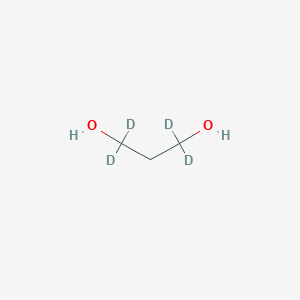
Ethylene-d4 glycol
Overview
Description
Ethylene-d4 glycol is a deuterated form of ethylene glycol . It is an organic compound that consists of two deuterium atoms replacing two hydrogen atoms in the ethylene glycol molecule . This deuterium labeling provides unique properties that make it valuable in various scientific applications .
Synthesis Analysis
Ethylene-d4 glycol serves as a versatile solvent and a starting material for organic syntheses . The deuterium labeling offered by ethylene-d4 glycol enables the tracking and identification of molecules in complex systems, allowing researchers to study reaction mechanisms, kinetics, and the behavior of molecules in biological systems .Molecular Structure Analysis
The molecular structure of Ethylene-d4 glycol consists of two deuterium atoms replacing two hydrogen atoms in the ethylene glycol molecule . This deuterium labeling provides unique properties that make it valuable in various scientific applications .Chemical Reactions Analysis
In terms of its mechanism of action, ethylene-d4 glycol shares similar properties and reactivity with ethylene glycol . It acts as a diol, featuring two hydroxyl groups, which allows it to participate in various chemical reactions . It can undergo esterification, ether formation, and oxidation reactions, among others .Physical And Chemical Properties Analysis
Ethylene-d4 glycol has a molecular weight of 66.09 . It is a liquid at room temperature . The refractive index is 1.428 (lit.) . The boiling point is 196-198 °C (lit.) and the density is 1.184 g/mL at 25 °C .Scientific Research Applications
Chemical Synthesis and Industrial Applications Ethylene glycol (EG) is a significant organic compound serving as a chemical intermediate in various industrial processes such as energy production, plastics, automobiles, and chemicals. The unique properties of EG have led to its exploration in numerous chemical systems for synthesis, primarily through fossil fuels and biomass-based resources. This review highlights the broad spectrum of EG's properties, synthesis advancements, and commercial applications, emphasizing catalytic reactivity and reaction mechanisms. It also addresses the challenges and future research opportunities associated with EG (Yue et al., 2012).
Solvation Properties and Intermolecular Interactions The thermochemical properties of EG as a solvent were extensively examined through solution calorimetry. The study measured the enthalpies of solution for 28 organic solutes in EG, marking the first time for such measurements. The research utilized Acree and Abraham multi-parameter correlations to describe the solvation enthalpies of various compounds in EG, including inert gases, ketones, esters, ethers, nitriles, alcohols, and amines. The study revealed linear correlations between the solvation enthalpies in EG and those in methanol, indicating the significance of hydrogen bonding interactions (Stolov et al., 2017).
Conformation and Hydrogen Bonding under Pressure In a study focusing on the behavior of EG under pressure, in situ high-pressure Raman and infrared absorption spectroscopy were used to investigate the pressure-induced variations in conformations and hydrogen bonding interactions of EG up to 10 GPa. The research indicated that EG remains liquid with a mixture of trans and gauche conformations up to 3.1 GPa. The study also noted the formation of new hydrogen bonding networks prior to the liquid-solid transition, which stabilizes the gauche conformation at pressures above 5 GPa (Murli et al., 2012).
Biotechnological and Environmental Applications Ethylene glycol is used in various industrial applications such as in the production of polyethylene terephthalate, antifreeze, and gas hydrate inhibitors. The study engineered Pseudomonas putida KT2440 for efficient EG metabolism via aerobic microbial processes. The research aimed to expand the metabolic portfolio of P. putida by elucidating the metabolic pathway for EG metabolism. The study provides a robust P. putida KT2440 strain for EG consumption, serving as a foundational strain for further biocatalyst development for applications in waste polyester plastics remediation and biomass-derived wastewater stream treatment (Franden et al., 2018).
Safety And Hazards
Ethylene-d4 glycol is harmful if swallowed . It may cause damage to organs (Kidney) through prolonged or repeated exposure if swallowed . It is advised to avoid breathing mist, gas or vapors, and avoid contacting with skin and eye . Use personal protective equipment and wear chemical impermeable gloves . Ensure adequate ventilation and remove all sources of ignition .
properties
IUPAC Name |
1,1,2,2-tetradeuterioethane-1,2-diol | |
---|---|---|
Source | PubChem | |
URL | https://pubchem.ncbi.nlm.nih.gov | |
Description | Data deposited in or computed by PubChem | |
InChI |
InChI=1S/C2H6O2/c3-1-2-4/h3-4H,1-2H2/i1D2,2D2 | |
Source | PubChem | |
URL | https://pubchem.ncbi.nlm.nih.gov | |
Description | Data deposited in or computed by PubChem | |
InChI Key |
LYCAIKOWRPUZTN-LNLMKGTHSA-N | |
Source | PubChem | |
URL | https://pubchem.ncbi.nlm.nih.gov | |
Description | Data deposited in or computed by PubChem | |
Canonical SMILES |
C(CO)O | |
Source | PubChem | |
URL | https://pubchem.ncbi.nlm.nih.gov | |
Description | Data deposited in or computed by PubChem | |
Isomeric SMILES |
[2H]C([2H])(C([2H])([2H])O)O | |
Source | PubChem | |
URL | https://pubchem.ncbi.nlm.nih.gov | |
Description | Data deposited in or computed by PubChem | |
Molecular Formula |
C2H6O2 | |
Source | PubChem | |
URL | https://pubchem.ncbi.nlm.nih.gov | |
Description | Data deposited in or computed by PubChem | |
Molecular Weight |
66.09 g/mol | |
Source | PubChem | |
URL | https://pubchem.ncbi.nlm.nih.gov | |
Description | Data deposited in or computed by PubChem | |
Product Name |
Ethylene-d4 glycol | |
CAS RN |
2219-51-4 | |
Record name | 1,2-Ethane-1,1,2,2-d4-diol | |
Source | CAS Common Chemistry | |
URL | https://commonchemistry.cas.org/detail?cas_rn=2219-51-4 | |
Description | CAS Common Chemistry is an open community resource for accessing chemical information. Nearly 500,000 chemical substances from CAS REGISTRY cover areas of community interest, including common and frequently regulated chemicals, and those relevant to high school and undergraduate chemistry classes. This chemical information, curated by our expert scientists, is provided in alignment with our mission as a division of the American Chemical Society. | |
Explanation | The data from CAS Common Chemistry is provided under a CC-BY-NC 4.0 license, unless otherwise stated. | |
Record name | 1,2-ethane-[2H4]diol | |
Source | European Chemicals Agency (ECHA) | |
URL | https://echa.europa.eu/substance-information/-/substanceinfo/100.017.028 | |
Description | The European Chemicals Agency (ECHA) is an agency of the European Union which is the driving force among regulatory authorities in implementing the EU's groundbreaking chemicals legislation for the benefit of human health and the environment as well as for innovation and competitiveness. | |
Explanation | Use of the information, documents and data from the ECHA website is subject to the terms and conditions of this Legal Notice, and subject to other binding limitations provided for under applicable law, the information, documents and data made available on the ECHA website may be reproduced, distributed and/or used, totally or in part, for non-commercial purposes provided that ECHA is acknowledged as the source: "Source: European Chemicals Agency, http://echa.europa.eu/". Such acknowledgement must be included in each copy of the material. ECHA permits and encourages organisations and individuals to create links to the ECHA website under the following cumulative conditions: Links can only be made to webpages that provide a link to the Legal Notice page. | |
Synthesis routes and methods I
Procedure details
Synthesis routes and methods II
Procedure details
Synthesis routes and methods III
Procedure details
Synthesis routes and methods IV
Procedure details
Synthesis routes and methods V
Procedure details
Retrosynthesis Analysis
AI-Powered Synthesis Planning: Our tool employs the Template_relevance Pistachio, Template_relevance Bkms_metabolic, Template_relevance Pistachio_ringbreaker, Template_relevance Reaxys, Template_relevance Reaxys_biocatalysis model, leveraging a vast database of chemical reactions to predict feasible synthetic routes.
One-Step Synthesis Focus: Specifically designed for one-step synthesis, it provides concise and direct routes for your target compounds, streamlining the synthesis process.
Accurate Predictions: Utilizing the extensive PISTACHIO, BKMS_METABOLIC, PISTACHIO_RINGBREAKER, REAXYS, REAXYS_BIOCATALYSIS database, our tool offers high-accuracy predictions, reflecting the latest in chemical research and data.
Strategy Settings
Precursor scoring | Relevance Heuristic |
---|---|
Min. plausibility | 0.01 |
Model | Template_relevance |
Template Set | Pistachio/Bkms_metabolic/Pistachio_ringbreaker/Reaxys/Reaxys_biocatalysis |
Top-N result to add to graph | 6 |
Feasible Synthetic Routes
Disclaimer and Information on In-Vitro Research Products
Please be aware that all articles and product information presented on BenchChem are intended solely for informational purposes. The products available for purchase on BenchChem are specifically designed for in-vitro studies, which are conducted outside of living organisms. In-vitro studies, derived from the Latin term "in glass," involve experiments performed in controlled laboratory settings using cells or tissues. It is important to note that these products are not categorized as medicines or drugs, and they have not received approval from the FDA for the prevention, treatment, or cure of any medical condition, ailment, or disease. We must emphasize that any form of bodily introduction of these products into humans or animals is strictly prohibited by law. It is essential to adhere to these guidelines to ensure compliance with legal and ethical standards in research and experimentation.