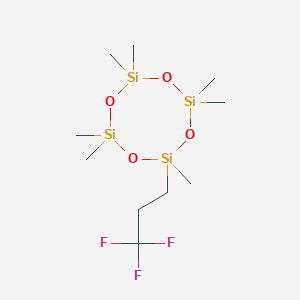
2,2,4,4,6,6,8-Heptamethyl-8-(3,3,3-trifluoropropyl)-1,3,5,7,2,4,6,8-tetroxatetrasilocane
Overview
Description
2,2,4,4,6,6,8-Heptamethyl-8-(3,3,3-trifluoropropyl)-1,3,5,7,2,4,6,8-tetroxatetrasilocane is a useful research compound. Its molecular formula is C10H25F3O4Si4 and its molecular weight is 378.64 g/mol. The purity is usually 95%.
BenchChem offers high-quality this compound suitable for many research applications. Different packaging options are available to accommodate customers' requirements. Please inquire for more information about this compound including the price, delivery time, and more detailed information at info@benchchem.com.
Scientific Research Applications
Nanostructures and Surface Dewettability
A study by Zeng and Zheng (2007) focused on the synthesis of an organic-inorganic amphiphile by capping poly(ethylene oxide) (PEO) with hepta(3,3,3-trifluoropropyl) polyhedral oligomeric silsesquioxane (POSS). The incorporation of this compound into epoxy resin resulted in the formation of nanostructured thermosetting composites. These composites exhibited enhanced surface hydrophobicity and reduced surface free energy, attributed to the enrichment of the POSS moiety on the surface of the nanostructured thermosets, as evidenced by various characterization techniques (Zeng & Zheng, 2007).
Surface Hydrophobicity Enhancement
Another study by Zeng, Wang, and Zheng (2011) described the synthesis of POSS-capped poly(hydroxyether of bisphenol A) (PH) telechelics and their incorporation into epoxy. This resulted in microphase-separated morphologies and significantly enhanced surface hydrophobicity of the epoxy thermosets. The nanostructures were interpreted based on the self-assembly behavior of POSS-capped PH telechelics, with atomic force microscopy (AFM) revealing the distinct morphologies (Zeng, Wang, & Zheng, 2011).
Polymerization Rate Enhancement
Andrianov and Yakushkina (1966) demonstrated that the replacement of a methyl group in octamethylcyclotetrasiloxane by a 3,3,3-trifluoropropyl group led to a significant increase in the polymerization rate. This study highlights the impact of fluorinated groups on the reactivity and polymerization kinetics of siloxane-based compounds (Andrianov & Yakushkina, 1966).
Catalytic Activity and Optical Properties
Araki and Naka (2011) synthesized dumbbell-shaped trifluoropropyl-substituted POSS derivatives linked by aliphatic chains, which upon baking, formed optical transparent films with refractive indices in the range of 1.38 to 1.39. These materials were considered for low-temperature processing applications due to their low refractive indices and high thermal stabilities (Araki & Naka, 2011).
These studies collectively illustrate the versatility and potential of "2,2,4,4,6,6,8-Heptamethyl-8-(3,3,3-trifluoropropyl)-1,3,5,7,2,4,6,8-tetroxatetrasilocane" and its derivatives in enhancing the properties of polymers and composites, contributing to advancements in materials science and engineering.
The scientific research applications of this compound, a compound with potential applications in materials science and chemistry, are diverse and innovative. Here are some key findings from recent research:
Nanostructures and Surface Dewettability
One study focused on the nanostructures and surface dewettability of epoxy thermosets containing a related compound, hepta(3,3,3-trifluoropropyl) polyhedral oligomeric silsesquioxane (POSS)-capped poly(ethylene oxide). The incorporation of POSS-capped polymers into epoxy resin resulted in significant enhancements in surface hydrophobicity and reductions in surface free energy, attributed to the migration of the POSS moiety to the surface of the thermosets. This was evidenced through various characterization techniques, including field emission scanning electronic microscopy (FESEM), transmission electronic microscopy (TEM), and atomic force microscopy (AFM) (Zeng & Zheng, 2007).
Synthesis of Organocyclosiloxanes
Another research explored the synthesis of organocyclosiloxanes with polar substituents, where compounds such as 3-acetoxypropyl)heptamethylcyclotetrasiloxane and heptamethyl[5,5,5-trifluoro-2-(trifluoromethyl)pentyl]cyclotetrasiloxane were synthesized. The study found that replacing one methyl group in octamethylcyclotetrasiloxane with a 3,3,3-trifluoropropyl group significantly increased the rate of polymerization (Andrianov & Yakushkina, 1966).
Synthesis and Characterization of Calcium Beta-Diketonate Complexes
Research on the synthesis and characterization of calcium beta-diketonate complexes with compounds like 2,2,6,6-tetramethyl-3,5-heptanedione provided insights into the formation of mononuclear and dinuclear adducts. The study involved characterizations through elemental analyses, IR, (1)H and (13)C NMR spectroscopies, and single-crystal X-ray diffraction, revealing complex structures and bonding (El Habra et al., 2010).
Properties
IUPAC Name |
2,2,4,4,6,6,8-heptamethyl-8-(3,3,3-trifluoropropyl)-1,3,5,7,2,4,6,8-tetraoxatetrasilocane | |
---|---|---|
Details | Computed by LexiChem 2.6.6 (PubChem release 2019.06.18) | |
Source | PubChem | |
URL | https://pubchem.ncbi.nlm.nih.gov | |
Description | Data deposited in or computed by PubChem | |
InChI |
InChI=1S/C10H25F3O4Si4/c1-18(2)14-19(3,4)16-21(7,9-8-10(11,12)13)17-20(5,6)15-18/h8-9H2,1-7H3 | |
Details | Computed by InChI 1.0.5 (PubChem release 2019.06.18) | |
Source | PubChem | |
URL | https://pubchem.ncbi.nlm.nih.gov | |
Description | Data deposited in or computed by PubChem | |
InChI Key |
KEKBVVIRPIPNQK-UHFFFAOYSA-N | |
Details | Computed by InChI 1.0.5 (PubChem release 2019.06.18) | |
Source | PubChem | |
URL | https://pubchem.ncbi.nlm.nih.gov | |
Description | Data deposited in or computed by PubChem | |
Canonical SMILES |
C[Si]1(O[Si](O[Si](O[Si](O1)(C)C)(C)CCC(F)(F)F)(C)C)C | |
Details | Computed by OEChem 2.1.5 (PubChem release 2019.06.18) | |
Source | PubChem | |
URL | https://pubchem.ncbi.nlm.nih.gov | |
Description | Data deposited in or computed by PubChem | |
Molecular Formula |
C10H25F3O4Si4 | |
Details | Computed by PubChem 2.1 (PubChem release 2019.06.18) | |
Source | PubChem | |
URL | https://pubchem.ncbi.nlm.nih.gov | |
Description | Data deposited in or computed by PubChem | |
DSSTOX Substance ID |
DTXSID70604756 | |
Record name | 2,2,4,4,6,6,8-Heptamethyl-8-(3,3,3-trifluoropropyl)-1,3,5,7,2,4,6,8-tetroxatetrasilocane | |
Source | EPA DSSTox | |
URL | https://comptox.epa.gov/dashboard/DTXSID70604756 | |
Description | DSSTox provides a high quality public chemistry resource for supporting improved predictive toxicology. | |
Molecular Weight |
378.64 g/mol | |
Details | Computed by PubChem 2.1 (PubChem release 2021.05.07) | |
Source | PubChem | |
URL | https://pubchem.ncbi.nlm.nih.gov | |
Description | Data deposited in or computed by PubChem | |
CAS No. |
1744-30-5 | |
Record name | 2,2,4,4,6,6,8-Heptamethyl-8-(3,3,3-trifluoropropyl)-1,3,5,7,2,4,6,8-tetroxatetrasilocane | |
Source | EPA DSSTox | |
URL | https://comptox.epa.gov/dashboard/DTXSID70604756 | |
Description | DSSTox provides a high quality public chemistry resource for supporting improved predictive toxicology. | |
Retrosynthesis Analysis
AI-Powered Synthesis Planning: Our tool employs the Template_relevance Pistachio, Template_relevance Bkms_metabolic, Template_relevance Pistachio_ringbreaker, Template_relevance Reaxys, Template_relevance Reaxys_biocatalysis model, leveraging a vast database of chemical reactions to predict feasible synthetic routes.
One-Step Synthesis Focus: Specifically designed for one-step synthesis, it provides concise and direct routes for your target compounds, streamlining the synthesis process.
Accurate Predictions: Utilizing the extensive PISTACHIO, BKMS_METABOLIC, PISTACHIO_RINGBREAKER, REAXYS, REAXYS_BIOCATALYSIS database, our tool offers high-accuracy predictions, reflecting the latest in chemical research and data.
Strategy Settings
Precursor scoring | Relevance Heuristic |
---|---|
Min. plausibility | 0.01 |
Model | Template_relevance |
Template Set | Pistachio/Bkms_metabolic/Pistachio_ringbreaker/Reaxys/Reaxys_biocatalysis |
Top-N result to add to graph | 6 |
Feasible Synthetic Routes
Disclaimer and Information on In-Vitro Research Products
Please be aware that all articles and product information presented on BenchChem are intended solely for informational purposes. The products available for purchase on BenchChem are specifically designed for in-vitro studies, which are conducted outside of living organisms. In-vitro studies, derived from the Latin term "in glass," involve experiments performed in controlled laboratory settings using cells or tissues. It is important to note that these products are not categorized as medicines or drugs, and they have not received approval from the FDA for the prevention, treatment, or cure of any medical condition, ailment, or disease. We must emphasize that any form of bodily introduction of these products into humans or animals is strictly prohibited by law. It is essential to adhere to these guidelines to ensure compliance with legal and ethical standards in research and experimentation.