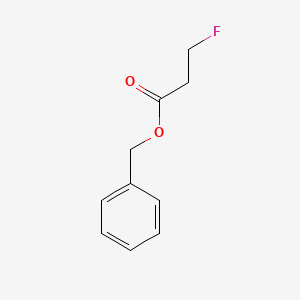
Benzyl 3-fluoropropanoate
Beschreibung
Benzyl 3-fluoropropanoate is an ester derived from benzyl alcohol and 3-fluoropropanoic acid. Its structure features a fluorine atom at the β-position (third carbon) of the propanoate chain.
Eigenschaften
IUPAC Name |
benzyl 3-fluoropropanoate | |
---|---|---|
Source | PubChem | |
URL | https://pubchem.ncbi.nlm.nih.gov | |
Description | Data deposited in or computed by PubChem | |
InChI |
InChI=1S/C10H11FO2/c11-7-6-10(12)13-8-9-4-2-1-3-5-9/h1-5H,6-8H2 | |
Source | PubChem | |
URL | https://pubchem.ncbi.nlm.nih.gov | |
Description | Data deposited in or computed by PubChem | |
InChI Key |
WSBVWPKJUJCDIR-UHFFFAOYSA-N | |
Source | PubChem | |
URL | https://pubchem.ncbi.nlm.nih.gov | |
Description | Data deposited in or computed by PubChem | |
Canonical SMILES |
C1=CC=C(C=C1)COC(=O)CCF | |
Source | PubChem | |
URL | https://pubchem.ncbi.nlm.nih.gov | |
Description | Data deposited in or computed by PubChem | |
Molecular Formula |
C10H11FO2 | |
Source | PubChem | |
URL | https://pubchem.ncbi.nlm.nih.gov | |
Description | Data deposited in or computed by PubChem | |
Molecular Weight |
182.19 g/mol | |
Source | PubChem | |
URL | https://pubchem.ncbi.nlm.nih.gov | |
Description | Data deposited in or computed by PubChem | |
Vorbereitungsmethoden
Direct Fluorination of Propanoate Esters
Electrophilic Fluorination Using TFEDMA
The stereoselective fluorination of lactic acid derivatives with N,N-diethylaminosulfur trifluoride (TFEDMA) represents a scalable one-step route. Adapted from EP2089351B1, this method substitutes methyl lactate with benzyl lactate to yield benzyl 3-fluoropropanoate. The reaction proceeds at 0–30°C in dichloromethane or 1,2-dichloroethane over 8–20 hours, achieving 70–85% yields and 96–97% enantiomeric excess (ee). The absence of solvent further simplifies purification, though prolonged reaction times may lead to racemization.
Reaction Scheme:
2\text{Cl}2, \, 25^\circ\text{C}} \text{this compound} + \text{byproducts}
Halex Fluorination with Alkali Metal Fluorides
Nucleophilic fluorination using KF or CsF in polar aprotic solvents (e.g., DMF, DMSO) is a cost-effective alternative. For example, benzyl 3-bromopropanoate reacts with anhydrous KF at 80°C for 12 hours to yield the fluorinated product. However, competing elimination reactions reduce yields to 50–60%, necessitating chromatographic purification.
Esterification of 3-Fluoropropanoic Acid
Acid-Catalyzed Fischer Esterification
Condensing 3-fluoropropanoic acid with benzyl alcohol under acidic conditions (HSO, TsOH) achieves 80–90% conversion. Azeotropic removal of water using toluene or cyclohexane shifts equilibrium toward product formation. This method is preferred for its simplicity but requires careful control of stoichiometry to minimize diastereomer formation.
Optimized Conditions:
Acylation via 3-Fluoropropanoyl Chloride
3-Fluoropropanoyl chloride, synthesized from oxalyl chloride and 3-fluoropropanoic acid, reacts with benzyl alcohol in dichloromethane at 0°C. Triethylamine neutralizes HCl, driving the reaction to completion within 2 hours. This method achieves 95% purity but requires stringent anhydrous conditions.
Process Optimization and Scalability
Design of Experiments (DoE) in Flow Chemistry
A face-centered central composite design (CCF) optimizes continuous-flow synthesis by varying residence time (0.5–3.5 min), temperature (30–70°C), and reagent equivalents. For this compound, DoE identified 2.5 min, 50°C, and 6 equivalents of fluorinating agent as optimal, improving yield from 65% to 82%.
Table 1: DoE Factors and Responses
Factor | Range | Optimal Value |
---|---|---|
Residence time (min) | 0.5–3.5 | 2.5 |
Temperature (°C) | 30–70 | 50 |
Fluorinating agent | 2–10 equivalents | 6 |
Yield | 65–82% | 82% |
Solvent and Catalytic System Screening
High-throughput screening of Brønsted acids (e.g., HSO, TsOH) and solvents (MeOH, CHCl) revealed sulfuric acid in methanol as superior for one-pot esterification-deprotection sequences. Methanol’s dual role as solvent and weak base minimized side reactions, enhancing yield to 86%.
Analyse Chemischer Reaktionen
Types of Reactions
Benzyl 3-fluoropropanoate undergoes various chemical reactions, including:
Hydrolysis: The ester group can be hydrolyzed to yield 3-fluoropropanoic acid and benzyl alcohol.
Reduction: The ester can be reduced to the corresponding alcohol using reducing agents like lithium aluminum hydride.
Substitution: The fluorine atom can be substituted with other nucleophiles under appropriate conditions.
Common Reagents and Conditions
Hydrolysis: Acidic or basic conditions with water.
Reduction: Lithium aluminum hydride in anhydrous ether.
Substitution: Nucleophiles such as amines or thiols in the presence of a base.
Major Products Formed
Hydrolysis: 3-fluoropropanoic acid and benzyl alcohol.
Reduction: 3-fluoropropanol.
Substitution: Various substituted derivatives depending on the nucleophile used.
Wissenschaftliche Forschungsanwendungen
Benzyl 3-fluoropropanoate has several applications in scientific research:
Medicinal Chemistry: It is used in the design and synthesis of potential drug candidates due to the unique properties imparted by the fluorine atom, such as increased lipophilicity and metabolic stability.
Organic Synthesis: It serves as a building block for the synthesis of more complex molecules in organic chemistry.
Material Science: The compound is explored for its potential use in the development of new materials with specific properties.
Wirkmechanismus
The mechanism of action of benzyl 3-fluoropropanoate in biological systems involves its interaction with specific molecular targets. The fluorine atom can influence the compound’s binding affinity and selectivity towards enzymes or receptors. The ester group can undergo hydrolysis to release the active 3-fluoropropanoic acid, which can then interact with its molecular targets .
Vergleich Mit ähnlichen Verbindungen
Structural and Functional Group Comparisons
Key analogs include:
Reactivity and Stability
- Hydrolysis Sensitivity : Aliphatic fluorine (as in the target compound) may accelerate ester hydrolysis compared to chlorine due to stronger electron-withdrawing effects, increasing carbonyl electrophilicity .
- Steric Effects : Trifluoromethyl groups () and bulky substituents (e.g., benzotriazolyl in ) hinder nucleophilic attack, unlike the smaller fluorine in the target compound.
Physical Properties
- Solubility: Fluorinated esters generally exhibit lower water solubility than non-halogenated analogs. However, aliphatic fluorine (target) may slightly improve solubility compared to chlorine () due to reduced hydrophobicity.
Table 1: Comparative Analysis of Key Esters
Property | This compound | Phenyl 3-Chloropropanoate | Methyl 3-Fluorobenzoate |
---|---|---|---|
Molecular Weight (g/mol) | 182.19 | 184.62 | 154.14 |
Halogen Type | F | Cl | F |
Substituent Position | Aliphatic chain | Aliphatic chain | Aromatic ring |
Predicted Hydrolysis Rate | High | Moderate | Low (stabilized by ring) |
Key Applications | Synthetic intermediate | Laboratory reagent | Organic synthesis |
Biologische Aktivität
Chemical Structure and Properties
This compound is an ester derived from 3-fluoropropanoic acid and benzyl alcohol. Its structure can be represented as follows:
The introduction of fluorine into the molecule often alters its chemical and biological properties, enhancing its potential as a bioactive compound.
Enzyme Inhibition
Fluorinated compounds are known to act as inhibitors for several enzymes. In particular, the presence of fluorine can enhance the binding affinity of inhibitors to target enzymes. Studies have shown that β-halo amino acids, which include fluorinated variants, can effectively inhibit alanine racemase (Alr), an essential enzyme in bacterial cell wall synthesis. While direct studies on this compound's role as an enzyme inhibitor are lacking, its structural characteristics imply a similar potential.
Study on Fluorinated Esters
A study focused on the biological activity of various fluorinated esters reported that certain derivatives exhibited notable insecticidal properties. Benzyl esters were part of this study, indicating that modifications to the benzyl group could influence activity against pests. Although this compound was not specifically mentioned, the findings suggest it could possess similar bioactivity.
Synthesis and Evaluation
In a synthetic study, researchers evaluated the effectiveness of this compound in various reactions, highlighting its utility in creating more complex fluorinated compounds. The synthesis involved using specific conditions that favored regioselectivity during reactions with [^18F]-fluoride. This indicates its relevance in radiochemistry and potential applications in medical imaging.
Data Tables
The following table summarizes key findings related to this compound and related compounds:
Q & A
Basic Research Questions
Q. What are the recommended methods for synthesizing Benzyl 3-fluoropropanoate, and how can reaction efficiency be optimized?
- This compound can be synthesized via esterification of 3-fluoropropanoic acid with benzyl alcohol under acid catalysis. To optimize efficiency, consider using coupling agents like HBTU or PYBOP (mentioned in ) to activate the carboxylic acid. Catalytic oxidation methods (e.g., using Fe(NO₃)₃, as in ) may also be adapted for intermediate steps. Monitor reaction progress via TLC or NMR to ensure completion.
Q. What analytical techniques are critical for characterizing this compound purity and structure?
- Use ¹H/¹³C NMR to confirm the ester linkage and fluorine substitution. GC-MS or HPLC (with UV detection) is recommended for purity assessment. For fluorinated analogs, ¹⁹F NMR provides specificity in identifying fluorine environments . Cross-reference spectral data with PubChem entries (e.g., ) for validation.
Q. How should this compound be stored to maintain stability during experiments?
Advanced Research Questions
Q. What mechanistic insights explain the reactivity of the fluorine substituent in this compound under nucleophilic conditions?
- The electron-withdrawing fluorine atom increases electrophilicity at the carbonyl carbon, enhancing susceptibility to nucleophilic attack. Computational studies (e.g., DFT calculations) can model transition states, while kinetic isotope effects (KIEs) or Hammett plots may quantify substituent effects. Compare reactivity with non-fluorinated analogs to isolate fluorine-specific contributions .
Q. How do solvent polarity and temperature influence the hydrolysis kinetics of this compound?
- Hydrolysis rates are accelerated in polar protic solvents (e.g., water/ethanol mixtures) due to stabilization of the tetrahedral intermediate. Arrhenius plots derived from variable-temperature experiments (e.g., 25–60°C) can determine activation energies. Use pH-stat titrations or in situ IR spectroscopy to track ester cleavage .
Q. What strategies mitigate side reactions during Suzuki-Miyaura cross-coupling involving fluorinated benzyl esters?
- Substitute boronic acids with sterically hindered variants (e.g., 2-benzyloxy-4-fluorophenylboronic acid; ) to reduce protodeboronation. Optimize catalyst systems (e.g., Pd(OAc)₂ with SPhos ligand) and degas solvents to prevent oxidative byproducts. Monitor boron retention via ICP-MS .
Q. How can computational modeling predict the metabolic pathways of this compound in biological systems?
Q. Data Contradiction and Validation
Q. How should researchers address discrepancies in reported melting points or spectral data for fluorinated esters?
- Cross-validate data across multiple sources (e.g., PubChem, Kanto Reagents catalogs) and replicate measurements using calibrated instruments. For example, discrepancies in boronic acid melting points ( vs. 3) may arise from polymorphic forms or impurities. Publish raw data (e.g., DSC thermograms) to enhance reproducibility .
Q. What protocols ensure reproducibility in fluorinated compound synthesis across different laboratories?
Q. Safety and Compliance
Q. What first-aid measures are critical for accidental exposure to this compound?
Q. How should waste containing fluorinated esters be disposed of to comply with environmental regulations?
Featured Recommendations
Most viewed | ||
---|---|---|
Most popular with customers |
Haftungsausschluss und Informationen zu In-Vitro-Forschungsprodukten
Bitte beachten Sie, dass alle Artikel und Produktinformationen, die auf BenchChem präsentiert werden, ausschließlich zu Informationszwecken bestimmt sind. Die auf BenchChem zum Kauf angebotenen Produkte sind speziell für In-vitro-Studien konzipiert, die außerhalb lebender Organismen durchgeführt werden. In-vitro-Studien, abgeleitet von dem lateinischen Begriff "in Glas", beinhalten Experimente, die in kontrollierten Laborumgebungen unter Verwendung von Zellen oder Geweben durchgeführt werden. Es ist wichtig zu beachten, dass diese Produkte nicht als Arzneimittel oder Medikamente eingestuft sind und keine Zulassung der FDA für die Vorbeugung, Behandlung oder Heilung von medizinischen Zuständen, Beschwerden oder Krankheiten erhalten haben. Wir müssen betonen, dass jede Form der körperlichen Einführung dieser Produkte in Menschen oder Tiere gesetzlich strikt untersagt ist. Es ist unerlässlich, sich an diese Richtlinien zu halten, um die Einhaltung rechtlicher und ethischer Standards in Forschung und Experiment zu gewährleisten.