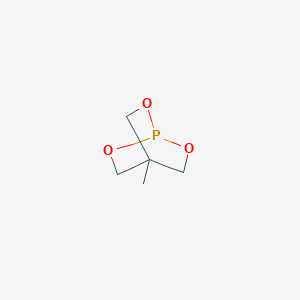
Trimethylolethane cyclic phosphite
- Klicken Sie auf QUICK INQUIRY, um ein Angebot von unserem Expertenteam zu erhalten.
- Mit qualitativ hochwertigen Produkten zu einem WETTBEWERBSFÄHIGEN Preis können Sie sich mehr auf Ihre Forschung konzentrieren.
Übersicht
Beschreibung
Trimethylolethane cyclic phosphite is a six-membered cyclic organophosphorus compound derived from the esterification of trimethylolethane (2-(hydroxymethyl)-2-methyl-1,3-propanediol) with phosphorous acid. Its structure features a central phosphorus atom bonded to three oxygen atoms, forming a cyclic triester with a rigid six-membered ring. This compound is notable for its role in coordination chemistry, particularly as a ligand in transition metal complexes, such as rhodium catalysts . The constrained geometry of the cyclic phosphite enhances stability and influences its electronic properties, making it valuable in catalytic applications.
Vorbereitungsmethoden
. Die Reaktion umfasst typischerweise die folgenden Schritte:
Reaktion von Phenylbernsteinsäure mit Methylamin: Diese Reaktion wird in Gegenwart eines Katalysators wie Palladiumdiacetat und 4,4'-Bipyridin in Tetrahydrofuran und Wasser bei 80 °C für 24 Stunden durchgeführt.
Analyse Chemischer Reaktionen
Phensuximid unterliegt verschiedenen Arten von chemischen Reaktionen, darunter:
Oxidation: Phensuximid kann oxidiert werden, um verschiedene Oxidationsprodukte zu bilden.
Reduktion: Es kann unter bestimmten Bedingungen reduziert werden, um reduzierte Formen der Verbindung zu erhalten.
Substitution: Phensuximid kann Substitutionsreaktionen eingehen, insbesondere am Stickstoffatom.
Häufige Reagenzien und Bedingungen: Typische Reagenzien sind Oxidationsmittel wie Kaliumpermanganat und Reduktionsmittel wie Lithiumaluminiumhydrid.
Hauptprodukte: Die Hauptprodukte, die aus diesen Reaktionen gebildet werden, hängen von den verwendeten spezifischen Reagenzien und Bedingungen ab.
Wissenschaftliche Forschungsanwendungen
Phensuximid hat mehrere Anwendungen in der wissenschaftlichen Forschung:
Chemie: Es wird als Modellverbindung in Studien zu Succinimidderivaten und deren Reaktivität verwendet.
Biologie: Phensuximid wird auf seine Auswirkungen auf die neuronale Aktivität und seine potenziellen neuroprotektiven Eigenschaften untersucht.
Medizin: Neben seiner Verwendung bei der Behandlung von Epilepsie wird Phensuximid auf seine potenzielle Verwendung bei der Behandlung anderer neurologischer Erkrankungen untersucht.
Industrie: Die Verbindung wird bei der Synthese anderer Pharmazeutika und als Referenzstandard in der analytischen Chemie verwendet
5. Wirkmechanismus
Der genaue Wirkmechanismus von Phensuximid ist nicht vollständig geklärt. Es wird angenommen, dass es auf inhibitorischen neuronalen Systemen wirkt, die wichtig für die Generierung des Drei-pro-Sekunde-Rhythmus sind, der mit Petit-Mal-Anfällen verbunden ist. Seine Wirkungen können mit seiner Fähigkeit zusammenhängen, die durch Depolarisation induzierte Akkumulation von cyclischem AMP und cyclischem GMP im Gehirngewebe zu hemmen .
Wirkmechanismus
The exact mechanism of action of phensuximide is not fully understood. it is believed to act on inhibitory neuronal systems that are important in generating the three-per-second rhythm associated with petit mal seizures. Its effects may be related to its ability to inhibit depolarization-induced accumulation of cyclic AMP and cyclic GMP in brain tissue .
Vergleich Mit ähnlichen Verbindungen
Comparative Structural Analysis with Analogous Compounds
Trimethylolethane cyclic phosphite is distinguished from other phosphorus compounds by its cyclic structure and substituent arrangement. Key comparisons include:
Non-Cyclic Phosphites
- Trimethyl Phosphite (CAS 121-45-9): A non-cyclic triester with three methoxy groups bonded to phosphorus. Unlike this compound, its linear structure allows free rotation around P–O bonds, resulting in lower thermal stability and distinct reactivity in hydrolysis and oxidation reactions .
- Triethylphosphine (CAS 554-70-1): A tertiary phosphine with a pyramidal geometry. While both compounds act as ligands, Triethylphosphine’s stronger σ-donor capacity contrasts with the cyclic phosphite’s weaker π-acceptor properties .
Constrained Cyclic Phosphites
- 1-Methyl-4-phospha-3,5,8-trioxabicyclo[2.2.2]octane: A bicyclic phosphite with restricted alkoxy group rotation. This constraint leads to a higher dipole moment (1.94 D) compared to non-cyclic analogs, similar to this compound’s rigid geometry .
- Spirocyclic Phosphazenes: These nitrogen-phosphorus hybrids (e.g., tetrachloromonospirocyclotriphosphazenes) exhibit sp³ hybridization at phosphorus but differ in reactivity due to the presence of P–N bonds instead of P–O bonds .
Thermal and Chemical Stability
- This compound : The six-membered ring structure confers high thermal stability, as evidenced by its use in rhodium complexes synthesized at elevated temperatures .
- Trimethyl Phosphite : Prone to hydrolysis under acidic conditions, limiting its utility in aqueous environments. Its decomposition temperature (~150°C) is lower than cyclic analogs .
- Constrained Bicyclic Phosphites : Exhibit exceptional stability due to restricted conformational flexibility, though synthesis challenges (e.g., requiring transesterification at 120°C) limit scalability .
Data Tables Summarizing Key Comparisons
Table 1: Structural and Functional Comparison
Table 2: Stability and Reactivity
Compound | Thermal Stability | Hydrolytic Stability | Notable Reactivity |
---|---|---|---|
This compound | High | Moderate | Stable under catalytic conditions |
Trimethyl Phosphite | Moderate | Low | Rapid hydrolysis in acidic media |
Triethylphosphine | Low | High | Pyrophoric, strong σ-donor ligand |
Q & A
Basic Research Questions
Q. What synthetic methodologies are reliable for preparing trimethylolethane cyclic phosphite, and how can reaction efficiency be monitored?
- Methodological Answer: The synthesis typically involves transesterification reactions between diols and phosphite precursors. For example, substituting diols like 1,3-diphenylpropane-1,3-diol with trimethylolethane under mild conditions (room temperature in pyridine) allows quantitative conversion, as confirmed by <sup>31</sup>P NMR spectroscopy . Monitoring reaction progress via <sup>1</sup>H and <sup>31</sup>P NMR is critical to track intermediate formation and avoid side reactions. Thermal stability during synthesis should be controlled, as elevated temperatures (e.g., 120°C) may destabilize the cyclic phosphite structure .
Q. Which analytical techniques are most effective for characterizing the structural and electronic properties of this compound?
- Methodological Answer: X-ray crystallography is indispensable for resolving the three-dimensional orientation of the phosphite ligand in coordination complexes, as demonstrated in rhodium-based studies . Complementarily, infrared (IR) spectroscopy can identify P=O and P–O–C vibrational modes, while <sup>31</sup>P NMR provides insights into phosphorus coordination environments. For electronic properties, cyclic voltammetry (CV) can assess redox behavior, though this requires immobilizing the compound in an electrolyte system .
Q. How should researchers handle and store this compound to ensure stability?
- Methodological Answer: Store the compound in anhydrous conditions under inert gas (e.g., argon) to prevent hydrolysis. Thermal stability tests suggest decomposition occurs above 120°C, so refrigeration (4°C) is recommended for long-term storage . Safety data sheets (SDS) for analogous phosphites emphasize avoiding moisture and incompatible substances like strong oxidizers .
Advanced Research Questions
Q. How can structural modifications of this compound enhance its performance in asymmetric catalysis?
- Methodological Answer: Modifying the phosphite’s substituents (e.g., introducing chiral diols or fluorinated groups) can tune steric and electronic effects. For example, phosphite-pyridine ligands (e.g., L73/L74) achieve >99% enantioselectivity in Pd-catalyzed allylic substitutions by optimizing donor groups . X-ray crystallography of rhodium complexes reveals how ligand orientation affects catalytic activity, guiding rational design .
Q. What experimental approaches reconcile contradictions in cyclic phosphite synthesis yields across studies?
- Methodological Answer: Discrepancies often arise from diol geometry and reaction conditions. For instance, five-membered cyclic phosphites may fail due to ring strain, while six-membered analogs (using 1,3-diols) form readily . Systematic variation of diol chain length, solvent polarity, and catalyst loading—coupled with DFT calculations—can identify thermodynamic vs. kinetic control factors .
Q. How does this compound interact with enzymes like phosphite dehydrogenase, and how can this be quantified?
- Methodological Answer: Phosphite dehydrogenase (Pdh) variants can bind non-natural cofactors, but interactions with cyclic phosphites require enzymatic assays. Measure activity via NADH/NAD<sup>+</sup> coupling or <sup>31</sup>P NMR to track phosphite oxidation. Structural studies (e.g., X-ray crystallography) of Pdh-phosphite complexes reveal binding motifs, enabling mutagenesis to enhance specificity .
Q. What electrochemical methods are suitable for studying this compound in energy storage systems?
- Methodological Answer: Cyclic voltammetry (CV) and electrochemical impedance spectroscopy (EIS) evaluate redox stability and interfacial resistance in battery electrolytes. For example, phosphite additives in Li-ion cells reduce flammability; similar protocols can test trimethylolethane derivatives by integrating them into model electrolytes (e.g., 1 M LiPF6 in EC/DMC) and cycling against graphite anodes .
Q. How can researchers design experiments to assess the phytotoxicity or biostimulant effects of this compound?
- Methodological Answer: Adopt randomized block designs with controlled phosphite concentrations (e.g., 0–5.00 L ha<sup>-1</sup>) and measure plant growth metrics (height, biomass) and pathogen resistance. EC50 values for fungal inhibition (e.g., Phytophthora) are calculated via dose-response curves using logarithmic trendlines . Metabolomic profiling (LC-MS/MS) can identify biochemical pathways influenced by phosphite .
Eigenschaften
CAS-Nummer |
1449-91-8 |
---|---|
Molekularformel |
C5H9O3P |
Molekulargewicht |
148.1 g/mol |
IUPAC-Name |
4-methyl-2,6,7-trioxa-1-phosphabicyclo[2.2.2]octane |
InChI |
InChI=1S/C5H9O3P/c1-5-2-6-9(7-3-5)8-4-5/h2-4H2,1H3 |
InChI-Schlüssel |
OMAIORNZIIDXOB-UHFFFAOYSA-N |
SMILES |
CC12COP(OC1)OC2 |
Kanonische SMILES |
CC12COP(OC1)OC2 |
Key on ui other cas no. |
1449-91-8 |
Herkunft des Produkts |
United States |
Haftungsausschluss und Informationen zu In-Vitro-Forschungsprodukten
Bitte beachten Sie, dass alle Artikel und Produktinformationen, die auf BenchChem präsentiert werden, ausschließlich zu Informationszwecken bestimmt sind. Die auf BenchChem zum Kauf angebotenen Produkte sind speziell für In-vitro-Studien konzipiert, die außerhalb lebender Organismen durchgeführt werden. In-vitro-Studien, abgeleitet von dem lateinischen Begriff "in Glas", beinhalten Experimente, die in kontrollierten Laborumgebungen unter Verwendung von Zellen oder Geweben durchgeführt werden. Es ist wichtig zu beachten, dass diese Produkte nicht als Arzneimittel oder Medikamente eingestuft sind und keine Zulassung der FDA für die Vorbeugung, Behandlung oder Heilung von medizinischen Zuständen, Beschwerden oder Krankheiten erhalten haben. Wir müssen betonen, dass jede Form der körperlichen Einführung dieser Produkte in Menschen oder Tiere gesetzlich strikt untersagt ist. Es ist unerlässlich, sich an diese Richtlinien zu halten, um die Einhaltung rechtlicher und ethischer Standards in Forschung und Experiment zu gewährleisten.