiking samples with synthetic this compound standards and cross-referencing with established protocols, such as those described in lipidomics workflows . For reproducibility, statistical measures like intra- and inter-assay coefficients of variation (CV <15%) are recommended .
Q. How do researchers isolate this compound from complex biological samples without degradation?
- Methodological Answer: Extraction protocols use chloroform-methanol (2:1 v/v) with 0.01% butylated hydroxytoluene (BHT) to prevent oxidation. Centrifugation at 100,000×g for 1 hour separates this compound into the organic phase. Purity is confirmed via Western blot using anti-GM3 monoclonal antibodies (e.g., DH2) .
Q. What are the primary challenges in distinguishing this compound from structurally similar gangliosides (e.g., GM1, GM2) in lipidomic analyses?
- Methodological Answer: Structural differentiation requires tandem mass spectrometry (MS/MS) to identify unique fragment ions (e.g., m/z 290 for this compound’s sialic acid moiety). Liquid chromatography (LC) with hydrophilic interaction liquid chromatography (HILIC) columns improves separation resolution .
Advanced Research Questions
Q. How can conflicting data on this compound’s role in cancer metastasis be systematically resolved?
- Methodological Answer: Contradictory findings often arise from model variability (e.g., cell lines vs. in vivo tumors). A meta-analysis framework should include:
- Data Normalization: Adjust for baseline this compound levels across studies using z-scores.
- Cohort Stratification: Group data by cancer type (e.g., melanoma vs. breast cancer) and microenvironmental factors (hypoxia, pH).
- Mechanistic Validation: CRISPR/Cas9 knockout of this compound synthase (ST3GAL5) in isogenic cell lines to assess functional consistency .
Q. What computational models are effective for predicting this compound’s interactions with transmembrane receptors (e.g., EGFR)?
- Methodological Answer: Molecular dynamics (MD) simulations (e.g., GROMACS) with lipid bilayers model this compound-receptor binding. Key parameters include:
- Force Fields: CHARMM36 for glycolipids.
- Simulation Duration: ≥500 ns to capture stable interactions.
- Validation: Compare binding free energies (ΔG) from umbrella sampling with surface plasmon resonance (SPR) data .
Q. How do researchers address reproducibility issues in this compound-dependent signaling studies?
- Methodological Answer: Reproducibility requires:
- Standardized Protocols: Pre-print registration of methods (e.g., on BioProtocol).
- Blinded Analysis: Independent validation of flow cytometry or immunofluorescence data.
- Data Transparency: Raw datasets (e.g., mass spectrometry .RAW files) uploaded to repositories like MetaboLights .
Q. Data Analysis & Interpretation
Q. What statistical approaches are recommended for analyzing this compound’s correlation with disease biomarkers in heterogeneous cohorts?
- Methodological Answer: Use multivariate regression adjusted for covariates (age, BMI). For non-linear relationships, machine learning models (random forests, XGBoost) with SHAP (SHapley Additive exPlanations) values quantify feature importance. Cross-validation (k-fold ≥5) prevents overfitting .
Q. How can multi-omics integration (lipidomics, transcriptomics) elucidate this compound’s regulatory networks?
- Methodological Answer: Joint pathway analysis via tools like MetaboAnalyst 5.0 links this compound levels to gene clusters (e.g., sphingolipid metabolism). Weighted gene co-expression network analysis (WGCNA) identifies hub genes (e.g., UGCG) correlated with this compound abundance .
Q. Experimental Design & Optimization
Q. What controls are essential for in vitro studies of this compound’s immunomodulatory effects?
- Methodological Answer: Include:
- Negative Controls: Lipid-depleted serum or this compound-free liposomes.
- Positive Controls: Lipopolysaccharide (LPS)-treated cells for cytokine baselines.
- Technical Replicates: ≥3 independent experiments with ANOVA for variance assessment .
Q. How do researchers optimize this compound detection in low-abundance samples (e.g., cerebrospinal fluid)?
Properties
IUPAC Name |
sodium;(2S,4S,5R,6R)-5-acetamido-2-[(2S,3R,4S,5S,6R)-2-[(2R,3S,4R,5R,6R)-4,5-dihydroxy-2-(hydroxymethyl)-6-[(E,2S,3R)-3-hydroxy-2-(octadecanoylamino)octadec-4-enoxy]oxan-3-yl]oxy-3,5-dihydroxy-6-(hydroxymethyl)oxan-4-yl]oxy-4-hydroxy-6-[(1R,2R)-1,2,3-trihydroxypropyl]oxane-2-carboxylate | |
---|---|---|
Details | Computed by LexiChem 2.6.6 (PubChem release 2019.06.18) | |
Source | PubChem | |
URL | https://pubchem.ncbi.nlm.nih.gov | |
Description | Data deposited in or computed by PubChem | |
InChI |
InChI=1S/C59H108N2O21.Na/c1-4-6-8-10-12-14-16-18-19-21-23-25-27-29-31-33-46(69)61-40(41(66)32-30-28-26-24-22-20-17-15-13-11-9-7-5-2)38-77-56-51(73)50(72)53(45(37-64)79-56)80-57-52(74)55(49(71)44(36-63)78-57)82-59(58(75)76)34-42(67)47(60-39(3)65)54(81-59)48(70)43(68)35-62;/h30,32,40-45,47-57,62-64,66-68,70-74H,4-29,31,33-38H2,1-3H3,(H,60,65)(H,61,69)(H,75,76);/q;+1/p-1/b32-30+;/t40-,41+,42-,43+,44+,45+,47+,48+,49-,50+,51+,52+,53+,54+,55-,56+,57-,59-;/m0./s1 | |
Details | Computed by InChI 1.0.5 (PubChem release 2019.06.18) | |
Source | PubChem | |
URL | https://pubchem.ncbi.nlm.nih.gov | |
Description | Data deposited in or computed by PubChem | |
InChI Key |
GGQRTSSCXQEZNW-BLVJLEROSA-M | |
Details | Computed by InChI 1.0.5 (PubChem release 2019.06.18) | |
Source | PubChem | |
URL | https://pubchem.ncbi.nlm.nih.gov | |
Description | Data deposited in or computed by PubChem | |
Canonical SMILES |
CCCCCCCCCCCCCCCCCC(=O)NC(COC1C(C(C(C(O1)CO)OC2C(C(C(C(O2)CO)O)OC3(CC(C(C(O3)C(C(CO)O)O)NC(=O)C)O)C(=O)[O-])O)O)O)C(C=CCCCCCCCCCCCCC)O.[Na+] | |
Details | Computed by OEChem 2.1.5 (PubChem release 2019.06.18) | |
Source | PubChem | |
URL | https://pubchem.ncbi.nlm.nih.gov | |
Description | Data deposited in or computed by PubChem | |
Isomeric SMILES |
CCCCCCCCCCCCCCCCCC(=O)N[C@@H](CO[C@H]1[C@@H]([C@H]([C@@H]([C@H](O1)CO)O[C@H]2[C@@H]([C@H]([C@H]([C@H](O2)CO)O)O[C@@]3(C[C@@H]([C@H]([C@@H](O3)[C@@H]([C@@H](CO)O)O)NC(=O)C)O)C(=O)[O-])O)O)O)[C@@H](/C=C/CCCCCCCCCCCCC)O.[Na+] | |
Details | Computed by OEChem 2.1.5 (PubChem release 2019.06.18) | |
Source | PubChem | |
URL | https://pubchem.ncbi.nlm.nih.gov | |
Description | Data deposited in or computed by PubChem | |
Molecular Formula |
C59H107N2NaO21 | |
Details | Computed by PubChem 2.1 (PubChem release 2019.06.18) | |
Source | PubChem | |
URL | https://pubchem.ncbi.nlm.nih.gov | |
Description | Data deposited in or computed by PubChem | |
Molecular Weight |
1203.5 g/mol | |
Details | Computed by PubChem 2.1 (PubChem release 2021.05.07) | |
Source | PubChem | |
URL | https://pubchem.ncbi.nlm.nih.gov | |
Description | Data deposited in or computed by PubChem | |
Disclaimer and Information on In-Vitro Research Products
Please be aware that all articles and product information presented on BenchChem are intended solely for informational purposes. The products available for purchase on BenchChem are specifically designed for in-vitro studies, which are conducted outside of living organisms. In-vitro studies, derived from the Latin term "in glass," involve experiments performed in controlled laboratory settings using cells or tissues. It is important to note that these products are not categorized as medicines or drugs, and they have not received approval from the FDA for the prevention, treatment, or cure of any medical condition, ailment, or disease. We must emphasize that any form of bodily introduction of these products into humans or animals is strictly prohibited by law. It is essential to adhere to these guidelines to ensure compliance with legal and ethical standards in research and experimentation.