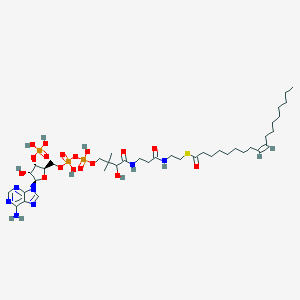
oleoyl-CoA
Descripción general
Descripción
Oleoyl-CoA (cis-Δ⁹-octadecenoyl-CoA) is a long-chain acyl-CoA thioester derived from oleic acid, a monounsaturated fatty acid (18:1n-9). It serves as a critical metabolic intermediate in lipid biosynthesis, membrane remodeling, and energy production. This compound participates in key enzymatic reactions, including triglyceride synthesis via diacylglycerol O-acyltransferase 1 (DGAT1) , β-oxidation, and desaturation pathways. Its amphipathic nature allows it to interact with membrane-bound enzymes and regulatory proteins, modulating ion channel activity (e.g., KATP channels) and enzyme kinetics .
Métodos De Preparación
Enzymatic Synthesis of Oleoyl-CoA
Substrate Activation and Transfer Mechanisms
The enzymatic synthesis of this compound involves two sequential steps: activation of oleic acid to oleoyl-adenylate and transfer of the oleoyl group to coenzyme A (CoA). This process is catalyzed by acyl-CoA synthetases (ACS), which require ATP and magnesium ions as cofactors . The reaction proceeds as follows:
Key parameters influencing yield include:
Enzyme Sources and Specificity
-
Mammalian systems : Liver ACS isoforms (ACSL1, ACSL3) achieve 80–90% conversion efficiency .
-
Microbial systems : Saccharomyces cerevisiae acyl-CoA synthetase Faa1p produces this compound at 0.8 μmol/min/mg protein .
-
Plant systems : Rapeseed (Brassica napus) elongases exhibit dual specificity for this compound and ATP-dependent primer elongation .
Chemical Synthesis Approaches
Direct Esterification of Oleic Acid
Chemical methods bypass enzymatic dependency but require stringent conditions to prevent hydrolysis:
Reagents and Conditions :
Reagent | Role | Optimal Concentration |
---|---|---|
Dicyclohexylcarbodiimide (DCC) | Carboxyl activation | 1.2 equivalents |
4-Dimethylaminopyridine (DMAP) | Nucleophilic catalyst | 0.1 equivalents |
Solvent | Anhydrous tetrahydrofuran (THF) | 0.5 M substrate |
Challenges :
Industrial Biotechnological Production
Engineered Microbial Systems
Industrial-scale synthesis employs genetically modified Escherichia coli or Yarrowia lipolytica strains overexpressing:
Case Study :
A Mycobacterium smegmatis strain expressing RaaS transcriptional regulator achieved 12 g/L this compound in fed-batch fermentation . Key process parameters included:
Downstream Processing
Purification Steps :
-
Cell disruption : High-pressure homogenization at 1,500 bar
-
Chromatography :
Comparative Analysis of Methods
Parameter | Enzymatic Synthesis | Chemical Synthesis | Microbial Production |
---|---|---|---|
Yield (%) | 85–92 | 55–65 | 90–95 |
Purity (%) | 98 | 75 | 95 |
Scale-up cost | High | Moderate | Low |
Byproducts | AMP, PP | Dicyclohexylurea | Cellular debris |
Key Findings :
Aplicaciones Científicas De Investigación
Role in Mycobacterial Survival
Oleoyl-CoA plays a significant role in the survival mechanisms of mycobacteria. Research indicates that it regulates the interaction of the transcriptional regulator RaaS with DNA, which is essential for mycobacterial persistence under stress conditions. In a study, this compound was shown to bind directly to RaaS, influencing gene expression related to mycobacterial efflux pumps and metabolic adaptation during growth phases. This binding was quantified with a dissociation constant () of approximately 3.65 μM, highlighting its potency as a regulatory molecule in bacterial metabolism .
Case Study: Mycobacterial Growth Regulation
- Objective : To understand how this compound affects mycobacterial gene expression.
- Method : Electrophoretic mobility shift assays (EMSAs) were used to assess the binding of this compound to RaaS.
- Findings : this compound completely inhibited RaaS-mediated band shifts at concentrations above 15 μM, indicating its strong regulatory role .
Modulation of K ATP Channels
This compound is also recognized for its ability to modulate ATP-sensitive potassium (K ATP) channels in cardiac and pancreatic tissues. Studies have demonstrated that this compound significantly reduces the ATP sensitivity of these channels, which is critical for maintaining cellular energy homeostasis. The modulation mechanism appears similar to that of phosphatidylinositol phosphates (PIPs), suggesting a shared pathway in lipid-mediated signaling .
Data Table: Effects of this compound on K ATP Channels
Channel Type | Initial (μM) | After this compound (μM) | Fold Change |
---|---|---|---|
Cardiac | 8 ± 0.4 | 1.4 ± 0.1 | 180 ± 12 |
Pancreatic | 18 ± 3 | 1.6 ± 0.3 | 99 ± 25 |
Industrial Applications
In industrial contexts, this compound derivatives are used in the synthesis of various bio-based lubricants and additives. For instance, estolides derived from oleic acid and other hydroxy fatty acids have been synthesized using immobilized lipases, showcasing this compound's utility in producing environmentally friendly lubricants with desirable properties like low viscosity and good thermal stability .
Case Study: Estolide Synthesis
- Objective : To synthesize estolides from oleic acid.
- Method : Utilization of immobilized lipases in solvent-free conditions.
- Results : The estolides exhibited excellent low-temperature properties and viscosity indices suitable for industrial applications .
Pharmacological Implications
This compound's influence extends into pharmacology, where it has been implicated in drug formulation strategies. For example, its role as a component in Pluronic lecithin organogels has been explored for enhancing the transdermal delivery of therapeutic agents like dexamethasone and ketoprofen. The presence of this compound improved the permeability profile of these drugs compared to traditional formulations .
Data Table: Drug Delivery Efficacy
Formulation Type | Drug Loaded | Permeability Improvement Factor |
---|---|---|
Pluronic Lecithin Organogel (Control) | Dexamethasone (0.5%) | Baseline |
Pluronic Lecithin Organogel (with this compound) | Ketoprofen (10%) | 3x |
Mecanismo De Acción
El oleoil-CoA ejerce sus efectos principalmente a través de su papel como donante de grupos acilo en diversas reacciones bioquímicas. Está involucrado en la síntesis de lípidos complejos, incluidos los fosfolípidos, los triglicéridos y los ésteres de colesterol. Los objetivos moleculares del oleoil-CoA incluyen enzimas como la acil-CoA:colesterol aciltransferasa, que cataliza la esterificación del colesterol, y la lisofosfatidilcolina aciltransferasa, que incorpora grupos oleoil en los fosfolípidos .
Compuestos Similares:
Palmitoil-CoA: Un derivado saturado de acil-CoA involucrado en vías metabólicas similares al oleoil-CoA.
Estearoil-CoA: Otro acil-CoA saturado que sirve como sustrato para la desaturación para formar oleoil-CoA.
Linoleoil-CoA: Un derivado de acil-CoA insaturado involucrado en la biosíntesis de ácidos grasos poliinsaturados.
Unicidad del Oleoil-CoA: El oleoil-CoA es único debido a su papel como producto principal de la estearoil-CoA desaturasa 1, que cataliza la conversión de estearoil-CoA a oleoil-CoA. Este paso de desaturación es crucial para la biosíntesis de ácidos grasos monoinsaturados, que son componentes esenciales de las membranas celulares y las moléculas de señalización .
Comparación Con Compuestos Similares
Comparison with Similar Acyl-CoA Compounds
Oleoyl-CoA is functionally and structurally distinct from other acyl-CoA thioesters. Below is a systematic comparison based on enzymatic interactions, binding affinities, and metabolic roles:
Substrate Specificity in Enzymatic Reactions
Modulation of Ion Channels
Structural and Binding Differences
- This compound vs. Palmitoyl-CoA: this compound’s monounsaturated cis-Δ⁹ bond induces kinked geometry, enhancing membrane insertion and enzyme binding flexibility compared to saturated palmitoyl-CoA .
- This compound vs. Stearoyl-CoA: SCD1 converts stearoyl-CoA to this compound, which is essential for synthesizing Harderian gland lipids; SCD1 knockout mice lack this pathway .
Mechanistic Insights from Mutagenesis and Binding Studies
- DGAT1-Oleoyl-CoA Interaction :
- KATP Channel Regulation :
Metabolic Pathways Involving this compound
- Triglyceride Synthesis: this compound is the preferred substrate for DGAT1, with a turnover rate (kcat) of 948 nmol/min/mg .
- Very Long-Chain Fatty Acid (VLCFA) Elongation: this compound is elongated to hexacosenoyl-CoA (26:1-CoA) in ABCD1-deficient cells, highlighting its role in pathological lipid accumulation .
- Cytochrome c-Mediated Lipid Production :
- This compound reacts with glycine to form oleoylglycine, with a Km of ~30 μM and maximal activity at 100 μM substrate .
Q & A
Basic Research Questions
Q. What methodologies are recommended for extracting and quantifying oleoyl-CoA from biological samples?
- Extraction : Use the Bligh-Dyer method (chloroform:methanol:water, 2:1:0.8 v/v) to isolate total lipids efficiently . For tissues with high water content, the Folch method (chloroform:methanol, 2:1 v/v) is preferred to minimize lipid degradation .
- Quantification : Employ liquid chromatography-mass spectrometry (LC-MS) for precise identification and quantification. Tandem MS (MS/MS) fragmentation patterns (e.g., m/z 1031.98 for this compound) confirm specificity .
Q. How can enzymatic assays assess this compound’s role in lipid metabolism?
- DGAT1 Inhibition : Use competitive inhibition assays with radiolabeled this compound (e.g., ³H-oleoyl-CoA) to study acyltransferase activity. Dose-dependent inhibition curves and Akaike’s criterion help determine kinetic models .
- Acyltransferase Specificity : Test LPGAT1’s preference for this compound over other acyl donors (e.g., lauroyl-CoA) via in vitro assays measuring phosphatidylglycerol synthesis rates .
Q. What techniques validate this compound stability in experimental setups?
- Store lyophilized this compound at -20°C (stable for 3 years) and dissolved forms at -80°C (6 months). Use bovine serum albumin (BSA) to prevent nonspecific binding in electrophysiology or binding assays .
Q. How is this compound desaturase cloned and expressed in heterologous systems?
- Extract total RNA from target tissues (e.g., oil palm mesocarp) and isolate mRNA for cDNA synthesis. Use Southern/Northern blotting to confirm gene integration and expression in E. coli .
Advanced Research Questions
Q. How can structural interactions between this compound and enzymes like ACAT1 be analyzed?
- Combine site-directed mutagenesis (e.g., S456Q/V452Q mutations in ACAT1) with LC-MS to assess binding affinity changes. Structural data (e.g., cryo-EM) can identify hydrophobic tunnels critical for this compound docking .
Q. What experimental designs resolve contradictions in this compound’s activating vs. inhibitory effects?
- Systematically vary concentrations (e.g., 1–100 µM) and assay conditions. For ion channels (e.g., K2P TREK subfamily), use inside-out patch clamp electrophysiology to measure activation, while DGAT1 assays require radiolabeled substrates to quantify inhibition .
Q. How is kinetic analysis performed for this compound-dependent enzymes?
- Fit dose-response data to competitive inhibition models (e.g., Cheng-Prusoff equation for IC50). Use Akaike’s information criterion (AICc) to select optimal kinetic models for enzymes like DGAT1 .
Q. What electrophysiological approaches study this compound’s modulation of ion channels?
- Apply 10 µM this compound to intracellular membranes via inside-out patches in Xenopus oocytes. Normalize current responses at +40 mV to basal activity and use BSA washout to confirm reversibility .
Q. How do mutagenesis studies probe this compound binding sites?
- Design mutants based on structural predictions (e.g., ACAT1’s cytosolic tunnel). Validate using LC-MS to quantify this compound binding and functional assays (e.g., acyl-CoA:cholesterol acyltransferase activity) .
Q. What advanced chromatographic techniques characterize this compound in enzyme complexes?
- Use LC-MS/MS with extracted ion chromatograms (EICs) to detect this compound in purified enzymes. Compare fragmentation patterns with standards and validate using loss-of-function mutants .
Q. Methodological Considerations for Data Contradictions
- Context-Dependent Effects : this compound may activate K2P channels at 10 µM but inhibit DGAT1 at similar concentrations . Control variables include tissue type (e.g., oocyte vs. mammalian cells), assay pH, and co-factor availability.
- Validation : Cross-validate findings using orthogonal methods (e.g., LC-MS for binding, enzymatic activity assays for function) .
Propiedades
IUPAC Name |
S-[2-[3-[[4-[[[5-(6-aminopurin-9-yl)-4-hydroxy-3-phosphonooxyoxolan-2-yl]methoxy-hydroxyphosphoryl]oxy-hydroxyphosphoryl]oxy-2-hydroxy-3,3-dimethylbutanoyl]amino]propanoylamino]ethyl] octadec-9-enethioate | |
---|---|---|
Details | Computed by Lexichem TK 2.7.0 (PubChem release 2021.05.07) | |
Source | PubChem | |
URL | https://pubchem.ncbi.nlm.nih.gov | |
Description | Data deposited in or computed by PubChem | |
InChI |
InChI=1S/C39H68N7O17P3S/c1-4-5-6-7-8-9-10-11-12-13-14-15-16-17-18-19-30(48)67-23-22-41-29(47)20-21-42-37(51)34(50)39(2,3)25-60-66(57,58)63-65(55,56)59-24-28-33(62-64(52,53)54)32(49)38(61-28)46-27-45-31-35(40)43-26-44-36(31)46/h11-12,26-28,32-34,38,49-50H,4-10,13-25H2,1-3H3,(H,41,47)(H,42,51)(H,55,56)(H,57,58)(H2,40,43,44)(H2,52,53,54) | |
Details | Computed by InChI 1.0.6 (PubChem release 2021.05.07) | |
Source | PubChem | |
URL | https://pubchem.ncbi.nlm.nih.gov | |
Description | Data deposited in or computed by PubChem | |
InChI Key |
XDUHQPOXLUAVEE-UHFFFAOYSA-N | |
Details | Computed by InChI 1.0.6 (PubChem release 2021.05.07) | |
Source | PubChem | |
URL | https://pubchem.ncbi.nlm.nih.gov | |
Description | Data deposited in or computed by PubChem | |
Canonical SMILES |
CCCCCCCCC=CCCCCCCCC(=O)SCCNC(=O)CCNC(=O)C(C(C)(C)COP(=O)(O)OP(=O)(O)OCC1C(C(C(O1)N2C=NC3=C(N=CN=C32)N)O)OP(=O)(O)O)O | |
Details | Computed by OEChem 2.3.0 (PubChem release 2021.05.07) | |
Source | PubChem | |
URL | https://pubchem.ncbi.nlm.nih.gov | |
Description | Data deposited in or computed by PubChem | |
Molecular Formula |
C39H68N7O17P3S | |
Details | Computed by PubChem 2.1 (PubChem release 2021.05.07) | |
Source | PubChem | |
URL | https://pubchem.ncbi.nlm.nih.gov | |
Description | Data deposited in or computed by PubChem | |
Molecular Weight |
1032.0 g/mol | |
Details | Computed by PubChem 2.1 (PubChem release 2021.05.07) | |
Source | PubChem | |
URL | https://pubchem.ncbi.nlm.nih.gov | |
Description | Data deposited in or computed by PubChem | |
Retrosynthesis Analysis
AI-Powered Synthesis Planning: Our tool employs the Template_relevance Pistachio, Template_relevance Bkms_metabolic, Template_relevance Pistachio_ringbreaker, Template_relevance Reaxys, Template_relevance Reaxys_biocatalysis model, leveraging a vast database of chemical reactions to predict feasible synthetic routes.
One-Step Synthesis Focus: Specifically designed for one-step synthesis, it provides concise and direct routes for your target compounds, streamlining the synthesis process.
Accurate Predictions: Utilizing the extensive PISTACHIO, BKMS_METABOLIC, PISTACHIO_RINGBREAKER, REAXYS, REAXYS_BIOCATALYSIS database, our tool offers high-accuracy predictions, reflecting the latest in chemical research and data.
Strategy Settings
Precursor scoring | Relevance Heuristic |
---|---|
Min. plausibility | 0.01 |
Model | Template_relevance |
Template Set | Pistachio/Bkms_metabolic/Pistachio_ringbreaker/Reaxys/Reaxys_biocatalysis |
Top-N result to add to graph | 6 |
Feasible Synthetic Routes
Descargo de responsabilidad e información sobre productos de investigación in vitro
Tenga en cuenta que todos los artículos e información de productos presentados en BenchChem están destinados únicamente con fines informativos. Los productos disponibles para la compra en BenchChem están diseñados específicamente para estudios in vitro, que se realizan fuera de organismos vivos. Los estudios in vitro, derivados del término latino "in vidrio", involucran experimentos realizados en entornos de laboratorio controlados utilizando células o tejidos. Es importante tener en cuenta que estos productos no se clasifican como medicamentos y no han recibido la aprobación de la FDA para la prevención, tratamiento o cura de ninguna condición médica, dolencia o enfermedad. Debemos enfatizar que cualquier forma de introducción corporal de estos productos en humanos o animales está estrictamente prohibida por ley. Es esencial adherirse a estas pautas para garantizar el cumplimiento de los estándares legales y éticos en la investigación y experimentación.