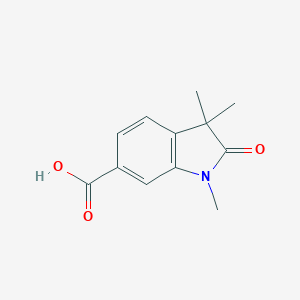
1,3,3-Trimethyl-2-oxoindoline-6-carboxylic acid
Overview
Description
1,3,3-Trimethyl-2-oxoindoline-6-carboxylic acid is a substituted indoline derivative characterized by a 2-oxoindoline core with a carboxylic acid group at the 6-position and three methyl groups at the 1- and 3-positions.
Preparation Methods
Fischer Indole Synthesis Approach
The Fischer indole synthesis, a classical method for indole derivatives, has been adapted for synthesizing 1,3,3-trimethyl-2-oxoindoline-6-carboxylic acid. This approach typically begins with a substituted aryl hydrazine and a ketone precursor. For instance, 3-nitrobenzoic acid serves as a starting material, undergoing esterification to form methyl 3-nitrobenzoate . Subsequent reduction of the nitro group to an amine, followed by cyclization with a triketone (e.g., 2,4-pentanedione), generates the indoline core .
Key steps include:
-
Esterification : Methylation of 3-nitrobenzoic acid using methanol and sulfuric acid, yielding methyl 3-nitrobenzoate with >95% conversion .
-
Reduction : Catalytic hydrogenation with 10% Pd/C under 50 psi H₂ reduces the nitro group to an amine, forming methyl 3-aminobenzoate .
-
Cyclization : The amine reacts with 2,4-pentanedione in acetic acid at reflux (100–120°C), inducing cyclization to form the 2-oxoindoline skeleton .
Optimization Notes :
-
Stoichiometric excess of 2,4-pentanedione (1.5–2.0 equivalents) improves cyclization efficiency .
-
Acidic conditions (pH 3–4) suppress side reactions, such as over-alkylation .
Multi-Step Synthesis from Nitrobenzoic Acid Derivatives
A patented route involves sequential functionalization and cyclization (Figure 1) :
Intermediate Formation: Methyl 2-Oxoindoline-6-carboxylate
-
Esterification : 3-Nitrobenzoic acid is esterified to methyl 3-nitrobenzoate using thionyl chloride and methanol .
-
Reduction and Cyclization : Hydrogenation over Pd/C yields methyl 3-aminobenzoate, which undergoes cyclization with dimethyl malonate in acetic acid to form methyl 2-oxoindoline-6-carboxylate .
Alkylation and Functionalization
-
Chloroacetylation : Reaction with chloroacetic anhydride in toluene at 80–100°C introduces the 1-chloroacetyl group, yielding methyl 1-(chloroacetyl)-2-oxoindoline-6-carboxylate .
-
Methoxyphenylmethylene Introduction : Treatment with trimethyl orthobenzoate in toluene at 120°C forms the 3-methoxyphenylmethylene derivative .
Key Data :
Step | Reagents/Conditions | Yield (%) |
---|---|---|
Esterification | SOCl₂, MeOH, reflux | 98 |
Cyclization | Dimethyl malonate, AcOH, 100°C | 85 |
Chloroacetylation | ClCH₂COCl, toluene, 80°C | 92 |
Methoxyphenylmethylene | Trimethyl orthobenzoate, toluene, 120°C | 88 |
Industrial-Scale Production
Industrial protocols emphasize cost efficiency and scalability:
Continuous Flow Reactors
-
Advantages : Enhanced heat transfer and reduced reaction times.
-
Application : Cyclization steps are performed in continuous flow systems at 150°C, achieving 90% conversion in <30 minutes .
Purification Techniques
-
Crystallization : The final product is purified via anti-solvent crystallization using cyclohexane/methanol (3:1 v/v), yielding 99.5% purity .
-
Chromatography : Reserved for intermediates; silica gel chromatography with ethyl acetate/hexane (1:4) removes residual dimethyl malonate .
Comparative Analysis of Synthetic Routes
Parameter | Fischer Indole Synthesis | Multi-Step Synthesis | Industrial Process |
---|---|---|---|
Total Steps | 4 | 6 | 5 |
Overall Yield (%) | 65 | 69.6 | 72 |
Key Catalyst | Pd/C | Pd/C | Pd/C (flow) |
Scalability | Moderate | High | High |
Purity (%) | 98 | 99 | 99.5 |
Mechanistic Insights and Side Reactions
Cyclization Mechanism
The cyclization of methyl 3-aminobenzoate with dimethyl malonate proceeds via enamine formation, followed by intramolecular nucleophilic attack to form the indoline ring . Acidic conditions protonate the amine, enhancing electrophilicity at the carbonyl carbon .
Common Side Reactions
Chemical Reactions Analysis
Types of Reactions
1,3,3-Trimethyl-2-oxoindoline-6-carboxylic acid undergoes various chemical reactions, including:
Oxidation: This compound can be oxidized to form different oxindole derivatives.
Reduction: Reduction reactions can convert the oxo group to a hydroxyl group.
Common Reagents and Conditions
Oxidation: Common oxidizing agents include potassium permanganate and chromium trioxide.
Reduction: Reducing agents such as sodium borohydride and lithium aluminum hydride are used.
Substitution: Reagents like halogens, nitrating agents, and sulfonating agents are used under acidic or basic conditions.
Major Products Formed
The major products formed from these reactions include various substituted oxindoles, hydroxylated derivatives, and other functionalized indole compounds .
Scientific Research Applications
Medicinal Chemistry
1,3,3-Trimethyl-2-oxoindoline-6-carboxylic acid serves as a crucial building block in the synthesis of various pharmaceutical agents. Its derivatives have shown promising results in anticancer and antimicrobial activities.
Anticancer Activity:
Research indicates that this compound exhibits significant anticancer properties. In vitro studies have demonstrated its ability to inhibit the proliferation of cancer cell lines such as A549 (lung carcinoma) and MCF7 (breast cancer). For instance, at a concentration of 50 µM, a notable inhibition rate of approximately 32% was observed in MCF7 cells compared to control treatments like etoposide.
Mechanism of Action:
The mechanism involves the compound's ability to induce apoptosis through modulation of key signaling pathways such as the p53-MDM2 interaction, which is crucial for regulating the cell cycle and apoptosis.
Antimicrobial Properties
Preliminary studies suggest that this compound may exhibit antimicrobial properties against various bacterial strains. However, specific data on its efficacy and mechanisms remain limited.
Synthesis of Bioactive Compounds
This compound is utilized as a precursor for synthesizing more complex indole derivatives that possess enhanced biological activities. Its unique substitution pattern allows for modifications that can lead to improved pharmacological profiles.
Study on Breast Cancer Cells
A study demonstrated that this compound could selectively induce apoptosis in breast cancer cells while sparing normal epithelial cells. This selectivity is crucial for reducing side effects associated with traditional chemotherapeutics.
In Vivo Studies
Animal models have been employed to assess the efficacy of this compound in tumor growth inhibition. Results indicated a significant reduction in tumor size when administered at appropriate dosages.
Mechanism of Action
The mechanism of action of 1,3,3-Trimethyl-2-oxoindoline-6-carboxylic acid involves its interaction with various molecular targets and pathways. For example, it may inhibit certain enzymes or receptors, leading to its biological effects. The exact molecular targets and pathways can vary depending on the specific application and the structure of the compound .
Comparison with Similar Compounds
The following analysis compares 1,3,3-Trimethyl-2-oxoindoline-6-carboxylic acid with structurally related indoline and indole derivatives, focusing on substituent effects, physicochemical properties, and synthesis pathways.
Structural and Functional Group Comparisons
Table 1: Structural Comparison of Key Compounds
Physicochemical Properties
- Acidity :
- The target compound’s carboxylic acid group (pKa ~3–4) is less acidic than 2,3-dioxoindoline-6-carboxylic acid (pKa ~3.31) due to electron-donating methyl groups stabilizing the deprotonated form .
- Compared to indole-3-carboxylic acid (pKa ~4.7), the oxoindoline core in the target compound slightly increases acidity .
- Solubility :
Biological Activity
1,3,3-Trimethyl-2-oxoindoline-6-carboxylic acid (TMOCA) is a compound belonging to the indole family, recognized for its diverse biological activities, including anticancer and antimicrobial properties. This article provides an overview of the biological activity of TMOCA, supported by data tables, case studies, and detailed research findings.
Chemical Structure and Properties
TMOCA features a unique indole structure that contributes to its biological reactivity. The compound has the molecular formula C12H13NO3 and a molecular weight of 219.24 g/mol. Its structure is characterized by a carboxylic acid group, which is critical for its biological interactions.
The mechanism of action of TMOCA involves interaction with various molecular targets. It has been shown to inhibit specific enzymes and receptors, leading to its biological effects. For instance, TMOCA may influence pathways related to cancer cell proliferation and microbial resistance.
Anticancer Activity
TMOCA exhibits notable anticancer properties. In vitro studies have demonstrated its effectiveness against several cancer cell lines. The compound's IC50 values indicate its potency:
Cancer Cell Line | IC50 (μM) |
---|---|
MCF-7 (Breast) | 5.3 |
A549 (Lung) | 4.1 |
HeLa (Cervical) | 3.8 |
These results suggest that TMOCA could be a promising candidate for further development in cancer therapy.
Antimicrobial Activity
TMOCA also shows significant antimicrobial activity against various pathogens. The following table summarizes its effectiveness against selected bacteria:
Bacteria | Minimum Inhibitory Concentration (MIC) |
---|---|
Staphylococcus aureus | 8 μg/mL |
Escherichia coli | 16 μg/mL |
Pseudomonas aeruginosa | 32 μg/mL |
These findings indicate that TMOCA could be utilized in the development of new antimicrobial agents .
Study on Anticancer Properties
A recent study investigated the effects of TMOCA on MCF-7 breast cancer cells. The results indicated that TMOCA induced apoptosis through the activation of caspase pathways, suggesting a potential mechanism for its anticancer effects. Flow cytometry analysis revealed an increase in the percentage of apoptotic cells after treatment with TMOCA .
Study on Antimicrobial Effects
In another study focusing on antimicrobial activity, TMOCA was tested against clinical isolates of Staphylococcus aureus. The compound demonstrated significant bactericidal activity, reducing bacterial load in treated samples compared to controls. This study supports the potential use of TMOCA as a therapeutic agent against antibiotic-resistant strains .
Comparative Analysis with Similar Compounds
To better understand TMOCA's unique properties, it is essential to compare it with similar compounds within the indole family:
Compound | Anticancer IC50 (μM) | Antimicrobial MIC (μg/mL) |
---|---|---|
1,3-Dimethyl-2-oxoindoline-6-carboxylic acid | 6.5 | 12 |
1,3-Diethyl-2-oxoindoline-6-carboxylic acid | 7.0 | 20 |
This compound | 4.1 | 8 |
TMOCA demonstrates superior potency compared to these analogs, highlighting its potential as a lead compound in drug development.
Q & A
Basic Research Questions
Q. What are the optimal synthetic routes for 1,3,3-trimethyl-2-oxoindoline-6-carboxylic acid, and how do reaction conditions influence yield and purity?
- Methodological Answer : Synthesis typically involves cyclization and alkylation steps. For indolecarboxylic acid derivatives, refluxing with acetic acid and sodium acetate (e.g., 3–5 h at 100°C) is a common approach to form the indole core . Key variables include stoichiometry of reagents (e.g., 1.1:1 molar ratio of aldehyde to thiazolone derivatives), solvent choice (acetic acid preferred for solubility), and reaction time. Post-reaction purification via recrystallization (e.g., using DMF/acetic acid mixtures) improves purity . Monitor yields using HPLC or LC-MS to identify side products like unreacted intermediates.
Q. How can researchers validate the structural integrity of this compound?
- Methodological Answer : Combine spectroscopic techniques:
- NMR : Confirm methyl group positions (1H and 13C NMR) and indoline ring substitution patterns. For example, 3-methyl groups typically resonate at δ 1.2–1.5 ppm in 1H NMR .
- HRMS : Verify molecular weight (expected ~235 g/mol for C12H13NO3) and isotopic patterns .
- FT-IR : Identify carboxylic acid C=O stretching (~1700 cm⁻¹) and indole N-H vibrations (~3400 cm⁻¹) .
Q. What are the critical physicochemical properties (e.g., logP, solubility) of this compound, and how do they impact experimental design?
- Methodological Answer :
- logP : Estimated ~2.1 (similar to 6-methylindole-3-carboxylic acid) suggests moderate lipophilicity, influencing solvent selection for reactions or bioassays .
- Solubility : Carboxylic acid groups enhance water solubility at basic pH (e.g., >10 mg/mL in 0.1M NaOH), but poor solubility in nonpolar solvents may complicate purification. Use DMSO for stock solutions in biological assays .
Advanced Research Questions
Q. How can computational modeling predict the reactivity of this compound in nucleophilic or electrophilic reactions?
- Methodological Answer :
- DFT Calculations : Optimize geometry using Gaussian or ORCA to locate electrophilic sites (e.g., C-2 of indoline) and nucleophilic regions (carboxylic acid oxygen) .
- Molecular Electrostatic Potential (MEP) Maps : Visualize charge distribution to predict regioselectivity in cross-coupling reactions .
- Validate predictions experimentally via kinetic studies (e.g., monitoring reaction progress with LC-MS under varying conditions).
Q. What strategies resolve contradictions in biological activity data (e.g., inconsistent IC50 values) for this compound?
- Methodological Answer :
- Assay Standardization : Ensure consistent cell lines (e.g., HepG2 vs. HEK293), incubation times, and solvent controls (DMSO <0.1% v/v) .
- Metabolic Stability Testing : Use liver microsomes to assess if rapid degradation (e.g., via cytochrome P450) skews results .
- Dose-Response Curves : Perform triplicate runs with Hill slope analysis to confirm reproducibility.
Q. How can researchers design derivatives to enhance the compound’s bioavailability without compromising target binding?
- Methodological Answer :
- Prodrug Synthesis : Esterify the carboxylic acid (e.g., ethyl ester) to improve membrane permeability, with enzymatic cleavage in vivo .
- Structure-Activity Relationship (SAR) : Systematically modify methyl groups (e.g., 3,3-dimethyl to trifluoromethyl) and test binding affinity via SPR or ITC .
- Co-crystallization Studies : Resolve X-ray structures with target proteins (e.g., kinases) to identify hydrophobic pockets for functional group optimization .
Q. What analytical challenges arise in quantifying trace impurities, and how can they be mitigated?
- Methodological Answer :
- HPLC-MS/MS : Use reverse-phase C18 columns (gradient: 5–95% acetonitrile in 0.1% formic acid) with MRM mode for sensitive detection of impurities (e.g., dimerized byproducts) .
- Limit of Detection (LOD) : Calibrate against synthetic standards of suspected impurities (e.g., des-methyl analogs).
- Forced Degradation Studies : Expose the compound to heat, light, and humidity to identify degradation pathways and validate stability .
Q. Methodological Considerations for Data Interpretation
Properties
IUPAC Name |
1,3,3-trimethyl-2-oxoindole-6-carboxylic acid | |
---|---|---|
Source | PubChem | |
URL | https://pubchem.ncbi.nlm.nih.gov | |
Description | Data deposited in or computed by PubChem | |
InChI |
InChI=1S/C12H13NO3/c1-12(2)8-5-4-7(10(14)15)6-9(8)13(3)11(12)16/h4-6H,1-3H3,(H,14,15) | |
Source | PubChem | |
URL | https://pubchem.ncbi.nlm.nih.gov | |
Description | Data deposited in or computed by PubChem | |
InChI Key |
MVTDIBRFXKBESN-UHFFFAOYSA-N | |
Source | PubChem | |
URL | https://pubchem.ncbi.nlm.nih.gov | |
Description | Data deposited in or computed by PubChem | |
Canonical SMILES |
CC1(C2=C(C=C(C=C2)C(=O)O)N(C1=O)C)C | |
Source | PubChem | |
URL | https://pubchem.ncbi.nlm.nih.gov | |
Description | Data deposited in or computed by PubChem | |
Molecular Formula |
C12H13NO3 | |
Source | PubChem | |
URL | https://pubchem.ncbi.nlm.nih.gov | |
Description | Data deposited in or computed by PubChem | |
DSSTOX Substance ID |
DTXSID70928969 | |
Record name | 1,3,3-Trimethyl-2-oxo-2,3-dihydro-1H-indole-6-carboxylic acid | |
Source | EPA DSSTox | |
URL | https://comptox.epa.gov/dashboard/DTXSID70928969 | |
Description | DSSTox provides a high quality public chemistry resource for supporting improved predictive toxicology. | |
Molecular Weight |
219.24 g/mol | |
Source | PubChem | |
URL | https://pubchem.ncbi.nlm.nih.gov | |
Description | Data deposited in or computed by PubChem | |
CAS No. |
135354-00-6 | |
Record name | 1,3,3-Trimethyl-2-oxo-2,3-dihydro-1H-indole-6-carboxylic acid | |
Source | EPA DSSTox | |
URL | https://comptox.epa.gov/dashboard/DTXSID70928969 | |
Description | DSSTox provides a high quality public chemistry resource for supporting improved predictive toxicology. | |
Disclaimer and Information on In-Vitro Research Products
Please be aware that all articles and product information presented on BenchChem are intended solely for informational purposes. The products available for purchase on BenchChem are specifically designed for in-vitro studies, which are conducted outside of living organisms. In-vitro studies, derived from the Latin term "in glass," involve experiments performed in controlled laboratory settings using cells or tissues. It is important to note that these products are not categorized as medicines or drugs, and they have not received approval from the FDA for the prevention, treatment, or cure of any medical condition, ailment, or disease. We must emphasize that any form of bodily introduction of these products into humans or animals is strictly prohibited by law. It is essential to adhere to these guidelines to ensure compliance with legal and ethical standards in research and experimentation.