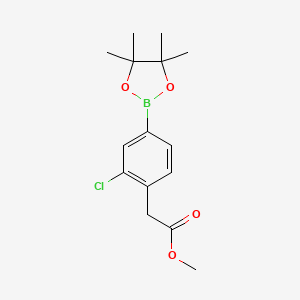
Methyl 2-(2-chloro-4-(4,4,5,5-tetramethyl-1,3,2-dioxaborolan-2-yl)phenyl)acetate
Overview
Description
“Methyl 2-(2-chloro-4-(4,4,5,5-tetramethyl-1,3,2-dioxaborolan-2-yl)phenyl)acetate” is a chemical compound with the formula C15H20BClO4 . It is commonly used in the pharmaceutical and chemical industries .
Synthesis Analysis
The compound can be synthesized through a five-step substitution reaction . The process involves the use of potassium acetate, water, dimethyl sulfoxide, and bis(pinacol)diborane . The yield of this synthesis process is approximately 83% .Molecular Structure Analysis
The molecular structure of the compound was confirmed by MS, 1H NMR, 13C NMR, and FT-IR spectroscopy methods . The crystal structure was determined by X-ray diffraction and conformational analysis . The results show that the crystal structure determined by X-ray single crystal diffraction is consistent with the molecular structure after DFT optimization .Chemical Reactions Analysis
The compound is suitable for various types of reactions including Buchwald-Hartwig Cross Coupling Reaction, Heck Reaction, Hiyama Coupling, Negishi Coupling, Sonogashira Coupling, Stille Coupling, and Suzuki-Miyaura Coupling .Physical and Chemical Properties Analysis
The compound has a number of physical and chemical properties. It has a high GI absorption, is BBB permeant, and is a P-gp substrate . Its water solubility is moderately soluble with a Log S (SILICOS-IT) of -5.08 .Scientific Research Applications
Synthesis and Crystal Structure Analysis
- Methyl 2-(2-chloro-4-(4,4,5,5-tetramethyl-1,3,2-dioxaborolan-2-yl)phenyl)acetate is studied for its synthesis, crystal structure, and density functional theory (DFT) analysis. This compound is obtained through a three-step substitution reaction and its structure is confirmed by various spectroscopy methods and X-ray diffraction. The study provides insights into the molecular structures and physicochemical properties of such compounds (Huang et al., 2021).
Design and Synthesis for Potential HGF-mimetic Agents
- Boron-containing derivatives of this compound are synthesized and evaluated for their potential as Hepatocyte Growth Factor (HGF)-mimetic agents. This involves complex reactions and the biological activities of the synthetic compounds are under evaluation (Das, Tang, & Sanyal, 2011).
Vibrational Properties and Crystallography
- The compound's vibrational properties and crystal structures are studied using spectroscopy and X-ray diffraction. Density functional theory calculations are performed for comparative analysis, providing insights into its vibrational absorption bands and molecular structure (Wu, Chen, Chen, & Zhou, 2021).
Synthesis of Mercapto- and Piperazino-methyl-phenylboronic Acid Derivatives
- The compound is used in the synthesis of derivatives with inhibitory activity against serine proteases, exploring the interaction of these compounds with biological targets. This research highlights the potential pharmaceutical applications of such compounds (Spencer et al., 2002).
Fungicidal Activity Studies
- The compound is used in synthesizing derivatives that exhibit fungicidal activity against certain pathogens. This application is critical in agriculture and plant protection (Liu et al., 2014).
Hydrogen Peroxide Vapor Detection
- It is involved in the synthesis of organic thin-film fluorescence probes for detecting hydrogen peroxide vapor, a crucial component in explosives detection (Fu et al., 2016).
Polyfluorene Nanoparticles for Fluorescence Brightness
- The compound is utilized in the synthesis of polyfluorene nanoparticles, which exhibit bright fluorescence emissions. This application is significant in the field of materials science, particularly for imaging and sensor technologies (Fischer, Baier, & Mecking, 2013).
Cytotoxicity and Cellular Uptake Studies
- Studies involving boronated phosphonium salts containing this compound focus on their cytotoxicity and cellular uptake. This research is crucial in understanding the potential medical applications, especially in cancer therapy (Morrison et al., 2010).
Synthesis and Characterization of Various Derivatives
- Various derivatives of this compound are synthesized and characterized, exploring its potential in different scientific applications, ranging from structural analysis to potential therapeutic uses (Liao, Liu, Wang, & Zhou, 2022).
Herbicidal Activity Analysis
- Its derivatives are analyzed for herbicidal activity, demonstrating its utility in agricultural science and pest management (Zhou, Xue, Wang, & Qu, 2010).
Safety and Hazards
Mechanism of Action
Target of Action
Compounds with similar structures are often used in the phosphitylation of alcohols and heteroatomic nucleophiles .
Mode of Action
It is known that similar compounds are used as reagents for the phosphitylation of alcohols and heteroatomic nucleophiles, resulting in the formation of useful glycosyl donors and ligands .
Biochemical Pathways
It is known that similar compounds are used in various types of coupling reactions, including buchwald-hartwig cross coupling, heck reaction, hiyama coupling, negishi coupling, sonogashira coupling, stille coupling, and suzuki-miyaura coupling .
Pharmacokinetics
It is known that similar compounds have a boiling point of 815-82 °C/13 mmHg and a density of 1149 g/mL at 25 °C .
Action Environment
The action, efficacy, and stability of Methyl 2-(2-chloro-4-(4,4,5,5-tetramethyl-1,3,2-dioxaborolan-2-yl)phenyl)acetate can be influenced by various environmental factors. For instance, it is known that similar compounds should be stored in a locked place . Furthermore, they react violently with water , indicating that they should be handled and stored in an inert atmosphere .
Biochemical Analysis
Biochemical Properties
Methyl 2-(2-chloro-4-(4,4,5,5-tetramethyl-1,3,2-dioxaborolan-2-yl)phenyl)acetate plays a significant role in biochemical reactions, particularly in the context of enzyme interactions. This compound can interact with enzymes such as proteases and kinases, potentially inhibiting their activity through the formation of covalent bonds with active site residues. The boronic ester group in the compound is known to form reversible covalent bonds with serine and threonine residues in the active sites of enzymes, leading to enzyme inhibition . Additionally, this compound can interact with proteins involved in signal transduction pathways, thereby modulating cellular responses.
Cellular Effects
The effects of this compound on various cell types and cellular processes are profound. This compound has been shown to influence cell signaling pathways, particularly those involving kinases and phosphatases. By inhibiting specific kinases, the compound can alter gene expression patterns and cellular metabolism . For instance, the inhibition of kinases involved in the MAPK/ERK pathway can lead to changes in cell proliferation and apoptosis. Furthermore, this compound can affect cellular metabolism by modulating the activity of enzymes involved in metabolic pathways, leading to alterations in metabolite levels and energy production.
Molecular Mechanism
The molecular mechanism of action of this compound involves its interaction with biomolecules at the molecular level. The boronic ester group in the compound can form reversible covalent bonds with nucleophilic residues in proteins, such as serine, threonine, and cysteine . This interaction can lead to the inhibition of enzyme activity by blocking the active site or inducing conformational changes in the enzyme structure. Additionally, the compound can influence gene expression by modulating the activity of transcription factors and other regulatory proteins, leading to changes in the transcriptional landscape of the cell.
Temporal Effects in Laboratory Settings
In laboratory settings, the effects of this compound can change over time. The stability of the compound is a critical factor, as it can undergo hydrolysis or degradation under certain conditions . Studies have shown that the compound remains stable under neutral to slightly acidic conditions but can degrade in highly basic environments. Long-term exposure to the compound can lead to sustained inhibition of enzyme activity and prolonged alterations in cellular function. In vitro and in vivo studies have demonstrated that the compound can have lasting effects on cellular processes, including changes in cell proliferation and apoptosis.
Dosage Effects in Animal Models
The effects of this compound vary with different dosages in animal models. At low doses, the compound can selectively inhibit specific enzymes without causing significant toxicity . At higher doses, the compound can exhibit toxic effects, including hepatotoxicity and nephrotoxicity. Threshold effects have been observed, where a certain dosage is required to achieve a therapeutic effect, but exceeding this dosage can lead to adverse effects. Animal studies have also shown that the compound can affect various physiological processes, including immune responses and metabolic regulation.
Metabolic Pathways
This compound is involved in several metabolic pathways. The compound can be metabolized by enzymes such as cytochrome P450s, leading to the formation of various metabolites . These metabolites can further interact with other enzymes and cofactors, influencing metabolic flux and metabolite levels. The compound can also affect the activity of enzymes involved in glycolysis and the tricarboxylic acid cycle, leading to changes in energy production and metabolic homeostasis.
Transport and Distribution
The transport and distribution of this compound within cells and tissues are mediated by specific transporters and binding proteins . The compound can be taken up by cells through passive diffusion or active transport mechanisms. Once inside the cell, it can bind to intracellular proteins and be distributed to various cellular compartments. The localization and accumulation of the compound can influence its activity and function, as well as its potential toxicity.
Subcellular Localization
The subcellular localization of this compound is crucial for its activity and function. The compound can be targeted to specific cellular compartments through post-translational modifications or targeting signals . For example, the compound can be directed to the nucleus, where it can interact with transcription factors and influence gene expression. Alternatively, it can be localized to the mitochondria, where it can affect metabolic processes and energy production. The subcellular localization of the compound can also determine its potential toxicity, as accumulation in certain organelles can lead to cellular damage.
Properties
IUPAC Name |
methyl 2-[2-chloro-4-(4,4,5,5-tetramethyl-1,3,2-dioxaborolan-2-yl)phenyl]acetate | |
---|---|---|
Source | PubChem | |
URL | https://pubchem.ncbi.nlm.nih.gov | |
Description | Data deposited in or computed by PubChem | |
InChI |
InChI=1S/C15H20BClO4/c1-14(2)15(3,4)21-16(20-14)11-7-6-10(12(17)9-11)8-13(18)19-5/h6-7,9H,8H2,1-5H3 | |
Source | PubChem | |
URL | https://pubchem.ncbi.nlm.nih.gov | |
Description | Data deposited in or computed by PubChem | |
InChI Key |
SGIQMJIVHOIJJX-UHFFFAOYSA-N | |
Source | PubChem | |
URL | https://pubchem.ncbi.nlm.nih.gov | |
Description | Data deposited in or computed by PubChem | |
Canonical SMILES |
B1(OC(C(O1)(C)C)(C)C)C2=CC(=C(C=C2)CC(=O)OC)Cl | |
Source | PubChem | |
URL | https://pubchem.ncbi.nlm.nih.gov | |
Description | Data deposited in or computed by PubChem | |
Molecular Formula |
C15H20BClO4 | |
Source | PubChem | |
URL | https://pubchem.ncbi.nlm.nih.gov | |
Description | Data deposited in or computed by PubChem | |
Molecular Weight |
310.6 g/mol | |
Source | PubChem | |
URL | https://pubchem.ncbi.nlm.nih.gov | |
Description | Data deposited in or computed by PubChem | |
Retrosynthesis Analysis
AI-Powered Synthesis Planning: Our tool employs the Template_relevance Pistachio, Template_relevance Bkms_metabolic, Template_relevance Pistachio_ringbreaker, Template_relevance Reaxys, Template_relevance Reaxys_biocatalysis model, leveraging a vast database of chemical reactions to predict feasible synthetic routes.
One-Step Synthesis Focus: Specifically designed for one-step synthesis, it provides concise and direct routes for your target compounds, streamlining the synthesis process.
Accurate Predictions: Utilizing the extensive PISTACHIO, BKMS_METABOLIC, PISTACHIO_RINGBREAKER, REAXYS, REAXYS_BIOCATALYSIS database, our tool offers high-accuracy predictions, reflecting the latest in chemical research and data.
Strategy Settings
Precursor scoring | Relevance Heuristic |
---|---|
Min. plausibility | 0.01 |
Model | Template_relevance |
Template Set | Pistachio/Bkms_metabolic/Pistachio_ringbreaker/Reaxys/Reaxys_biocatalysis |
Top-N result to add to graph | 6 |
Feasible Synthetic Routes
Disclaimer and Information on In-Vitro Research Products
Please be aware that all articles and product information presented on BenchChem are intended solely for informational purposes. The products available for purchase on BenchChem are specifically designed for in-vitro studies, which are conducted outside of living organisms. In-vitro studies, derived from the Latin term "in glass," involve experiments performed in controlled laboratory settings using cells or tissues. It is important to note that these products are not categorized as medicines or drugs, and they have not received approval from the FDA for the prevention, treatment, or cure of any medical condition, ailment, or disease. We must emphasize that any form of bodily introduction of these products into humans or animals is strictly prohibited by law. It is essential to adhere to these guidelines to ensure compliance with legal and ethical standards in research and experimentation.