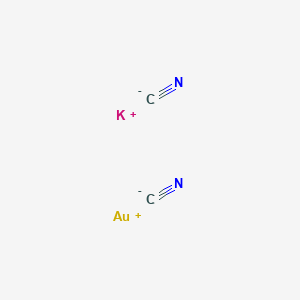
Potassium dicyanoaurate
- Click on QUICK INQUIRY to receive a quote from our team of experts.
- With the quality product at a COMPETITIVE price, you can focus more on your research.
Overview
Description
Potassium dicyanoaurate is a chemical compound with the molecular formula K[Au(CN)2]. It appears as a yellow crystalline powder that is soluble in water and has a metallic taste. This compound is widely used in various fields, including medical, environmental, and industrial research.
Preparation Methods
Synthetic Routes and Reaction Conditions: Potassium dicyanoaurate can be synthesized through the reaction of gold with potassium cyanide in the presence of oxygen. The reaction typically occurs under controlled conditions to ensure the formation of the desired product:
4Au+8KCN+O2+2H2O→4K[Au(CN)2]+4KOH
Industrial Production Methods: In industrial settings, the production of this compound involves similar synthetic routes but on a larger scale. The process is optimized for efficiency and yield, often involving continuous flow reactors and stringent quality control measures to ensure the purity of the final product.
Types of Reactions:
Oxidation: this compound can undergo oxidation reactions, where it is converted to higher oxidation states.
Reduction: It can also be reduced to elemental gold under specific conditions.
Substitution: The compound can participate in substitution reactions where the cyanide ligands are replaced by other ligands.
Common Reagents and Conditions:
Oxidation: Common oxidizing agents include hydrogen peroxide and nitric acid.
Reduction: Reducing agents such as sodium borohydride or hydrazine are often used.
Substitution: Ligands like thiourea or phosphines can be used in substitution reactions.
Major Products Formed:
Oxidation: Higher oxidation state gold complexes.
Reduction: Elemental gold.
Substitution: Gold complexes with different ligands.
Scientific Research Applications
Catalysis
Role in Catalytic Reactions:
Potassium dicyanoaurate serves as an effective catalyst in several organic reactions. Its unique properties facilitate faster and more selective chemical processes, particularly beneficial in pharmaceutical manufacturing. For instance, it has been utilized in the synthesis of complex organic molecules through various catalytic pathways.
Case Study:
A study demonstrated the use of this compound as a catalyst for the oxidation of alcohols to aldehydes and ketones. The reaction exhibited high selectivity and efficiency, showcasing the compound's potential in organic synthesis applications.
Materials Science
Gold Nanoparticle Synthesis:
One of the most significant applications of this compound is in the synthesis of gold nanoparticles. These nanoparticles are crucial for various applications in drug delivery, imaging, and diagnostics within biomedical research.
Electrochemical Applications:
The compound is also employed in electrochemical studies, enhancing the performance of sensors and batteries. Its ability to form stable gold nanoparticles contributes to improved electrochemical activity and efficiency .
Application Area | Description |
---|---|
Gold Nanoparticle Synthesis | Used as a precursor for producing gold nanoparticles for drug delivery and imaging. |
Electrochemistry | Enhances performance in sensor development and battery efficiency. |
Photovoltaic Cells | Contributes to the fabrication of thin-film solar cells for improved energy conversion. |
Biochemistry
Biochemical Assays:
this compound is utilized in biochemical assays due to its interaction with biomolecules. It can influence enzyme activity and protein interactions, making it valuable for studying biochemical pathways.
Case Study:
Research has shown that this compound can be used in assays to detect gold concentrations in biological samples, aiding in understanding gold's effects on human health .
Environmental Research
Environmental Monitoring:
The compound is employed in studies related to environmental monitoring and remediation. Its ability to bind with heavy metals allows it to be used in processes aimed at removing toxic substances from contaminated environments.
Case Study:
A study highlighted the effectiveness of this compound in binding with lead ions from wastewater, demonstrating its potential for environmental cleanup applications .
Analytical Chemistry
Detection Techniques:
In analytical chemistry, this compound is used for detecting and quantifying gold in various samples. This application is essential for quality control in industries that utilize gold or require monitoring of environmental contamination.
Case Study:
Analytical methods employing this compound have been developed to measure trace amounts of gold in environmental samples, providing critical data for pollution assessment .
Mechanism of Action
The mechanism of action of Potassium dicyanoaurate involves its interaction with molecular targets and pathways. In catalysis, the compound facilitates the activation of substrates and the formation of reactive intermediates. In biological systems, it can interact with proteins and enzymes, influencing their activity and function. The cyanide ligands play a crucial role in stabilizing the gold center and modulating its reactivity.
Comparison with Similar Compounds
Potassium dicyanoaurate can be compared with other gold cyanide complexes, such as:
Aurate(1-), tetracyano-, potassium (K[Au(CN)4]): This compound has four cyanide ligands and exhibits different reactivity and applications.
Aurate(1-), bis(cyano-c)-, sodium (Na[Au(CN)2]): Similar to the potassium salt but with sodium as the counterion, affecting its solubility and reactivity.
Uniqueness: this compound is unique due to its specific combination of gold and cyanide ligands, which confer distinct chemical and physical properties. Its solubility in water and stability make it particularly useful in various research and industrial applications.
Biological Activity
Potassium dicyanoaurate (KAu(CN)₂) is a gold(I) complex that has garnered interest in various fields, particularly in medicinal chemistry and toxicology. This article explores its biological activities, including its potential therapeutic effects and toxicological implications, supported by case studies and research findings.
This compound is a coordination compound composed of potassium ions and dicyanoaurate anions. Its unique structure allows it to interact with biological systems in diverse ways, making it a subject of interest for researchers investigating metal complexes in medicine.
Anticancer Activity
Recent studies have highlighted the anticancer properties of gold complexes, including this compound. Research indicates that gold(I) complexes can induce apoptosis in cancer cells through various mechanisms:
- Mechanism of Action : Gold complexes can interfere with cellular redox states, leading to the generation of reactive oxygen species (ROS). This oxidative stress can trigger intrinsic apoptotic pathways in cancer cells .
- Targeting Actin Dynamics : Some studies have shown that gold complexes can inhibit actin polymerization, which is crucial for cancer cell motility and proliferation. For instance, N-Heterocyclic Carbene (NHC) gold complexes demonstrated significant inhibition of actin polymerization comparable to established inhibitors like cytochalasin B .
Case Studies
-
Cholestatic Hepatitis from Gold Potassium Cyanide Ingestion :
A notable case involved a 27-year-old male who ingested this compound in a suicide attempt. He exhibited symptoms such as vomiting and hepatic dysfunction, leading to a diagnosis of acute gold toxicity. Pathological examination revealed centrilobular cholestasis and eosinophilic degeneration in the liver . This case underscores the potential hepatotoxic effects of this compound. -
Contact Allergy :
In a comparative study on contact allergies to gold salts, only one patient showed an allergic reaction to this compound among 2853 tested individuals. This suggests that while some individuals may develop sensitivities to gold compounds, this compound is less frequently implicated compared to other gold salts like sodium thiosulfate .
Toxicological Implications
The toxicological profile of this compound is significant due to its potential for causing adverse effects:
- Hepatotoxicity : The aforementioned case study reveals the risk of liver damage associated with high doses of this compound. Monitoring blood levels of gold after exposure can help assess the extent of toxicity .
- Allergic Reactions : Although rare, allergic reactions to this compound highlight the need for caution in individuals with known sensitivities to gold compounds .
Research Findings
Research has demonstrated various biological activities associated with this compound:
Properties
CAS No. |
13967-50-5 |
---|---|
Molecular Formula |
C2AuKN2 |
Molecular Weight |
288.10 g/mol |
IUPAC Name |
potassium;gold(1+);dicyanide |
InChI |
InChI=1S/2CN.Au.K/c2*1-2;;/q2*-1;2*+1 |
InChI Key |
XTFKWYDMKGAZKK-UHFFFAOYSA-N |
SMILES |
[C-]#N.[C-]#N.[K+].[Au+] |
Canonical SMILES |
[C-]#N.[C-]#N.[K+].[Au+] |
Related CAS |
14950-87-9 (Parent) |
Origin of Product |
United States |
Retrosynthesis Analysis
AI-Powered Synthesis Planning: Our tool employs the Template_relevance Pistachio, Template_relevance Bkms_metabolic, Template_relevance Pistachio_ringbreaker, Template_relevance Reaxys, Template_relevance Reaxys_biocatalysis model, leveraging a vast database of chemical reactions to predict feasible synthetic routes.
One-Step Synthesis Focus: Specifically designed for one-step synthesis, it provides concise and direct routes for your target compounds, streamlining the synthesis process.
Accurate Predictions: Utilizing the extensive PISTACHIO, BKMS_METABOLIC, PISTACHIO_RINGBREAKER, REAXYS, REAXYS_BIOCATALYSIS database, our tool offers high-accuracy predictions, reflecting the latest in chemical research and data.
Strategy Settings
Precursor scoring | Relevance Heuristic |
---|---|
Min. plausibility | 0.01 |
Model | Template_relevance |
Template Set | Pistachio/Bkms_metabolic/Pistachio_ringbreaker/Reaxys/Reaxys_biocatalysis |
Top-N result to add to graph | 6 |
Feasible Synthetic Routes
Disclaimer and Information on In-Vitro Research Products
Please be aware that all articles and product information presented on BenchChem are intended solely for informational purposes. The products available for purchase on BenchChem are specifically designed for in-vitro studies, which are conducted outside of living organisms. In-vitro studies, derived from the Latin term "in glass," involve experiments performed in controlled laboratory settings using cells or tissues. It is important to note that these products are not categorized as medicines or drugs, and they have not received approval from the FDA for the prevention, treatment, or cure of any medical condition, ailment, or disease. We must emphasize that any form of bodily introduction of these products into humans or animals is strictly prohibited by law. It is essential to adhere to these guidelines to ensure compliance with legal and ethical standards in research and experimentation.