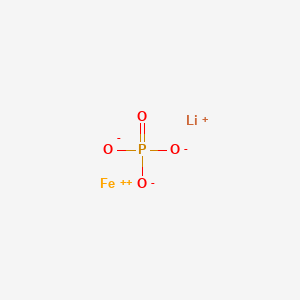
Lithium iron phosphate
Overview
Description
Lithium iron phosphate (LiFePO4), a member of the lithium metal phosphate family, has emerged as a significant cathode material for lithium-ion batteries. It is renowned for its stability, safety, and long cycle life, making it a prime candidate for large format applications such as electric and hybrid electric vehicles . The material's intrinsic thermal stability and continual performance improvements have accelerated its adoption in the industry .
Synthesis Analysis
Several synthesis methods have been developed to optimize the properties of LiFePO4. A low-cost aqueous precipitation technique has been employed to create phase pure, homogeneous, and well-crystallized LiFePO4 by co-precipitating an Fe(II) precursor and subsequent heat treatment in nitrogen . Hydrothermal methods have also been successful, with LiFePO4 being synthesized in a glucose solution, leading to carbon-coated particles that exhibit excellent electrochemical performance . Additionally, spray-pyrolysis followed by sintering has been used to synthesize Mg-doped LiFePO4, which significantly improves electronic conductivity . Supercritical hydrothermal synthesis has been highlighted as a potential industrial-scale method due to its ability to control key parameters like temperature and pressure .
Molecular Structure Analysis
The molecular structure of LiFePO4 has been extensively studied. It is known to crystallize in the olivine structure, which is conducive to the stability and electrochemical performance of the material . The FTIR spectroscopy has been used to probe the local environment in LiFePO4, distinguishing between different phases and types of cationic environments . The structure of novel lithium iron phosphates like LiFe2P3O10 has been determined, showing that it crystallizes in the monoclinic system .
Chemical Reactions Analysis
LiFePO4 undergoes electrochemical reactions during battery operation. The material's electrochemical properties have been systematically measured, showing that Mg-doping can drastically improve its performance . The electrochemical oxidation of lithium iron thio-phosphate, a related material, has shown a potential plateau at around 3.0 V, which is the highest among the sulfides examined .
Physical and Chemical Properties Analysis
The physical and electrochemical properties of LiFePO4 are influenced by the synthesis method. For instance, the sol-gel method has been used to create a porous structure with a carbon layer, which enhances the electrochemical performance . The hydrothermal synthesis of LiFePO4 has resulted in crystalline material with no impurities, which is comparable to those formed by conventional high-temperature synthesis . The electrochemical performance of LiFePO4 has been enhanced by in situ carbon coating, leading to high discharge capacities and excellent cycling stability .
Scientific Research Applications
Atomic Layer Deposition in Metal Phosphates Lithium iron phosphate (LFP) has gained prominence as an electrode material for lithium-ion batteries (LIBs) due to its excellent thermal and structural stability. However, its applications extend beyond LIBs, including use in electrocatalytic water splitting, biocompatible coatings, and protective coatings for luminescent materials. Atomic Layer Deposition (ALD) emerges as a significant technique for depositing metal phosphates, offering unique capabilities like high thickness and compositional control (Henderick et al., 2022).
High-Power Lithium-Ion Cells for Low-Voltage Applications LFP is ideal for vehicle electrical systems due to its superior cycle stability, compactness, and weight advantages over conventional batteries. It demonstrates exceptional performance in 12V applications and stands out for its constant power output and energy efficiency (Bank et al., 2021).
Enhancing Electrical Conductivity LFP's initially poor electronic conductivity can be improved significantly through thermal nanocrystallization. This enhancement is crucial for its application as cathodes in Li-ion rechargeable batteries (Pietrzak et al., 2011).
Carbon-Nanotube-Decorated LFP for LIBs Carbon-nanotube-decorated nano-LFP cathode materials show superior high-rate and low-temperature performances in LIBs, essential for electric vehicles and stationary energy storage for wind and solar energy (Wu et al., 2013).
Polyanion Glasses as Battery Cathode Materials LFP's success as a lithium-ion battery cathode material has led to the exploration of other crystalline polyanionic materials. However, challenges like low electrical conductivity and irreversible phase changes during cycling need addressing (Kercher et al., 2013).
Evolution from Research to Industrial Applications The journey of LFP from fundamental research to commercialization provides valuable insights for improving LFP batteries and designing next-generation batteries (Li & Ma, 2019).
Two-Dimensional Lithium Diffusion in LFP Studies on single-crystal lithium iron phosphate microrods reveal two-dimensional lithium diffusivity, offering insights into phase transformation behaviors and guiding better electrode design (Hong et al., 2017).
Synthesis and Performance Improvement Methods Various methods for preparing LFP are explored, including solid-state and solution-based synthesis routes. Improving its electrochemical performance remains a critical focus (Satyavani et al., 2016).
LFP in Electric Vehicles and Hybrid Electric Vehicles Experimental analysis under different operating conditions highlights LFP's potential application in electric and hybrid electric vehicles, focusing on its dynamic load variation and storage process efficiency (Marongiu et al., 2010).
Energy Storage in Power Grid Management LFP energy storage shows potential benefits in micro-source systems due to low internal resistance and longer service life compared to acid batteries, crucial in power grid stabilization and energy surplus absorption (Kopiczko & Jaworski, 2021).
Improved Fabrication of LFP Cathode Materials Exploration of cold sintering process, microwave sintering, and hydrothermal synthesis for LFP cathode materials opens avenues for enhancing preparation processes and industrialization prospects (An & Li, 2022).
Improving Tap Density of LFP Research focuses on improving LFP's low volumetric energy density by enhancing its tap density through the preparation of spherical particles, balancing high tap density with good electrochemical properties (Xi-xi et al., 2010).
Phase-Transforming Electrodes Structural studies of LFP provide insights into its high performance in high-rate discharge-charge cycling applications, despite the presence of a miscibility gap and phase transformation challenges (Owen & Hector, 2014).
Laser Structured LFP Composite Electrodes Three-dimensional structuring of LFP composite cathode layers using ultrashort laser radiation improves its capacity retention, cycle stability, and lithium-ion diffusion kinetics (Mangang et al., 2016).
Ultrafast Charging of LFP with Gaseous Oxidants The discovery of a direct gas–solid delithiation reaction in LFP offers a pathway for ultrafast charging under ambient conditions, a significant advancement for lithium-ion batteries (Kuss et al., 2013).
Nanotechnology in LFP Cathodes Reviewing the phase transition mechanism in LFP and the application potential of nanotechnology highlights the challenges and advancements in the high-performance battery sector, particularly the role of nanoscale LFP (Baoquan et al., 2020).
Polymer-Templated LFP/C Nanonetworks LFP nanonetworks, created using a sol-gel based synthesis with block copolymer templating, demonstrate high rate performance and excellent cycle life in lithium-ion batteries (Fischer et al., 2018).
Future Directions
The demand for LFP batteries is expected to grow due to their low cost, high safety, and long cycle life . Research is ongoing to improve the comprehensive performance of LFP cathode materials . There is also interest in developing low-carbon, cost-effective lithium extraction techniques and cathode material regeneration processes .
properties
IUPAC Name |
lithium;iron(2+);phosphate | |
---|---|---|
Source | PubChem | |
URL | https://pubchem.ncbi.nlm.nih.gov | |
Description | Data deposited in or computed by PubChem | |
InChI |
InChI=1S/Fe.Li.H3O4P/c;;1-5(2,3)4/h;;(H3,1,2,3,4)/q+2;+1;/p-3 | |
Source | PubChem | |
URL | https://pubchem.ncbi.nlm.nih.gov | |
Description | Data deposited in or computed by PubChem | |
InChI Key |
GELKBWJHTRAYNV-UHFFFAOYSA-K | |
Source | PubChem | |
URL | https://pubchem.ncbi.nlm.nih.gov | |
Description | Data deposited in or computed by PubChem | |
Canonical SMILES |
[Li+].[O-]P(=O)([O-])[O-].[Fe+2] | |
Source | PubChem | |
URL | https://pubchem.ncbi.nlm.nih.gov | |
Description | Data deposited in or computed by PubChem | |
Molecular Formula |
FeLiO4P | |
Record name | Lithium iron phosphate | |
Source | Wikipedia | |
URL | https://en.wikipedia.org/wiki/Lithium_iron_phosphate | |
Description | Chemical information link to Wikipedia. | |
Source | PubChem | |
URL | https://pubchem.ncbi.nlm.nih.gov | |
Description | Data deposited in or computed by PubChem | |
DSSTOX Substance ID |
DTXSID20571409 | |
Record name | Iron(2+) lithium phosphate (1/1/1) | |
Source | EPA DSSTox | |
URL | https://comptox.epa.gov/dashboard/DTXSID20571409 | |
Description | DSSTox provides a high quality public chemistry resource for supporting improved predictive toxicology. | |
Molecular Weight |
157.8 g/mol | |
Source | PubChem | |
URL | https://pubchem.ncbi.nlm.nih.gov | |
Description | Data deposited in or computed by PubChem | |
Product Name |
Lithium iron phosphate | |
CAS RN |
15365-14-7 | |
Record name | Phosphoric acid, iron(2+) lithium salt (1:1:1) | |
Source | ChemIDplus | |
URL | https://pubchem.ncbi.nlm.nih.gov/substance/?source=chemidplus&sourceid=0015365147 | |
Description | ChemIDplus is a free, web search system that provides access to the structure and nomenclature authority files used for the identification of chemical substances cited in National Library of Medicine (NLM) databases, including the TOXNET system. | |
Record name | Phosphoric acid, iron(2+) lithium salt (1:1:1) | |
Source | EPA Chemicals under the TSCA | |
URL | https://www.epa.gov/chemicals-under-tsca | |
Description | EPA Chemicals under the Toxic Substances Control Act (TSCA) collection contains information on chemicals and their regulations under TSCA, including non-confidential content from the TSCA Chemical Substance Inventory and Chemical Data Reporting. | |
Record name | Iron(2+) lithium phosphate (1/1/1) | |
Source | EPA DSSTox | |
URL | https://comptox.epa.gov/dashboard/DTXSID20571409 | |
Description | DSSTox provides a high quality public chemistry resource for supporting improved predictive toxicology. | |
Record name | Phosphoric acid, iron(2+) lithium salt (1:1:1) | |
Source | European Chemicals Agency (ECHA) | |
URL | https://echa.europa.eu/substance-information/-/substanceinfo/100.124.705 | |
Description | The European Chemicals Agency (ECHA) is an agency of the European Union which is the driving force among regulatory authorities in implementing the EU's groundbreaking chemicals legislation for the benefit of human health and the environment as well as for innovation and competitiveness. | |
Explanation | Use of the information, documents and data from the ECHA website is subject to the terms and conditions of this Legal Notice, and subject to other binding limitations provided for under applicable law, the information, documents and data made available on the ECHA website may be reproduced, distributed and/or used, totally or in part, for non-commercial purposes provided that ECHA is acknowledged as the source: "Source: European Chemicals Agency, http://echa.europa.eu/". Such acknowledgement must be included in each copy of the material. ECHA permits and encourages organisations and individuals to create links to the ECHA website under the following cumulative conditions: Links can only be made to webpages that provide a link to the Legal Notice page. | |
Retrosynthesis Analysis
AI-Powered Synthesis Planning: Our tool employs the Template_relevance Pistachio, Template_relevance Bkms_metabolic, Template_relevance Pistachio_ringbreaker, Template_relevance Reaxys, Template_relevance Reaxys_biocatalysis model, leveraging a vast database of chemical reactions to predict feasible synthetic routes.
One-Step Synthesis Focus: Specifically designed for one-step synthesis, it provides concise and direct routes for your target compounds, streamlining the synthesis process.
Accurate Predictions: Utilizing the extensive PISTACHIO, BKMS_METABOLIC, PISTACHIO_RINGBREAKER, REAXYS, REAXYS_BIOCATALYSIS database, our tool offers high-accuracy predictions, reflecting the latest in chemical research and data.
Strategy Settings
Precursor scoring | Relevance Heuristic |
---|---|
Min. plausibility | 0.01 |
Model | Template_relevance |
Template Set | Pistachio/Bkms_metabolic/Pistachio_ringbreaker/Reaxys/Reaxys_biocatalysis |
Top-N result to add to graph | 6 |
Feasible Synthetic Routes
Citations
Disclaimer and Information on In-Vitro Research Products
Please be aware that all articles and product information presented on BenchChem are intended solely for informational purposes. The products available for purchase on BenchChem are specifically designed for in-vitro studies, which are conducted outside of living organisms. In-vitro studies, derived from the Latin term "in glass," involve experiments performed in controlled laboratory settings using cells or tissues. It is important to note that these products are not categorized as medicines or drugs, and they have not received approval from the FDA for the prevention, treatment, or cure of any medical condition, ailment, or disease. We must emphasize that any form of bodily introduction of these products into humans or animals is strictly prohibited by law. It is essential to adhere to these guidelines to ensure compliance with legal and ethical standards in research and experimentation.