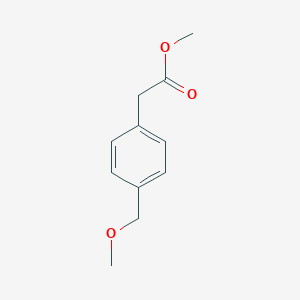
Methyl 4-(methoxymethyl)phenylacetate
- Click on QUICK INQUIRY to receive a quote from our team of experts.
- With the quality product at a COMPETITIVE price, you can focus more on your research.
Overview
Description
Methyl 4-(methoxymethyl)phenylacetate is a chemical compound with the molecular formula C₁₁H₁₄O₃ and a molecular weight of 194.23 . It is a clear liquid at ambient temperature .
Molecular Structure Analysis
The InChI code for Methyl 4-(methoxymethyl)phenylacetate is 1S/C11H14O3/c1-13-8-10-5-3-9(4-6-10)7-11(12)14-2/h3-6H,7-8H2,1-2H3 . This code provides a specific textual representation of the compound’s molecular structure.Physical And Chemical Properties Analysis
Methyl 4-(methoxymethyl)phenylacetate is a clear liquid with a molecular weight of 194.23 . It has a refractive index of n20/D 1.516 (lit.) and a density of 1.135 g/mL at 25 °C (lit.) .Scientific Research Applications
Synthesis and Chemical Behavior
Studies on phenylacetic acid derivatives, including the synthesis of compounds via reactions involving related structures, highlight the chemical versatility and potential for generating various bioactive molecules. For instance, Varma et al. (2006) reported on the production of phenylacetic acid derivatives by Curvularia lunata, indicating the biological pathways that can produce related compounds (Varma et al., 2006).
Quantum mechanical studies, such as those by Mason et al. (2016), on the aldol reaction involving methoxyphenylacetate derivatives underscore the importance of understanding the reaction mechanisms and the stereochemistry of products related to methyl 4-(methoxymethyl)phenylacetate (Mason et al., 2016).
Research on the synthesis of functionalized molecules, for example, Shahvelayati et al. (2017) described the synthesis of complex thiazol-2(3H)-imine derivatives, showcasing methodologies that could be applicable in manipulating the structure of methyl 4-(methoxymethyl)phenylacetate for the development of new chemical entities (Shahvelayati et al., 2017).
Potential Bioactivity and Environmental Impact
Investigations into the environmental behavior and bioactivity of methoxy-functionalized compounds and phenylacetate derivatives provide insights into the potential ecological and pharmaceutical relevance of methyl 4-(methoxymethyl)phenylacetate. For instance, Jørgensen et al. (2012) explored the leaching potential of azoxystrobin and its degradation product, emphasizing the environmental mobility and persistence of related compounds (Jørgensen et al., 2012).
Synthesis and evaluation of novel compounds for bioactivity, as conducted by Guan et al. (2011), who synthesized coumarin derivatives containing methoxyimino phenylacetate, highlight the potential for discovering new bioactive molecules with structures akin to methyl 4-(methoxymethyl)phenylacetate (Guan et al., 2011).
Safety And Hazards
Methyl 4-(methoxymethyl)phenylacetate has been classified with the GHS07 pictogram and has the signal word "Warning" . Hazard statements include H315, H319, and H335, indicating that it may cause skin irritation, serious eye irritation, and may cause respiratory irritation . Precautionary statements include P271, P261, and P280, advising to avoid breathing dust/fume/gas/mist/vapors/spray, and to wear protective gloves/protective clothing/eye protection/face protection .
properties
IUPAC Name |
methyl 2-[4-(methoxymethyl)phenyl]acetate |
Source
|
---|---|---|
Source | PubChem | |
URL | https://pubchem.ncbi.nlm.nih.gov | |
Description | Data deposited in or computed by PubChem | |
InChI |
InChI=1S/C11H14O3/c1-13-8-10-5-3-9(4-6-10)7-11(12)14-2/h3-6H,7-8H2,1-2H3 |
Source
|
Source | PubChem | |
URL | https://pubchem.ncbi.nlm.nih.gov | |
Description | Data deposited in or computed by PubChem | |
InChI Key |
PICKHCWVNHPTSX-UHFFFAOYSA-N |
Source
|
Source | PubChem | |
URL | https://pubchem.ncbi.nlm.nih.gov | |
Description | Data deposited in or computed by PubChem | |
Canonical SMILES |
COCC1=CC=C(C=C1)CC(=O)OC |
Source
|
Source | PubChem | |
URL | https://pubchem.ncbi.nlm.nih.gov | |
Description | Data deposited in or computed by PubChem | |
Molecular Formula |
C11H14O3 |
Source
|
Source | PubChem | |
URL | https://pubchem.ncbi.nlm.nih.gov | |
Description | Data deposited in or computed by PubChem | |
DSSTOX Substance ID |
DTXSID60557540 |
Source
|
Record name | Methyl [4-(methoxymethyl)phenyl]acetate | |
Source | EPA DSSTox | |
URL | https://comptox.epa.gov/dashboard/DTXSID60557540 | |
Description | DSSTox provides a high quality public chemistry resource for supporting improved predictive toxicology. | |
Molecular Weight |
194.23 g/mol |
Source
|
Source | PubChem | |
URL | https://pubchem.ncbi.nlm.nih.gov | |
Description | Data deposited in or computed by PubChem | |
Product Name |
Methyl 4-(methoxymethyl)phenylacetate | |
CAS RN |
17833-56-6 |
Source
|
Record name | Methyl [4-(methoxymethyl)phenyl]acetate | |
Source | EPA DSSTox | |
URL | https://comptox.epa.gov/dashboard/DTXSID60557540 | |
Description | DSSTox provides a high quality public chemistry resource for supporting improved predictive toxicology. | |
Record name | Methyl 4-(methoxymethyl)phenylacetate | |
Source | European Chemicals Agency (ECHA) | |
URL | https://echa.europa.eu/information-on-chemicals | |
Description | The European Chemicals Agency (ECHA) is an agency of the European Union which is the driving force among regulatory authorities in implementing the EU's groundbreaking chemicals legislation for the benefit of human health and the environment as well as for innovation and competitiveness. | |
Explanation | Use of the information, documents and data from the ECHA website is subject to the terms and conditions of this Legal Notice, and subject to other binding limitations provided for under applicable law, the information, documents and data made available on the ECHA website may be reproduced, distributed and/or used, totally or in part, for non-commercial purposes provided that ECHA is acknowledged as the source: "Source: European Chemicals Agency, http://echa.europa.eu/". Such acknowledgement must be included in each copy of the material. ECHA permits and encourages organisations and individuals to create links to the ECHA website under the following cumulative conditions: Links can only be made to webpages that provide a link to the Legal Notice page. | |
Disclaimer and Information on In-Vitro Research Products
Please be aware that all articles and product information presented on BenchChem are intended solely for informational purposes. The products available for purchase on BenchChem are specifically designed for in-vitro studies, which are conducted outside of living organisms. In-vitro studies, derived from the Latin term "in glass," involve experiments performed in controlled laboratory settings using cells or tissues. It is important to note that these products are not categorized as medicines or drugs, and they have not received approval from the FDA for the prevention, treatment, or cure of any medical condition, ailment, or disease. We must emphasize that any form of bodily introduction of these products into humans or animals is strictly prohibited by law. It is essential to adhere to these guidelines to ensure compliance with legal and ethical standards in research and experimentation.