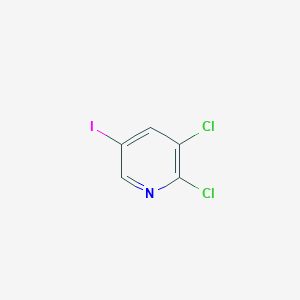
2,3-Dichloro-5-iodopyridine
Descripción
2,3-Dichloro-5-iodopyridine (C₅H₂Cl₂IN; molecular weight: 274.39 g/mol) is a halogenated pyridine derivative characterized by chlorine substituents at positions 2 and 3 and an iodine atom at position 4. It is synthesized via multi-step halogenation reactions starting from 5-chloro-6-hydroxynicotinic acid, involving diazotization with iodine (I₂) and potassium iodide (KI), followed by treatment with phosphorus pentachloride (PCl₅) . The compound’s electron-deficient aromatic ring and bulky iodine substituent make it reactive toward nucleophilic substitution and cross-coupling reactions, which are valuable in pharmaceutical and agrochemical synthesis.
Propiedades
IUPAC Name |
2,3-dichloro-5-iodopyridine | |
---|---|---|
Source | PubChem | |
URL | https://pubchem.ncbi.nlm.nih.gov | |
Description | Data deposited in or computed by PubChem | |
InChI |
InChI=1S/C5H2Cl2IN/c6-4-1-3(8)2-9-5(4)7/h1-2H | |
Source | PubChem | |
URL | https://pubchem.ncbi.nlm.nih.gov | |
Description | Data deposited in or computed by PubChem | |
InChI Key |
XSHFSZAXOOQKQS-UHFFFAOYSA-N | |
Source | PubChem | |
URL | https://pubchem.ncbi.nlm.nih.gov | |
Description | Data deposited in or computed by PubChem | |
Canonical SMILES |
C1=C(C=NC(=C1Cl)Cl)I | |
Source | PubChem | |
URL | https://pubchem.ncbi.nlm.nih.gov | |
Description | Data deposited in or computed by PubChem | |
Molecular Formula |
C5H2Cl2IN | |
Source | PubChem | |
URL | https://pubchem.ncbi.nlm.nih.gov | |
Description | Data deposited in or computed by PubChem | |
DSSTOX Substance ID |
DTXSID40619554 | |
Record name | 2,3-Dichloro-5-iodopyridine | |
Source | EPA DSSTox | |
URL | https://comptox.epa.gov/dashboard/DTXSID40619554 | |
Description | DSSTox provides a high quality public chemistry resource for supporting improved predictive toxicology. | |
Molecular Weight |
273.88 g/mol | |
Source | PubChem | |
URL | https://pubchem.ncbi.nlm.nih.gov | |
Description | Data deposited in or computed by PubChem | |
CAS No. |
97966-01-3 | |
Record name | 2,3-Dichloro-5-iodopyridine | |
Source | CAS Common Chemistry | |
URL | https://commonchemistry.cas.org/detail?cas_rn=97966-01-3 | |
Description | CAS Common Chemistry is an open community resource for accessing chemical information. Nearly 500,000 chemical substances from CAS REGISTRY cover areas of community interest, including common and frequently regulated chemicals, and those relevant to high school and undergraduate chemistry classes. This chemical information, curated by our expert scientists, is provided in alignment with our mission as a division of the American Chemical Society. | |
Explanation | The data from CAS Common Chemistry is provided under a CC-BY-NC 4.0 license, unless otherwise stated. | |
Record name | 2,3-Dichloro-5-iodopyridine | |
Source | EPA DSSTox | |
URL | https://comptox.epa.gov/dashboard/DTXSID40619554 | |
Description | DSSTox provides a high quality public chemistry resource for supporting improved predictive toxicology. | |
Métodos De Preparación
Synthetic Routes and Reaction Conditions: 2,3-Dichloro-5-iodopyridine can be synthesized through several methods. One common approach involves the chlorination of 3-chloro-5-iodo-2-pyridinol or the lithiation of 2-bromo-2,3-dichloropyridine at reduced temperatures . These methods typically require specific reaction conditions, such as the use of strong bases and controlled temperatures, to ensure the desired product is obtained with high purity and yield.
Industrial Production Methods: In industrial settings, the production of this compound often involves large-scale chlorination and iodination processes. These methods are optimized for efficiency and cost-effectiveness, utilizing advanced techniques and equipment to handle the reactive intermediates and ensure consistent product quality.
Análisis De Reacciones Químicas
Types of Reactions: 2,3-Dichloro-5-iodopyridine undergoes various chemical reactions, including:
Substitution Reactions: The compound can participate in nucleophilic substitution reactions, where the chlorine or iodine atoms are replaced by other functional groups.
Coupling Reactions: It can be used in palladium-catalyzed cross-coupling reactions, such as Suzuki-Miyaura and Stille couplings, to form carbon-carbon bonds
Common Reagents and Conditions:
Nucleophilic Substitution: Reagents like potassium fluoride (KF) in dimethylformamide (DMF) are commonly used.
Cross-Coupling Reactions: Palladium catalysts and bases like potassium carbonate (K2CO3) are typically employed.
Major Products: The major products formed from these reactions depend on the specific reagents and conditions used. For example, coupling reactions can yield various substituted pyridines, which are valuable intermediates in organic synthesis .
Aplicaciones Científicas De Investigación
2,3-Dichloro-5-iodopyridine has a wide range of applications in scientific research:
Biology: The compound is used in the development of biologically active molecules, such as enzyme inhibitors and receptor modulators.
Mecanismo De Acción
The mechanism by which 2,3-Dichloro-5-iodopyridine exerts its effects is primarily through its ability to participate in various chemical reactions. Its halogen atoms (chlorine and iodine) make it highly reactive, allowing it to form new bonds and modify existing molecular structures. This reactivity is harnessed in synthetic chemistry to create new compounds with desired properties.
Comparación Con Compuestos Similares
Comparison with Structurally Similar Compounds
2-Chloro-5-iodopyridine
- Molecular Formula : C₅H₃ClIN (239.44 g/mol)
- Substituents : Chlorine at position 2 and iodine at position 5.
- Key Differences :
- Applications : Used as a precursor in Suzuki-Miyaura couplings due to iodine’s role as a leaving group.
2,5-Dichloro-3-iodopyridine
- Molecular Formula : C₅H₂Cl₂IN (274.39 g/mol)
- Substituents : Chlorine at positions 2 and 5, iodine at position 3.
- Key Differences: Iodine at position 3 alters regioselectivity in substitution reactions compared to iodine at position 5 in this compound.
2,3-Dichloro-5-(trifluoromethyl)pyridine
- Molecular Formula : C₆H₂Cl₂F₃N (216.0 g/mol)
- Substituents : Chlorine at positions 2 and 3, trifluoromethyl (-CF₃) at position 5.
- Key Differences :
6-Chloro-5-iodo-3-nitropyridin-2-amine
- Molecular Formula : C₅H₃ClIN₃O₂ (316.46 g/mol)
- Substituents: Chlorine at position 6, iodine at position 5, nitro (-NO₂) at position 3, and amine (-NH₂) at position 2.
- Key Differences: The nitro group strongly deactivates the ring, directing electrophilic substitution to specific positions.
Structural and Reactivity Analysis
Electronic Effects
- This compound : The combined electron-withdrawing effects of two chlorine atoms and iodine create a highly electron-deficient ring, favoring nucleophilic aromatic substitution (NAS) at positions 4 and 6.
- Comparison :
- 2,3-Dichloro-5-(trifluoromethyl)pyridine : -CF₃ exerts stronger electron withdrawal than iodine, further reducing NAS reactivity but enhancing stability .
- 2-Chloro-5-iodopyridine : Reduced deactivation (only one chlorine) increases susceptibility to electrophilic substitution compared to the dichloro analog .
Steric Effects
- Iodine’s large atomic radius in this compound introduces steric hindrance at position 5, limiting access for bulky reagents.
- Comparison :
- In 2,5-Dichloro-3-iodopyridine , iodine at position 3 creates hindrance near the 2-chloro substituent, altering reaction pathways .
Actividad Biológica
Antitumor Activity
A significant area of research focuses on the antitumor potential of this compound and its derivatives. Studies have shown that compounds with similar structures can inhibit cancer cell proliferation by modulating key signaling pathways involved in tumor growth. For instance, derivatives have been evaluated for their ability to inhibit checkpoint kinase 1 (CHK1), an important regulator in the DNA damage response pathway.
Case Studies
- CHK1 Inhibition : A study investigated the development of CHK1 inhibitors derived from pyridine scaffolds, including those structurally related to this compound. These inhibitors demonstrated potent antitumor activity in vivo and were optimized for oral bioavailability, indicating their potential as therapeutic agents in cancer treatment .
- Cytotoxicity Assays : In vitro assays have been conducted to assess the cytotoxic effects of this compound on various cancer cell lines. Results indicated significant growth inhibition at micromolar concentrations, suggesting its efficacy as a lead compound for further development .
Comparative Analysis with Similar Compounds
To understand the unique properties of this compound, it is essential to compare it with structurally similar compounds:
Compound Name | Structure Description | Biological Activity |
---|---|---|
2-Chloro-3-iodopyridin-4-amine | One less chlorine atom; similar reactivity | Moderate cytotoxicity |
3-Amino-4-iodopyridine | Lacks chlorine atoms; different reactivity profile | Low cytotoxicity |
2,6-Dichloro-3-iodopyridin-4-amine | Chlorine atoms in different positions; similar uses | High anti-inflammatory activity |
2-Chloro-3,5-diiodopyridin-4-amine | Contains two iodine atoms; altered electronic properties | Potential antiviral properties |
The unique arrangement of halogen substituents on the pyridine ring contributes to the distinct reactivity and biological activity profiles among these compounds.
Synthesis and Applications
The synthesis of this compound typically involves several key steps that include halogenation of pyridine derivatives followed by purification processes such as recrystallization or chromatography. Its applications extend beyond medicinal chemistry; it also shows promise in agrochemical formulations due to its ability to act as a reactive intermediate in various chemical transformations.
Q & A
Q. What are the optimal synthetic routes for preparing 2,3-Dichloro-5-iodopyridine, and how do reaction conditions influence yield?
Basic
The synthesis typically involves sequential halogenation. A validated method (Example 3 in ) starts with 5-chloro-6-hydroxynicotinic acid, which undergoes iodination using I₂ and KI in aqueous Na₂CO₃ at 100°C under N₂. Subsequent chlorination with PCl₅ and POCl₃ yields the final product. Key factors include:
- Temperature control : Maintaining 100°C ensures efficient iodination.
- Stoichiometry : Excess PCl₅ (1.06 eq) minimizes incomplete chlorination.
- Reaction time : Extended heating (e.g., 4–6 hours) improves yield but risks decomposition.
Yields (~70–80%) depend on purity of intermediates and avoidance of moisture .
Q. What spectroscopic techniques are most effective for characterizing this compound?
Basic
Standard characterization includes:
- NMR : ¹H/¹³C NMR identifies substitution patterns (e.g., aromatic protons at δ 8.2–8.5 ppm).
- Mass spectrometry (HRMS) : Confirms molecular ion [M+H]⁺ at m/z 288.83 (C₅HCl₂IN).
- X-ray crystallography : Resolves crystal structure and halogen bonding (referenced in for similar compounds).
Cross-referencing with databases like PubChem ensures data validation .
Q. How can researchers resolve contradictions in reported reactivity of halogenated pyridines, such as competing substitution sites?
Advanced
Contradictions arise from varying electronic effects (e.g., iodine’s −I effect vs. chlorine’s +M effect). Strategies include:
- Computational modeling : Use software like MOE () to calculate Fukui indices for electrophilic/nucleophilic attack.
- Isotopic labeling : Track substitution pathways (e.g., ¹²⁷I vs. ¹²⁵I).
- Comparative studies : Test reactivity under identical conditions (e.g., solvent, catalyst) to isolate variables .
Q. What strategies mitigate competing side reactions during functionalization of this compound?
Advanced
Side reactions (e.g., dehalogenation or dimerization) are minimized by:
- Protecting groups : Temporarily block reactive sites (e.g., silylation of hydroxyl intermediates).
- Low-temperature catalysis : Pd-mediated cross-coupling at −20°C reduces iodine displacement.
- Inert atmospheres : N₂ or Ar prevents oxidation (critical in Example 2, ). Monitor progress via HPLC to detect intermediates .
Q. What are the key safety considerations when handling this compound in laboratory settings?
Basic
- PPE : Wear nitrile gloves, goggles, and a lab coat to avoid skin/eye contact (similar to 2-Chloro-5-nitropyridine in ).
- Ventilation : Use fume hoods due to potential release of HI or Cl₂ gases during decomposition.
- Spill management : Neutralize with sodium bicarbonate and adsorb using vermiculite .
Q. How does the electronic effect of iodine influence regioselectivity in nucleophilic aromatic substitution (NAS)?
Advanced
Iodine’s strong −I effect deactivates the pyridine ring, directing nucleophiles to the meta position (C-5). However, steric hindrance at C-5 (due to iodine’s size) may shift attack to less hindered sites (e.g., C-4). Experimental validation via competitive reactions (e.g., using NH₃ vs. alkoxides) and DFT calculations () clarifies dominant pathways .
Q. What common impurities arise in this compound synthesis, and how are they identified?
Basic
Common impurities include:
- Incomplete chlorination products : 3-Chloro-2-hydroxy-5-iodopyridine (detected via TLC, Rf ~0.3 in hexane:EtOAc 7:3).
- Di-iodinated byproducts : Identified by HRMS peaks at m/z 416.78.
Purification via column chromatography (silica gel, gradient elution) or recrystallization (ethanol/water) improves purity .
Q. How can computational chemistry predict reactivity and stability of this compound derivatives?
Advanced
- Molecular dynamics (MD) : Simulate solvent effects on reaction pathways (e.g., DMF vs. THF).
- Density Functional Theory (DFT) : Calculate bond dissociation energies (BDEs) to assess stability. For example, C–I BDE (~55 kcal/mol) predicts susceptibility to displacement.
- Docking studies : Predict binding affinity in medicinal chemistry applications (e.g., kinase inhibitors) .
Retrosynthesis Analysis
AI-Powered Synthesis Planning: Our tool employs the Template_relevance Pistachio, Template_relevance Bkms_metabolic, Template_relevance Pistachio_ringbreaker, Template_relevance Reaxys, Template_relevance Reaxys_biocatalysis model, leveraging a vast database of chemical reactions to predict feasible synthetic routes.
One-Step Synthesis Focus: Specifically designed for one-step synthesis, it provides concise and direct routes for your target compounds, streamlining the synthesis process.
Accurate Predictions: Utilizing the extensive PISTACHIO, BKMS_METABOLIC, PISTACHIO_RINGBREAKER, REAXYS, REAXYS_BIOCATALYSIS database, our tool offers high-accuracy predictions, reflecting the latest in chemical research and data.
Strategy Settings
Precursor scoring | Relevance Heuristic |
---|---|
Min. plausibility | 0.01 |
Model | Template_relevance |
Template Set | Pistachio/Bkms_metabolic/Pistachio_ringbreaker/Reaxys/Reaxys_biocatalysis |
Top-N result to add to graph | 6 |
Feasible Synthetic Routes
Featured Recommendations
Most viewed | ||
---|---|---|
Most popular with customers |
Descargo de responsabilidad e información sobre productos de investigación in vitro
Tenga en cuenta que todos los artículos e información de productos presentados en BenchChem están destinados únicamente con fines informativos. Los productos disponibles para la compra en BenchChem están diseñados específicamente para estudios in vitro, que se realizan fuera de organismos vivos. Los estudios in vitro, derivados del término latino "in vidrio", involucran experimentos realizados en entornos de laboratorio controlados utilizando células o tejidos. Es importante tener en cuenta que estos productos no se clasifican como medicamentos y no han recibido la aprobación de la FDA para la prevención, tratamiento o cura de ninguna condición médica, dolencia o enfermedad. Debemos enfatizar que cualquier forma de introducción corporal de estos productos en humanos o animales está estrictamente prohibida por ley. Es esencial adherirse a estas pautas para garantizar el cumplimiento de los estándares legales y éticos en la investigación y experimentación.