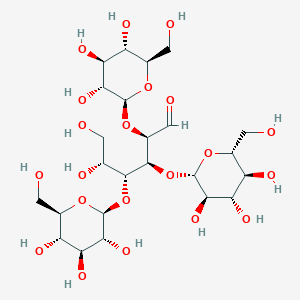
Cellopentaose
- Click on QUICK INQUIRY to receive a quote from our team of experts.
- With the quality product at a COMPETITIVE price, you can focus more on your research.
Overview
Description
Cellopentaose: is an oligosaccharide composed of five glucose units linked by β-1,4-glycosidic bonds. It is a type of cellooligosaccharide, which are derived from the partial hydrolysis of cellulose. This compound is significant in various biochemical and industrial applications due to its structural properties and its role as a substrate for enzymatic reactions.
Scientific Research Applications
Chemistry: Cellopentaose is used as a substrate in enzymatic studies to understand the mechanisms of cellulase enzymes. It serves as a model compound for studying the hydrolysis of cellulose and the action of various glycosidases.
Biology: In biological research, this compound is used to study the metabolism of oligosaccharides in microorganisms and plants. It is also employed in the development of prebiotics, which promote the growth of beneficial gut bacteria.
Medicine: this compound has potential applications in drug delivery systems due to its biocompatibility and ability to form complexes with various drugs. It is also explored for its role in promoting gut health as a prebiotic.
Industry: In the industrial sector, this compound is used in the production of biofuels and biodegradable materials. It is also utilized in the food industry as a non-digestible bulking agent and in the textile industry for processing cotton fibers .
Mechanism of Action
Target of Action
Cellopentaose, a penta oligosaccharide of cellulose , primarily targets enzymes known as endoglucanases and cellobiohydrolases . These enzymes, particularly those belonging to the glycoside hydrolase family 6 (GH6) and glycoside hydrolase family 9 (GH9), play a crucial role in the degradation of cellulose . They cleave the β-1,4-glycosidic linkages in cellulose, thereby breaking down the cellulose chains into cellooligosaccharides .
Mode of Action
This compound interacts with its target enzymes in a specific manner. For instance, GH6 cellobiohydrolase from Paenibacillus curdlanolyticus B-6 exhibits high substrate specificity towards this compound . This enzyme acts as an exo-type cellobiohydrolase, hydrolyzing cellobiose and cellooligosaccharides of higher degree of polymerization by cleaving the β-1,4 glycosidic linkages . The enzyme prefers to hydrolyze this compound, suggesting that this compound is the minimal length substrate for this enzyme .
Biochemical Pathways
The action of this compound on its target enzymes affects the biochemical pathways involved in cellulose degradation. In anaerobic cellulolytic microorganisms, the action of several cellulases releases cellobiose and longer cellodextrins, which are imported and further degraded in the cytosol to fuel the cells . The degradation of this compound by the target enzymes contributes to this process, facilitating the breakdown of cellulose and the production of energy.
Pharmacokinetics
For example, NcCel45A, an endoglucanase from Neurospora crassa OR74A, displays excellent stability at elevated temperatures up to 70 °C and pHs ranging from 5 to 9 . This suggests that the enzymes interacting with this compound may have robust pharmacokinetic properties that could impact the bioavailability of this compound.
Result of Action
The action of this compound results in the hydrolysis of cellulose, leading to the production of shorter cellooligosaccharides and cellobiose . This process contributes to the degradation of cellulose, facilitating the release of glucose units that can be used for energy production .
Action Environment
The action of this compound and its target enzymes can be influenced by various environmental factors. For instance, the activity of NcCel45A, an enzyme that interacts with this compound, is most active at pH 6.0 and 60 °C . Moreover, this enzyme is uniquely resistant to a wide range of organic solvents , suggesting that the action of this compound may be stable across a variety of environmental conditions.
Biochemical Analysis
Biochemical Properties
Cellopentaose is involved in several biochemical reactions, primarily as a substrate for enzymes such as β-glucosidases and cellulases. These enzymes catalyze the hydrolysis of this compound into glucose units. This compound interacts with cellulases, which break down the β-1,4-glycosidic bonds, and β-glucosidases, which further hydrolyze the resulting cellobiose into glucose . These interactions are crucial for the efficient degradation of cellulose in various organisms.
Cellular Effects
This compound influences various cellular processes, including cell signaling pathways, gene expression, and cellular metabolism. In microbial cells, this compound serves as a carbon source, promoting growth and energy production. It can induce the expression of genes encoding cellulolytic enzymes, enhancing the organism’s ability to degrade cellulose . Additionally, this compound affects cellular metabolism by providing glucose, which enters glycolysis and the citric acid cycle, generating ATP and other metabolic intermediates.
Molecular Mechanism
At the molecular level, this compound exerts its effects through binding interactions with cellulases and β-glucosidases. These enzymes recognize and bind to this compound, catalyzing its hydrolysis into glucose units. The binding of this compound to these enzymes involves specific amino acid residues in the active site, facilitating the cleavage of β-1,4-glycosidic bonds . This process is essential for the conversion of cellulose into fermentable sugars.
Temporal Effects in Laboratory Settings
In laboratory settings, the effects of this compound can vary over time. This compound is relatively stable under standard storage conditions, maintaining its biochemical activity for extended periods. Its degradation can occur in the presence of specific enzymes or under extreme conditions. Long-term studies have shown that this compound can sustain microbial growth and enzyme production over several days, indicating its stability and prolonged efficacy in biochemical assays .
Dosage Effects in Animal Models
The effects of this compound in animal models depend on the dosage administered. At low doses, this compound can enhance gut microbiota by serving as a prebiotic, promoting the growth of beneficial bacteria. At high doses, it may cause gastrointestinal discomfort or other adverse effects. Studies have shown that moderate doses of this compound can improve metabolic health by increasing the production of short-chain fatty acids and enhancing nutrient absorption .
Metabolic Pathways
This compound is involved in metabolic pathways related to carbohydrate metabolism. It is hydrolyzed by cellulases and β-glucosidases into glucose, which enters glycolysis and the citric acid cycle. These pathways generate ATP, NADH, and other metabolic intermediates essential for cellular functions. This compound also interacts with enzymes such as cellobiose phosphorylase, which catalyzes the phosphorolysis of cellobiose into glucose-1-phosphate and glucose .
Transport and Distribution
Within cells, this compound is transported and distributed through specific transporters and binding proteins. These transporters facilitate the uptake of this compound into the cytoplasm, where it undergoes enzymatic hydrolysis. The distribution of this compound within tissues depends on its interaction with various binding proteins, which can affect its localization and accumulation .
Preparation Methods
Synthetic Routes and Reaction Conditions: Cellopentaose can be synthesized through the controlled hydrolysis of cellulose using specific enzymes such as cellulases. The process involves breaking down cellulose into smaller oligosaccharides, including this compound. Enzymatic hydrolysis is typically carried out under mild conditions, with optimal pH and temperature settings tailored to the specific enzyme used.
Industrial Production Methods: Industrial production of this compound often involves the use of cellulase enzymes derived from microorganisms such as fungi and bacteria. The process includes the fermentation of lignocellulosic biomass, followed by enzymatic hydrolysis. The resulting mixture of cellooligosaccharides is then separated and purified using techniques such as chromatography, with cellulose affinity/partition chromatography being one of the most economical methods .
Chemical Reactions Analysis
Types of Reactions: Cellopentaose undergoes various chemical reactions, including:
Hydrolysis: Enzymatic hydrolysis by cellulases breaks down this compound into smaller glucose units.
Oxidation: this compound can be oxidized to produce gluconic acid derivatives.
Substitution: Chemical modifications can be made by substituting hydroxyl groups with other functional groups.
Common Reagents and Conditions:
Enzymatic Hydrolysis: Utilizes cellulase enzymes under optimal pH and temperature conditions.
Oxidation: Typically involves oxidizing agents such as hydrogen peroxide or periodate under controlled conditions.
Major Products Formed:
Hydrolysis: Produces glucose and smaller cellooligosaccharides.
Oxidation: Results in the formation of gluconic acid derivatives.
Comparison with Similar Compounds
Cellotriose: Composed of three glucose units linked by β-1,4-glycosidic bonds.
Cellotetraose: Composed of four glucose units linked by β-1,4-glycosidic bonds.
Maltopentaose: Composed of five glucose units linked by α-1,4-glycosidic bonds.
Uniqueness: Cellopentaose is unique due to its specific β-1,4-glycosidic linkages, which distinguish it from other oligosaccharides like maltopentaose that have α-1,4-glycosidic bonds. This structural difference influences its interaction with enzymes and its role in various biochemical processes. Additionally, this compound’s ability to act as a substrate for cellulase enzymes makes it particularly valuable in studies related to cellulose degradation and biofuel production .
Properties
{ "Design of the Synthesis Pathway": "The synthesis pathway for Cellopentaose involves the enzymatic hydrolysis of cellulose using cellulases.", "Starting Materials": [ "Cellulose", "Cellulases" ], "Reaction": [ "Cellulose is first pretreated to remove impurities and increase accessibility of the cellulose fibers.", "Cellulases are then added to the pretreated cellulose to break down the cellulose into smaller sugar units.", "The resulting mixture is then purified and the Cellopentaose is isolated using chromatography techniques." ] } | |
CAS No. |
2240-27-9 |
Molecular Formula |
C30H52O26 |
Molecular Weight |
828.7 g/mol |
IUPAC Name |
(2R,3R,4R,5R)-4-[(2S,3R,4R,5S,6R)-5-[(2S,3R,4R,5S,6R)-5-[(2S,3R,4R,5S,6R)-3,4-dihydroxy-6-(hydroxymethyl)-5-[(2S,3R,4S,5S,6R)-3,4,5-trihydroxy-6-(hydroxymethyl)oxan-2-yl]oxyoxan-2-yl]oxy-3,4-dihydroxy-6-(hydroxymethyl)oxan-2-yl]oxy-3,4-dihydroxy-6-(hydroxymethyl)oxan-2-yl]oxy-2,3,5,6-tetrahydroxyhexanal |
InChI |
InChI=1S/C30H52O26/c31-1-7(37)13(39)23(8(38)2-32)53-28-20(46)16(42)25(10(4-34)50-28)55-30-22(48)18(44)26(12(6-36)52-30)56-29-21(47)17(43)24(11(5-35)51-29)54-27-19(45)15(41)14(40)9(3-33)49-27/h1,7-30,32-48H,2-6H2/t7-,8+,9+,10+,11+,12+,13+,14+,15-,16+,17+,18+,19+,20+,21+,22+,23+,24+,25+,26+,27-,28-,29-,30-/m0/s1 |
InChI Key |
FJCUPROCOFFUSR-YIQJLYQHSA-N |
Isomeric SMILES |
C([C@@H]1[C@H]([C@@H]([C@H]([C@@H](O1)O[C@@H]2[C@H](O[C@H]([C@@H]([C@H]2O)O)O[C@@H]3[C@H](O[C@H]([C@@H]([C@H]3O)O)O[C@@H]4[C@H](O[C@H]([C@@H]([C@H]4O)O)O[C@H]([C@@H](CO)O)[C@@H]([C@H](C=O)O)O)CO)CO)CO)O)O)O)O |
SMILES |
C(C1C(C(C(C(O1)OC(C=O)C(C(C(CO)O)OC2C(C(C(C(O2)CO)O)O)O)OC3C(C(C(C(O3)CO)O)O)O)O)O)O)O |
Canonical SMILES |
C(C1C(C(C(C(O1)OC2C(OC(C(C2O)O)OC3C(OC(C(C3O)O)OC4C(OC(C(C4O)O)OC(C(CO)O)C(C(C=O)O)O)CO)CO)CO)O)O)O)O |
Synonyms |
O-β-D-Glucopyranosyl-(1-4)-O-β-D-glucopyranosyl-(1-4)-O-β-D-glucopyranosyl-(1-4)-O-β-D-glucopyranosyl-(1-4)-D-glucose; (Glc1-b-4)4-D-Glc; |
Origin of Product |
United States |
Q1: How does cellopentaose interact with carbohydrate-binding domains (CBDs)?
A1: this compound binds to CBDs, particularly those from cellulase enzymes, through a combination of hydrogen bonding and van der Waals interactions. The binding occurs along a cleft on the surface of the CBD, which typically accommodates around five glucopyranosyl units. [, , , ] This interaction plays a crucial role in the ability of cellulases to bind to and degrade cellulose.
Q2: Does this compound binding affect the stability of CBDs?
A2: Yes, binding of this compound to CBDs, such as CBDN1 from Cellulomonas fimi, enhances their thermal stability. [] This increased stability is attributed to the ligand binding shifting the unfolding equilibrium towards the native state of the protein.
Q3: What is the significance of this compound being a hydrolysis product of larger cellulose molecules?
A3: this compound acts as an intermediate product during the enzymatic breakdown of cellulose. [, , , , , ] Understanding its production and further hydrolysis by enzymes like β-glucosidases is crucial for optimizing cellulose degradation for biofuel production.
Q4: What is the molecular formula and weight of this compound?
A4: The molecular formula of this compound is C30H52O26. Its molecular weight is 828.72 g/mol.
Q5: What spectroscopic techniques are used to characterize this compound?
A5: NMR spectroscopy, including 1H-1H COSY and 13C-1H HSQC, is commonly employed to assign the structure and study the configuration of this compound in solution. X-ray photoelectron spectroscopy (XPS) can provide information about the chemical environment of carbon and oxygen atoms within the this compound molecule. []
Q6: How does this compound behave in aqueous solutions?
A6: this compound exhibits varying configurations of α- and β-anomers at the reducing end (C1) in aqueous solutions. As the degree of polymerization increases in cellooligosaccharides, the β-form becomes more prevalent. []
Q7: How do enzymes like β-glucosidases act on this compound?
A7: β-Glucosidases, such as those from Trichoderma reesei and Candida peltata, hydrolyze this compound by cleaving the β-1,4-glycosidic bonds. This process typically yields glucose and smaller cellooligosaccharides, ultimately contributing to the complete breakdown of cellulose. [, , ]
Q8: Can this compound be used as a substrate for enzymatic synthesis?
A8: Yes, this compound can serve as a substrate for certain glycosyltransferases, like xylosyltransferases from Arabidopsis thaliana. [] These enzymes can add xylosyl residues to this compound, leading to the formation of more complex carbohydrates like xyloglucan.
Q9: How is computational chemistry used to study this compound and related enzymes?
A9: Molecular docking simulations are used to predict the binding modes and interactions of this compound with enzymes like β-glucosidase B from Paenibacillus polymyxa. [] These insights help in understanding the substrate specificity and catalytic mechanism of these enzymes.
Q10: How does the chain length of cellooligosaccharides affect their binding to CBDs?
A10: Studies have shown that binding affinity to CBDs generally increases with the degree of polymerization of cellooligosaccharides. [] For example, cellohexaose exhibits a higher binding affinity compared to this compound. This suggests that a longer carbohydrate chain allows for more extensive interactions with the binding cleft of the CBD.
Q11: What is the effect of modifying specific amino acid residues in enzymes that interact with this compound?
A11: Mutating specific amino acid residues within the active site of enzymes, such as those involved in substrate binding or catalysis, can significantly impact their activity towards this compound. [] For instance, mutations in the substrate binding cleft of a rice β-glucosidase glycosynthase affected its ability to synthesize long p-nitrophenyl (pNP)-cellooligosaccharides.
Q12: How is this compound typically separated and purified from complex mixtures?
A12: Several techniques are employed for this compound purification, including:
- Size-exclusion chromatography: Bio-Gel P-2 columns are commonly used to separate this compound based on its molecular size. []
- Polyacrylamide gel chromatography: This method allows for the preparative separation of high-purity this compound. []
- Preparative silica column chromatography: This method provides efficient separation of this compound from other cellooligosaccharides. []
Q13: What methods are used to detect and quantify this compound?
A13: Common detection and quantification methods include:
- High-performance liquid chromatography (HPLC): HPLC is widely used to analyze the products of this compound hydrolysis by enzymes. [, ]
- Fluorophore-assisted carbohydrate electrophoresis (FACE): FACE offers a sensitive method for the qualitative analysis of this compound. []
- Electrospray ionization time-of-flight mass spectrometry (ESI-TOF-MS): This technique allows for the accurate determination of the molecular weight of this compound. []
Disclaimer and Information on In-Vitro Research Products
Please be aware that all articles and product information presented on BenchChem are intended solely for informational purposes. The products available for purchase on BenchChem are specifically designed for in-vitro studies, which are conducted outside of living organisms. In-vitro studies, derived from the Latin term "in glass," involve experiments performed in controlled laboratory settings using cells or tissues. It is important to note that these products are not categorized as medicines or drugs, and they have not received approval from the FDA for the prevention, treatment, or cure of any medical condition, ailment, or disease. We must emphasize that any form of bodily introduction of these products into humans or animals is strictly prohibited by law. It is essential to adhere to these guidelines to ensure compliance with legal and ethical standards in research and experimentation.