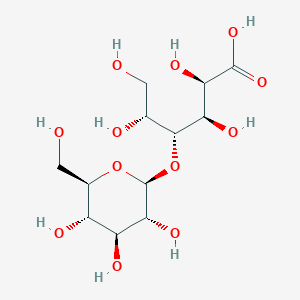
Cellobionic acid
Übersicht
Beschreibung
Cellobionic acid (CBA) is an aldobionic acid consisting of a β-1,4-linked disaccharide (glucose and gluconic acid) with a carboxyl group at the anomeric position of the non-reducing end . It is a key product of oxidative cellulose degradation by lytic polysaccharide monooxygenases (LPMOs) and cellobiose dehydrogenases (CDHs) in fungal and bacterial systems . Unlike conventional hydrolytic cellulases, LPMOs generate oxidized sugars like CBA, which enhance biomass saccharification by creating new cleavage sites in crystalline cellulose . CBA has gained attention as a sustainable substrate for biofuel production due to its compatibility with microbial fermentation pathways .
Vorbereitungsmethoden
Synthetic Routes and Reaction Conditions: Cellobionic acid can be synthesized through the oxidation of cellobiose. One common method involves the use of whole-cell biocatalysts, such as genetically modified Pseudomonas taetrolens or Gluconobacter oxydans. These microorganisms possess membrane-bound dehydrogenases that facilitate the oxidation process .
For example, Pseudomonas taetrolens expressing quinoprotein glucose dehydrogenase can convert cellobiose to this compound with high efficiency. The reaction conditions typically include a reaction temperature of 35°C, a cell density of 10 at OD 600nm, and a cellobiose concentration of 200 g/L. Under these conditions, the conversion can be completed in approximately 11 hours .
Industrial Production Methods: Industrial production of this compound can be achieved using whole-cell biocatalysis in stirred tank bioreactors. The engineered strains of Gluconobacter oxydans or Pseudomonas taetrolens are cultivated, and the cells are harvested and resuspended in a reaction buffer containing cellobiose. The biotransformation is carried out under aerobic conditions, and the product is subsequently purified .
Analyse Chemischer Reaktionen
Production of Cellobionic Acid via Bio-oxidation
Gluconobacter oxydans can efficiently bio-oxidize cellobiose to produce this compound. Studies have shown that engineered G. oxydans strains can yield high concentrations of CBA in aerobic batch processes .
-
The oxidation of cellobiose into CBA begins immediately at the start of biotransformation, with maximum oxygen uptake rate (OUR) .
-
The conversion rate decreases rapidly within 15 hours. Complete conversion can be achieved in around 21 hours, with a product yield exceeding 99% (mol/mol) .
-
To oxidize 98 g/L of cellobiose to this compound, 143 mM of is required .
Impact of Cellobiose Concentration on CBA Production
Initial Cellobiose Concentration (g/L) | Product Formation Rate (g/g/h) |
---|---|
25 | 2.1 |
50 | 4.9 |
98 | 8.3 |
190 | 13.9 |
290 | Not Applicable |
Hydrolytic Oxidation of Cellobiose Using Catalysts
Heterogeneous catalysts containing noble metals like platinum (Pt), gold (Au), ruthenium (Ru), and palladium (Pd) on a hypercrosslinked polystyrene (HPS) matrix can be used for hydrolytic oxidation of cellobiose . Platinum-containing catalysts have demonstrated high activity, achieving 100% cellobiose conversion .
Enzymatic Production of this compound from Cellulose
Neurospora crassa can produce cellobionate directly from cellulose using the enzymes cellulase and cellobiose dehydrogenase (CDH). Cellulases hydrolyze cellulose to cellobiose, while CDH oxidizes cellobiose to cellobionate . The oxidation of CDH is usually slow when molecular oxygen is the electron acceptor. The addition of laccase and a redox mediator improves the re-oxidation of CDH .
-
At pH 6, cellobiose conversion completes within 24 hours, with an average of 27.9 mM cellobiose converted to 28.6 mM CBA, showing an approximate 1:1 molar conversion .
-
Acidic conditions are required for efficient cellobiose to CBA conversion using the CDH-ABTS-laccase system .
This compound Phosphorylase Reaction
This compound phosphorylase (CBAP) catalyzes the reversible phosphorolysis of this compound into glucose 1-phosphate and gluconic acid .
-
The reaction is reversible, and the synthetic reaction using G1P and GlcA as the donor and acceptor substrates produces CBA and inorganic phosphate .
-
Deletion of the NcCBAP gene (ndvB) in N. crassa results in CbA accumulation during cultivation on cellulose .
Parameter | Value |
---|---|
kcat | 92 ± 2 s-1 |
KmA | 0.12 ± 0.03 mm |
KiA | 0.44 ± 0.2 mm |
KmB | 0.061 ± 0.009 mm |
Wissenschaftliche Forschungsanwendungen
Production Methods
The production of cellobionic acid can be achieved through various biotechnological approaches:
- Whole-Cell Biocatalysis : Recent studies have demonstrated the use of genetically modified strains of Pseudomonas taetrolens to convert cellobiose into CBA efficiently. This method reported a productivity of 18.2 g/L/h under optimized conditions, achieving complete conversion in 11 hours with sustained catalytic activity over multiple cycles .
- In Vitro Synthetic Enzymatic Systems : An innovative approach involves using an artificial in vitro synthetic enzymatic biosystem (ivSEBS) that utilizes starch as a substrate. This method has shown promising results, yielding 21.2 g/L CBA from maltodextrin in a scalable process .
- Bio-Oxidation Techniques : Engineered strains of Gluconobacter oxydans have also been employed to produce high concentrations of CBA through bio-oxidation processes, achieving yields greater than 99% .
Biochemical Properties
This compound is characterized by its unique chemical structure and properties that facilitate its applications:
- Chelation Properties : CBA acts as an efficient chelator for manganese ions (Mn(III)), which is beneficial in various catalytic processes and environmental remediation efforts .
- Metabolic Pathways : The compound plays a role in microbial cellulose degradation and can be phosphorylated to produce glucose-1-phosphate and gluconic acid through the action of this compound phosphorylase (CBAP) . Understanding these metabolic pathways is crucial for optimizing production methods and enhancing yield.
Pharmaceutical Industry
CBA has potential applications in drug formulation due to its biocompatibility and ability to enhance the solubility of poorly soluble drugs. Its role as a precursor in synthesizing bioactive compounds makes it valuable in pharmaceutical research.
Cosmetic Industry
In cosmetics, this compound is recognized for its moisturizing properties and ability to improve skin texture. Its antioxidant capabilities contribute to skin health, making it an appealing ingredient in skincare products.
Food Industry
CBA can serve as a food additive due to its natural origin and safety profile. Its sweetness and functional properties may enhance food products while providing nutritional benefits.
Environmental Applications
The chelation properties of CBA make it useful for bioremediation processes, particularly in removing heavy metals from contaminated environments. Its role in microbial metabolism also supports carbon cycling and sustainable practices in waste management.
Case Studies
Wirkmechanismus
The mechanism of action of cellobionic acid involves its interaction with specific enzymes and molecular targets:
Enzymatic Degradation: this compound is hydrolyzed by β-glucosidase to produce glucose and gluconic acid.
Oxidative Pathways: this compound is produced through the action of cellobiose dehydrogenase, which oxidizes cellobiose to cellobionolactone.
This compound Phosphorylase: This enzyme catalyzes the degradation of this compound, linking oxidative cellulose degradation to bioethanol fermentation pathways in microorganisms.
Vergleich Mit ähnlichen Verbindungen
Comparison with Similar Compounds
Structural and Functional Comparison
CBA belongs to the aldobionic acid family, which includes lactobionic acid (LBA) and maltobionic acid (MBA) . Key differences are:
- Key Insight : The β-1,4 linkage in CBA makes it resistant to hydrolysis by standard cellulases but degradable by specialized β-glucosidases (e.g., NCU08755 in Neurospora crassa) . In contrast, MBA’s α-1,4 linkage allows faster enzymatic processing in food industries .
Enzymatic Hydrolysis Efficiency
CBA hydrolysis is slower than cellobiose due to steric hindrance from the carboxyl group. For example:
- Cellic CTCec2 (industrial enzyme cocktail) hydrolyzes CBA at ~20% the rate of cellobiose .
- Novozym 188 (β-glucosidase) shows negligible activity on CBA, highlighting the need for specialized enzymes like CAP (this compound phosphorylase) .
Table 1: Hydrolysis Rates of this compound vs. Cellobiose
Enzyme Source | Substrate | Hydrolysis Rate (μmol/min/mg) | Reference |
---|---|---|---|
Cellic CTCec2 | Cellobiose | 12.5 ± 1.2 | |
Cellic CTCec2 | This compound | 2.5 ± 0.3 | |
N. crassa CAP | This compound | 8.7 ± 0.9 |
Metabolic Pathways in Microorganisms
- Neurospora crassa : Utilizes CBA via two pathways:
- Saccharomyces cerevisiae: Requires genetic engineering to express N. crassa CAP and gluconokinase (GnK) for CBA utilization. Co-feeding with xylose improves anaerobic CBA consumption by 5.5-fold .
- Escherichia coli: Naturally metabolizes CBA via the cryptic gene ascB, producing isobutanol at yields comparable to glucose (0.31 g/g) .
Table 2: CBA Utilization in Engineered Microbes
Organism | Pathway Components | Product Yield | Reference |
---|---|---|---|
E. coli (wild-type) | AscB (6-phospho-β-glucosidase) | Isobutanol: 0.31 g/g | |
S. cerevisiae | CBT-1 (transporter), CAP, GnK | Ethanol: 0.22 g/g |
Biologische Aktivität
Cellobionic acid (CBA) is a disaccharide formed from the coupling of β-D-glucosyl and D-gluconic acid residues through a (1→4) linkage. It has garnered attention due to its potential applications in various industries, including pharmaceuticals, cosmetics, and food. This article explores the biological activity of this compound, focusing on its enzymatic production, metabolic pathways, and implications for biotechnological applications.
Enzymatic Production and Metabolism
This compound Phosphorylase (CBAP)
One of the key enzymes involved in the metabolism of this compound is this compound phosphorylase (CBAP). This enzyme catalyzes the reversible phosphorolysis of CBA into glucose 1-phosphate and gluconic acid. Research has shown that CBAP exhibits a unique substrate recognition site that is crucial for its activity against aldonic acids, indicating its specialized role in carbohydrate metabolism .
Table 1: Kinetic Parameters of CBAP
Parameter | Value |
---|---|
92 ± 2 s | |
(A) | 0.12 ± 0.03 mM |
(A) | 0.44 ± 0.2 mM |
(B) | 0.061 ± 0.009 mM |
The kinetic parameters indicate that CBAP has a high catalytic efficiency, making it a significant player in the degradation of cellulose-derived products .
Microbial Production
this compound can also be produced by various microorganisms. For instance, Fibrobacter succinogenes, an anaerobic bacterium, was found to produce high levels of CBA when grown on cellulose or cellobiose substrates. This suggests that CBA plays a role in microbial cellulose degradation processes .
Applications of this compound
Biotechnological Applications
this compound has potential applications in several fields:
- Pharmaceuticals : Due to its biocompatibility and metabolic properties.
- Food Industry : As a natural sweetener or preservative.
- Cosmetics : In formulations for skin care due to its moisturizing properties.
Recent studies have demonstrated innovative methods for synthesizing CBA from inexpensive substrates like starch using engineered enzymatic systems, achieving significant yields . This approach highlights the economic viability of producing CBA on an industrial scale.
Case Studies
Case Study 1: Engineered Production Using Neurospora crassa
A study demonstrated that an engineered strain of Neurospora crassa could produce this compound directly from cellulose without the need for exogenous cellulases. By optimizing fermentation conditions and adding laccase as a redox mediator, researchers achieved substantial yields of CBA from cellulose substrates .
Case Study 2: Sustainable Synthesis from Starch
Another innovative approach involved developing an artificial in vitro synthetic enzymatic biosystem (ivSEBS) that enabled sustainable production of CBA from starch. Under optimized conditions, this system achieved a concentration of 6.2 g/L CBA from maltodextrin with a conversion rate of 60%, showcasing the potential for large-scale production .
Q & A
Basic Research Questions
Q. What are the established protocols for synthesizing and characterizing cellobionic acid in laboratory settings?
- Methodological Answer : this compound synthesis typically involves enzymatic or chemical oxidation of cellobiose. For enzymatic routes, glucose-methanol-choline (GMC) oxidoreductases or laccase-mediator systems are used, with reaction conditions optimized for pH (4.5–6.0) and temperature (25–40°C) . Characterization requires HPLC for purity analysis, NMR (e.g., for carboxyl group identification), and mass spectrometry for molecular weight confirmation. Ensure detailed documentation of solvent systems, catalyst concentrations, and purification steps to enable reproducibility .
Q. How can researchers design experiments to assess this compound’s role in lignocellulose degradation?
- Methodological Answer : Use a comparative approach with controlled variables:
- Substrate : Pure cellulose vs. lignocellulosic biomass.
- Enzymes : Include cellobiose dehydrogenases (CDHs) or lytic polysaccharide monooxygenases (LPMOs) from the CAZy database (e.g., Family AA9) .
- Controls : Omit enzymes or add inhibitors (e.g., EDTA for metalloenzymes).
Quantify this compound via colorimetric assays (e.g., DNS method) and correlate with reducing sugar yields. Replicate experiments ≥3 times to account for biomass heterogeneity .
Advanced Research Questions
Q. How should researchers resolve contradictions in reported enzymatic efficiency of this compound production across studies?
- Methodological Answer : Contradictions often arise from:
- Enzyme source : Recombinant vs. wild-type enzymes (e.g., Phanerochaete chrysosporium CDH vs. Neurospora crassa LPMO) may differ in redox cooperativity .
- Reaction milieu : Oxygen availability and electron donors (e.g., ascorbic acid) impact oxidation rates.
Address discrepancies by standardizing assay conditions (e.g., O saturation monitoring) and validating via orthogonal methods (e.g., HPLC vs. enzymatic assays) .
Q. What strategies are recommended for elucidating this compound’s metabolic pathways in microbial systems?
- Methodological Answer :
- Isotopic labeling : Use -cellobiose to trace carbon flux via GC-MS.
- Gene knockout : Target putative transporters (e.g., cbtA in E. coli) or phosphorylases to identify uptake/assimilation pathways.
- Multi-omics : Pair transcriptomics (RNA-seq) with metabolomics (LC-MS) to map regulatory networks under varying substrate conditions. Cross-reference data with KEGG/UniPathway annotations .
Q. How can computational modeling enhance understanding of this compound’s interactions with carbohydrate-active enzymes?
- Methodological Answer :
- Docking simulations : Use tools like AutoDock Vina to predict binding affinities with CAZy Family 1 glycoside hydrolases.
- MD simulations : Analyze solvation effects and hydrogen-bond stability in aqueous vs. non-polar environments (GROMACS/NAMD).
Validate models with mutagenesis (e.g., active-site residues D221/E222 in TrCel7A) and kinetic assays (, ) .
Q. Data Analysis & Reproducibility
Q. What statistical frameworks are suitable for analyzing this compound’s inhibitory effects on cellulases?
- Methodological Answer :
- Dose-response curves : Fit data to a sigmoidal model (e.g., Hill equation) to calculate IC.
- ANOVA : Compare inhibition across enzyme classes (e.g., endoglucanases vs. exoglucanases) with post-hoc Tukey tests.
Report effect sizes (Cohen’s d) and confidence intervals (95% CI) to quantify uncertainty .
Q. How can researchers ensure reproducibility when publishing this compound-related findings?
- Methodological Answer :
- Supplemental data : Include raw chromatograms, NMR spectra, and enzyme lot numbers.
- Code sharing : Upload scripts for data analysis (e.g., Python/R) to repositories like Zenodo.
- Replication guides : Detail biomass pretreatment protocols (e.g., particle size, moisture content) to mitigate batch variability .
Q. Literature & Resource Evaluation
Q. How to critically assess conflicting hypotheses about this compound’s role in fungal signaling?
- Methodological Answer :
- Primary vs. secondary sources : Prioritize studies with direct experimental evidence (e.g., qPCR for gene expression) over review articles.
- Bias evaluation : Check for conflicts of interest (e.g., industry-funded studies on biofuel applications).
Use tools like CONSORT for experimental rigor assessment .
Q. Ethical & Practical Considerations
Q. What FINER criteria apply when formulating this compound research questions?
- Methodological Answer :
Eigenschaften
IUPAC Name |
(2R,3R,4R,5R)-2,3,5,6-tetrahydroxy-4-[(2S,3R,4S,5S,6R)-3,4,5-trihydroxy-6-(hydroxymethyl)oxan-2-yl]oxyhexanoic acid | |
---|---|---|
Source | PubChem | |
URL | https://pubchem.ncbi.nlm.nih.gov | |
Description | Data deposited in or computed by PubChem | |
InChI |
InChI=1S/C12H22O12/c13-1-3(15)10(7(18)8(19)11(21)22)24-12-9(20)6(17)5(16)4(2-14)23-12/h3-10,12-20H,1-2H2,(H,21,22)/t3-,4-,5-,6+,7-,8-,9-,10-,12+/m1/s1 | |
Source | PubChem | |
URL | https://pubchem.ncbi.nlm.nih.gov | |
Description | Data deposited in or computed by PubChem | |
InChI Key |
JYTUSYBCFIZPBE-ZNLUKOTNSA-N | |
Source | PubChem | |
URL | https://pubchem.ncbi.nlm.nih.gov | |
Description | Data deposited in or computed by PubChem | |
Canonical SMILES |
C(C1C(C(C(C(O1)OC(C(CO)O)C(C(C(=O)O)O)O)O)O)O)O | |
Source | PubChem | |
URL | https://pubchem.ncbi.nlm.nih.gov | |
Description | Data deposited in or computed by PubChem | |
Isomeric SMILES |
C([C@@H]1[C@H]([C@@H]([C@H]([C@@H](O1)O[C@H]([C@@H](CO)O)[C@@H]([C@H](C(=O)O)O)O)O)O)O)O | |
Source | PubChem | |
URL | https://pubchem.ncbi.nlm.nih.gov | |
Description | Data deposited in or computed by PubChem | |
Molecular Formula |
C12H22O12 | |
Source | PubChem | |
URL | https://pubchem.ncbi.nlm.nih.gov | |
Description | Data deposited in or computed by PubChem | |
DSSTOX Substance ID |
DTXSID70201562 | |
Record name | Cellobionic acid | |
Source | EPA DSSTox | |
URL | https://comptox.epa.gov/dashboard/DTXSID70201562 | |
Description | DSSTox provides a high quality public chemistry resource for supporting improved predictive toxicology. | |
Molecular Weight |
358.30 g/mol | |
Source | PubChem | |
URL | https://pubchem.ncbi.nlm.nih.gov | |
Description | Data deposited in or computed by PubChem | |
CAS No. |
534-41-8 | |
Record name | Cellobionic acid | |
Source | CAS Common Chemistry | |
URL | https://commonchemistry.cas.org/detail?cas_rn=534-41-8 | |
Description | CAS Common Chemistry is an open community resource for accessing chemical information. Nearly 500,000 chemical substances from CAS REGISTRY cover areas of community interest, including common and frequently regulated chemicals, and those relevant to high school and undergraduate chemistry classes. This chemical information, curated by our expert scientists, is provided in alignment with our mission as a division of the American Chemical Society. | |
Explanation | The data from CAS Common Chemistry is provided under a CC-BY-NC 4.0 license, unless otherwise stated. | |
Record name | Cellobionic acid | |
Source | ChemIDplus | |
URL | https://pubchem.ncbi.nlm.nih.gov/substance/?source=chemidplus&sourceid=0000534418 | |
Description | ChemIDplus is a free, web search system that provides access to the structure and nomenclature authority files used for the identification of chemical substances cited in National Library of Medicine (NLM) databases, including the TOXNET system. | |
Record name | Cellobionic acid | |
Source | EPA DSSTox | |
URL | https://comptox.epa.gov/dashboard/DTXSID70201562 | |
Description | DSSTox provides a high quality public chemistry resource for supporting improved predictive toxicology. | |
Haftungsausschluss und Informationen zu In-Vitro-Forschungsprodukten
Bitte beachten Sie, dass alle Artikel und Produktinformationen, die auf BenchChem präsentiert werden, ausschließlich zu Informationszwecken bestimmt sind. Die auf BenchChem zum Kauf angebotenen Produkte sind speziell für In-vitro-Studien konzipiert, die außerhalb lebender Organismen durchgeführt werden. In-vitro-Studien, abgeleitet von dem lateinischen Begriff "in Glas", beinhalten Experimente, die in kontrollierten Laborumgebungen unter Verwendung von Zellen oder Geweben durchgeführt werden. Es ist wichtig zu beachten, dass diese Produkte nicht als Arzneimittel oder Medikamente eingestuft sind und keine Zulassung der FDA für die Vorbeugung, Behandlung oder Heilung von medizinischen Zuständen, Beschwerden oder Krankheiten erhalten haben. Wir müssen betonen, dass jede Form der körperlichen Einführung dieser Produkte in Menschen oder Tiere gesetzlich strikt untersagt ist. Es ist unerlässlich, sich an diese Richtlinien zu halten, um die Einhaltung rechtlicher und ethischer Standards in Forschung und Experiment zu gewährleisten.